Targeting breast cancer resistance protein (BCRP/ABCG2) in cancer
Introduction
As statistically estimated, there are approximately 2.3 million women diagnosed with breast cancer worldwide, with more than 665,684 patients dying from breast cancer in 2022 (1), showing that it has become the severest threat for the women. Breast cancer is sensitive to chemotherapeutic drugs. Chemotherapeutics can improve the poor prognosis and relapse, and also present alleviated effect on patients with advanced breast cancer. However, in the long-term clinical practice, the multidrug resistance (MDR) that occurs during the treatment of breast cancer by chemotherapy drugs has gradually caused concern (2). The resistance of cancer cells to drug was first discovered in 1970 (3,4). MDR decreases the sensitivity of breast cancer cells to chemotherapeutic drugs, hence the inhibitory effect of drugs on breast cancer cells is weakened or even lost. Therefore, the research on MDR of breast cancer has attracted more and more attention from scholars in the field of cancer therapy (5). Recently, it was shown that MDR is closely related to the ATP-binding cassette (ABC) transporter superfamily, which is one of the biggest active transport molecule families (5). ABC transporter is widespread in both eukaryotic and prokaryotic, and it has been found in all organisms (6,7). The ABC transporter superfamily refers to a class of membrane mosaic proteins that are capable of transporting a variety of substrates (8), including cholesterol, polypeptides, drugs, toxins, bile salts, organic anions, nucleosides, sterols, etc. (9,10). Normally the ABC family protects cells from xenobiotics by extruding them out of the cells (11,12). So far, 48 human ABC transporters have been discovered, which are divided into seven subfamilies (ABC-A to ABC-G) (7). Three of them are closely related to MDR, namely P-glycoprotein (P-gp/ABCB1), the first discovered ABC transporter (13), multidrug resistance-associated protein-1 (MRP1/ABCC1) and breast cancer resistance protein (BCRP/ABCG2) (14,15). And earlier studies showed that the process of cancer drug transport consists of at least 12 ABC transporters from four ABC subfamilies (16,17). Therefore, ABC transporters have gradually been recognized as one of the most important targets for the treatment of breast cancer MDR (17). At the same time, BCRP gradually shows its specificity and importance that differ from P-gp and MRP1 in the process of the emergence of the MDR in breast cancer (13,17). This article focuses on the importance and possible molecular mechanisms of BCRP in the development of MDR in breast cancer and the progress of its inhibitors, and explores the possible research directions and anti-tumor strategies of BCRP as a potential target for reversing MDR in breast cancer.
The structure of BCRP
BCRP is a member of the ATP-binding transporter superfamily G encoded by the ABCG2 gene located in chromosome 4q22 (18,19). It has been considered as the most necessary factor of drug resistance in MCF-7/MX cells (20). Human ABCG2 is a glycosylated plasma-membrane protein, and the molecular weight is about 75 kDa. BCRP normally can be found in the placenta, intestines, colon, liver, hematopoietic stem cells, ducts and lobules of the breast (21). Similar to P-gp and MRP1, BCRP has a certain homologous sequence on the structure and work as a “drug pump” (22). It is capable of pumping out the xenobiotics, organic conjugates of drugs, endogenous substance through the way depending on efflux which activated by ATP (3,20). It has wide range of substrates, including hydrophobic chemotherapeutics, hydrophilic organic anions conjugated, sulfate, glutathione and glucuronate, as the sulfated conjugated fit the BCRP better (23). Besides, it can act as transport way for nucleoside drug (24). Compared with the transporter P-gp and MRP1, which have two nucleotide-binding domains (NBD) and two transmembrane domains (TMD), BCRP consists of only one NBD at N-terminal and one TMD at C-terminal (Figure 1) (22). Thus, BCRP is called half-transporter. The half-transporters commonly locate in the cell, but BCRP is an exception as it is the first half-transporter locating on the cell membrane (25,26). BCRP is likely to form homodimers, tetramers, dodecamers and even larger multimeric structures by intramolecular disulfide linkages (27), while the formation of efflux channel cavity can significantly improve its external pump efficiency (17). Among all polymers, only the homodimers are really active (17). The energy-dependent efflux pump inside BCRP is a special among the ABC family (28). The high-resolution crystal 3D structure of BCRP has been published (22,29,30), considerably promoting the design and development of BCRP inhibitors (31). Although it is discussed that there is no connection between the function of BCRP and poor clinical results (32), the correlation between BCRP and MDR has still remained to be elucidated.
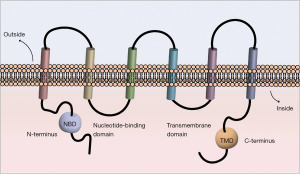
The mechanisms of MDR in breast cancer
The causes of MDR in breast cancer vary, and the occurrence mechanisms are widely investigated. By far, the combined effects are considered as the main reason for the occurrence and the development of MDR in breast cancer. Although the specific mechanism has not yet been fully explained, the following three types are currently concerned.
Abnormal activation of membrane transporter function
The transporters are proteins on the membrane having function of drug output pump, such as P-gp, MRP1, BCRP, lung resistance protein (LRP) and the like. These proteins are usually in an abnormal activation state in tumor tissue, which can pump chemotherapeutic drugs out of the cell to reduce intracellular drug accumulation and cause drug resistance (33). P-gp, MRP1 and BCRP belong to ATP-binding transporters, but the MDR mediated by them is inconsistent, and they have different substrate spectrums, although they share several kinds of inhibitors, like elacridar (GF120918) (34), cyclosporine A (35), biricodar (VX-710) (36) and tariquidar (XR-9576) (37). At present, in clinical patients with malignant tumors, the combination of chemotherapeutic drugs and transporter reversal agents is considered better to restore the sensitivity of drug-resistant cells to drugs (38,39).
Cell apoptosis escapes
Cell apoptosis escape (cell resistance to apoptosis) is also one of the causes of MDR in breast cancer. Under normal circumstances, apoptosis is cell death caused by gene control, so once the apoptotic pathway is inhibited, it is very likely that MDR will be induced (40). The study found that P-gp is one of the bridges between MDR and apoptosis. P-gp can inhibit the activation of caspase-3 and reduce the sensitivity of cell L1210 to cisplatin. P-gp can reduce the sensitivity of cell L1210 to cisplatin followed with inactivation of caspase-3. P-gp also can prevent apoptosis induced by the Fas death receptor pathway independent of its drug efflux activity (41). On the other hand, abnormalities of apoptosis-related factors such as p53, Bcl-2 family, and survivin can mediate the development of MDR breast cancer (42).
Molecular markers of drug resistance
For the past few years, new markers of drug resistance of breast cancer have spread out, providing new targets and directions for reversing breast cancer MDR (17). In the breast cancer mitoxantrone tolerant cell line MCF-7/MX, abnormal expression of some genes or proteins can regulate the occurrence of MDR induced by related signaling pathways (43). Studies have shown that the expression of cytokeratin 8 (CK8), CUE domain containing 2 (CUEDC2), nucleophosmin (NPM1) is several times higher than the human breast cancer cell MCF-7 (44,45). CUEDC2 can use ubiquitin proteasome to degrade estrogen receptor (ER), resulting in the endure of breast cancer patients to endocrinotherapy (45). CUEDC2 has the possibility to become a new target of therapy, which aims at dealing with tamoxifen resistance in the field of breast cancer (45,46). High expression of NPM1 can activate phosphatidylinositol-3-kinases (PI3K)/Akt signaling pathway and inhibit downstream apoptotic factors (47). When the expression of NPM1 was interfered, the drug resistance of MCF-7/MX cells was evidently reduced (48). Annexin a2 was also reported as a key marker molecule in breast cancer drug resistance and metastasis, and after Annexin a2 gene silencing, the proliferation rate of MCF-7/adriamycin (ADM) was decreased, both rate and the speed of tumor formation were significantly decreased (49). In recent years, more and more breast cancer drug resistance molecular markers have been discovered, providing original new drug targets and new ideas for clinical treatment of breast cancer resistance (15).
BCRP-mediated MDR in breast cancer
BCRP promoter triggers transcription and activates drug resistance
The promoter region of the BCRP gene has no TATA box, but contains a CAAT box, an AP1/2 site, and multiple Sp-1 sites downstream of the CpG island (22). Many biological factors can bind to specific elements of the enhancer or promoter region of the BCRP gene, such as nuclear factor kappa-B (NF-κB) and progesterone receptor (PR), ER, etc., mediate the development of breast cancer MDR by enhancing the transcriptional regulation of BCRP (Figure 2) (50). It was reported that the overexpression of human epidermal growth factor receptor 2 (HER2) will up-regulate BCRP via NF-κB and PI3K/Akt (28). In addition, BCRP expression is also regulated by a number of nuclear transcription factors, including the peroxisome proliferator-activated receptor-γ (PPARγ) and hypoxia inducible factor-1 (HIF-1) (50).
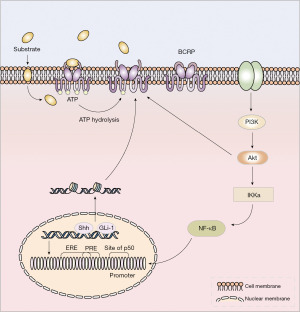
NF-κB
NF-κB is an important nuclear transcription factor composed of a series of proteins in the form of homologous or heterodimeric forms including p50, p52, p65 (RelA) and RelB in the Rel family. The morphology of p65/p50 heterodimers is most common in these dimeric forms (51). NF-κB (p50) is present in the promoter region of BCRP, so NF-κB can activate BCRP transcriptional expression by binding to this site (52). In MCF-7 cells overexpressing HER2, BCRP is overexpressed, the sensitivity of these cells to many chemotherapeutic drugs such as paclitaxel, cisplatin, mitoxantrone, and 5-fluorouracil decreased significantly (28). HER2 up-regulates BCRP expression by activating the PI3K/Akt/NF-κB signaling pathway (28). The effect of drug duration can be reversed by pretreating HER2 with NF-κB inhibitor Bay11-7082, PI3K inhibitor LY294002 or transfecting with the dominant-negative IkBa mutation expression plasmid (28). To a certain extent, it can restore cells sensitivity to drugs and reduce the occurrence of drug resistance.
ER/PR
Breast cancer is a hormone-dependent tumor, and the activation of related hormone receptors is closely related to the occurrence of breast cancer resistance (53). The BCRP gene promoter sequence (-243/-115) has an estrogen response element (ERE), and the progesterone response element (PRE) is located at BCRP promoter -187 to -173 segment (54). Due to the overlap of PRE and ERE, all of estradiol, estriol and estrone can bind to them and activate transcription of BCRP (54). Zhang et al. constructed a BCRP-overexpressing breast cancer resistant cell line and found that toremifene mediated by ERα can bind to the ERE of the upstream regulatory sequence of the BCRP promoter, negatively regulate the expression of BCRP and inhibit BCRP’s function of transporting drug, which effectively reverses BCRP-mediated MDR in vitro (55). Pradhan et al. also found that there is an interaction between ER and NF-κB (56). They co-regulate BCRP transcription, thus estrogen and inflammatory factors can execute their respective roles by regulating the binding of related transcription factors to adjacent response elements, and activate the activity (56). Furthermore, the research indicates that estrogen treatment increases the level of BCRP (54). The discovery of this transcriptional mechanism further indicates that both inflammation and estrogen can promote cancer progression and are of great significance for the treatment of late-period breast cancer (57).
PR isoforms PRA and PRB regulate BCRP differentially in human placental choriocarcinoma cells. Progesterone markedly increases BCRP expression and activity in PRB-transfected cells but not PRA (58). However, another report showed that progesterone acts via PRA and PRB to regulate BCRP expression in a placental cell line (59).
PPAR
Valproic acid elevates BCRP transcriptional activity through enhancing the binding of PPARα to its response element in the BCRP promoter (60). PPARγ can increase BCRP expression in the placenta, which is beneficial to understand the mechanisms that protect the fetus from xenobiotic exposure during development (61).
Pregnane X receptor (PXR)
The PXR has been reported to be involved in regulating the expression of majority of transporters (62). The expression of PXR in renal tubular epithelium cells was significantly increased by doxorubicin (DOX) at a specific concentration causing cellular damage. The organic cation transporter 1 (OCT1) and BCRP express significantly lower in the DOX-treated cells than in the untreated cells (63).
HIF
It was found that under low oxygen conditions, HIF-1α/ARNT heterodimer binding to a hypoxia response element (HRE) activates BCRP transcription (64). HIF-2α directly increases BCRP expression and promotes the resistance of ovarian cancer stem cells (OCSCs) to ADR. HIF-2α overexpression endows OCSCs with resistance to ADR by activating BCRP expression and ADR transportation (65). Additionally, BCRP was controlled by a reactive oxygen species (ROS)-dependent cross-talk process between DOX and hypoxia, inducing drug resistance in HT-29 WT and HT-29DxR cells (66). Thus, HIF-1α or HIF-2α/BCRP axis was regarded as a therapeutic target for treating drug-resistant cancer (65-67).
Aryl hydrocarbon receptor (AHR)
AHR is a transcriptional activator of the human BCRP. AHR plays an unprecedented role in cellular adaptive response and cytoprotection by up-regulating BCRP (68). Activating AHR in human placental trophoblasts by buprenorphine, norbuprenorphine, R-methadone, and S-methadone leads to upregulation of BCRP expression. Overexpression of AhR further increased BCRP mRNA transcription and protein expression. Silence of AhR by shRNA decreased BCRP expression, which was reversed by recovering AhR expression (69).
BCRP is involved in the activation of tumor resistance signaling pathway
In BCRP-expressing cancer cells or tissues, BCRP can not only activate multiple signaling pathways or factors to mediate drug resistance, but also act as one of the activation signals, triggering other drug-resistant signaling pathways to mediate the occurrence of MDR in breast cancer (70,71).
PI3K/Akt signaling pathway
PI3K/Akt signaling pathway plays a very important role in regulating the physiological activities of normal cells (72). However, in a variety of cancer cells, excessive activation of PI3K/Akt can significantly enhance the proliferation, invasion and drug resistance of cancer cells (73). The latest research results showed that ABC transporter family, especially BCRP, plays a key role in PI3K/Akt-mediated breast cancer resistance (74). The PI3K/Akt pathway is significantly activated in DOX-tolerant A2780 cells, whereas BCRP expression is inhibited and cell sensitivity to DOX is up-regulated following inhibition of PI3K/Akt phosphorylation activation (75). In addition, after using PI3K/Akt inhibitor LY294002 or BCRP siRNA against MCF-7 cells, the cell cycle was arrested in G0/G1 phase (75). However, LY294002 or BCRP siRNA were used with mitoxantrone as a combination, the accumulation of MCF-7 cells blocked in G2/M phase is significantly increased (75). It can overcome the occurrence of MDR in breast cancer to some extent, and provide new ideas for clinical treatment (75,76). Komeili-Movahhed et al. reported that the activation of Akt can promote the enrichment of BCRP on the membrane surface and BCRP usually existed as functional homodimer (77) Thereby, the stimulation of Akt increased the efficiency of BCRP pump drugs and mediated resistance. Therefore, the relative expression of BCRP on the cell surface is regulated by the PI3K/Akt signaling pathway, in particular, changes in Akt activity can change the conformation of BCRP to localize it to the cell membrane, thereby affecting the extrusion of BCRP on substrates and promoting efflux (78) (Figure 3).
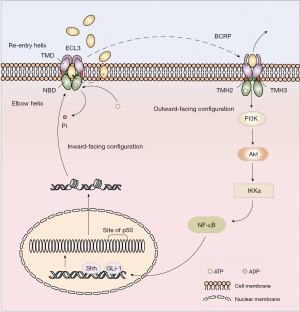
Therefore, BCRP expression and functional changes are closely related to the activation of PI3K/Akt signaling pathway. While BCRP itself mediates drug resistance, interaction with PI3K/Akt causes other drug resistance pathways to be activated to varying degrees. Such as anti-apoptosis, altered enzyme activity, immune escape, etc., together to mediate the occurrence of breast cancer MDR (76).
Mitogen-activated protein kinases (MAPKs) signaling pathway
The MAPKs control various cellular processes by receiving diverse extracellular signals to intracellular responses (79). Activation of mitogen-activated protein kinase cascade increased expression of BCRP, in which was activated by epidermal growth factor (EGF) (80). Activation of MAPK/ERK pathway up-regulates P-gp expression but down-regulated BCRP expression, while activation of JNK pathway up-regulated BCRP expression (81). BCRP-specific inhibitor, fumitremorgin C (FTC), and MAPK pathway inhibitor, U0126, could induce cell apoptosis by degrading endogenous BCRP in Hep-2T cells. Furthermore, inhibition of MAPK pathway by U0126 promoted anti-tumor effects of MX in vivo (82). BCRP expression increased significantly by c-Jun N-terminal kinase (JNK) inhibitor SP600125 exposure in UL-1 and Ema cells, but was unaffected by U0126 exposure in CLBL-1 cells, in which activation of extracellular regulating kinase (ERK) pathway was observed in non-treated cells. These results suggested that MAPK/ERK and JNK pathways downregulated BCRP expression (83).
Hedgehog (Hh) signaling pathway
Abnormal activation of Hh signaling pathway is closely related to the occurrence and development of many carcinomas (84,85). In recent years, it was found that Hh signaling pathway is also involved in the process of tumor resistance, and mainly through the regulation of membrane transporter expression and activity to reduce the sensitivity of tumor cells to chemotherapeutic drugs (86).
In the study of human breast cancer paclitaxel-resistant cells MCF-7/PTX, it was found that Shh and Gli-1 were highly expressed in the drug-resistant cells, and Shh and Gli-1 were the target genes of Hh pathway, which was considered to be the representative marker activated by this pathway (87). So chemotherapy drugs may mediate breast cancer resistance by up-regulating Hh signaling pathway-related proteins and genes, and targeted therapy for this signaling pathway will be a new direction to overcome breast cancer resistance (88). In tamoxifen-resistant breast cancer, tamoxifen resistance is closely linked to the activation of PI3K/Akt and Hh signaling pathways. Targeting Hh signaling pathway alone or in combination with PI3K/Akt pathways may be new options for the treatment of tamoxifen-resistant breast cancer (89). Knockdown of Hh pathway components smoothened (SMO) or GLI1 (glioma-associated oncogene homolog) inhibits proliferation of tamoxifen resistant cells (89). Although there is no direct report on the direct relationship between BCRP and Hh signaling pathway in breast cancer resistance, we can speculate that there must be some interaction between them, being worthy of further investigation.
BCRP inhibitors
As BCRP plays a critical role in the occurrence of MDR, the substrates of it include many chemotherapeutic drugs, e.g., flavopiridol, the camptothecins, 9-aminocamptothecin, topotecan, irinotecan and its active metabolite SN-38, the indolocarbazoles J-107088, NB-506, compound A and UCN-01, methotrexate, porphyrins (23). And the mutation inside gene will render new type of drug resistance (90,91) and change the drug efflux activity (91,92). It is observed that the variation of amino acid will affect the function of BCRP and the drug elimination. The mutation of amino acid has differences between ethnicities (93-95).
The inhibition on BCRP becomes one of the promising directions to against MDR. Studies have found that many tyrosine kinase inhibitors such as gefitinib, imatinib, erlotinib, nilotinib, can decrease the dynamic of BCRP-ATPase, and inhibit BCRP to some degree (23,71). Furthermore, some protease inhibitors, antifungal azoles and immunosuppressants were also proved to be BCRP inhibitors. And these compounds belong to non-specific BCRP inhibitors because they have been proved to be inhibitors for other specific proteins (23). There are many specific BCRP inhibitors that shows the high affinity with the BCRP and prospectively becomes a new drug for cancer therapy. Since the first discovery of BCRP, the list of substrates and inhibitors keep extending (23,71,96).
Non-specific inhibitors
At present, a various of non-specific inhibitors of BCRP has been found that they can restrain P-gp and BCRP simultaneously, or they can inhibit BCRP while having potent activity on the other targets (23). For example, GF120918 developed as a second-generation inhibitor of P-gp was found to inhibit BCRP with IC50 of about 50 nmol/L (34). The human immunodeficiency virus protease inhibitor, such as ritonavir, saquinavir could also show great inhibitory effect on BCRP. In addition, some small-molecule tyrosine kinase inhibitors, including imatinib, nilotinib, lapatinib, are not only the substrates of BCRP but also the inhibitors of BCRP, which can block the binding cavity 1 of the BCRP protein and prevent drug efflux (20). Therefore, small-molecule inhibitors cooperated with traditional chemotherapy can reverse the MDR and enhance therapeutic effect. Aspergillus fumigate C (FTC) was the first discovery of inhibitor that could effectively inhibit BCRP function (97).
Some natural compounds also exhibit inhibition on BCRP. Curcumin, a chemical isolated from the Curcuma longa L., has been proved in vitro to inhibit MDR mediated by ABCG2 (98). And its major metabolite, tetrahydrocurcumin, also can modulate the function of ABCB1, ABCG2, and ABCC1, extending the MDR reversing activity of curcuminoids in vivo (99). Additionally, curcumin can enhance the tumoricidal effect of mitomycin C by inhibiting ABCG2 expression in stem cell-like breast cancer cells (100). All is shown in Table 1.
Table 1
Drug | Pharmacological action | Clinical application | Reference |
---|---|---|---|
Gefitinib, erlotinib, lapatinib | EGFR tyrosine kinase inhibitor | Non-small cell lung cancer, breast cancer | (23,101,102) |
Imatinib mesylate | PDGFR tyrosine kinase inhibitor | Gastrointestinal stromal tumors, chronic myelogenous leukemia | (103) |
Nilotinib, dasatinib, bosutinib | Inhibits Bcr-Abl tyrosine kinase | Chronic myelogenous leukemia | (104) |
Ritonavir, saquinavir, nelfinavir, lopinavir | Inhibits HIV protease | HIV infections | (105,106) |
GF120918 (elacridar) | Inhibits P-gp | Anti-cancer | (107) |
Boceprevir telaprevir | Inhibits HCV protease | Hepatitis C | (108,109) |
Dipyridamole, nicardipine, nimodipine, nitrendipine | Inhibit calcium channel | Hypertension | (110,111) |
Ketoconazole, itraconazole, fluconazole | Anti-fungal | Treat and prevent superficial and systemic fungal infections | (112) |
Cyclosporin A, tacrolimus, sirolimus | Immunosuppress | Acute leukemia, organ transplant rejection | (113) |
Tariquidar derivative | Inhibits P-gp | Lung cancer, breast cancer | (114) |
Fumitremorgin C and analogues: Ko132, Ko134 and Ko143 | Inhibits ABCG2/BRCP | Breast cancer | (115) |
Eltrombopag | Platelet generator | Increase platelet | (116) |
Curcumin, tetrahydrocurcumin | Suppress NF-κB, inhibits ABC drug transporters, G0/G1 arrest, mitochondria-mediated apoptosis | Human colon carcinomas, lung cancer cells, breast cancer | (117) |
Cryptotanshinone | Anti-cancer, G0/G1 arrest, inhibits ABCG2/BRCP | Cardiovascular diseases | (118) |
BCRP, breast cancer resistance protein; EGFR, epidermal growth factor receptor; PDGFR, platelet-derived growth factor receptor; HIV, human immunodeficiency virus; HCV, hepatitis C virus; P-gp, P-glycoprotein; NF-κB, nuclear factor kappa-B.
Specific inhibitors
In addition to the above-mentioned non-specific inhibitors, specific inhibitors of BCRP were also found. Generally, the specific inhibitors can be separated into six groups, acrylonitriles, anti-human immunodeficiency virus (HIV)-based modulators, flavonoids and chromone derivatives, natural product derivatives and other types of compounds (23,103).
The application of acrylonitriles is relatively narrowed at present, a member of the group named YHO-13177 may play a role in a posttranscriptional downregulation of BCRP (119), but the mode of it is unclear. Zidovudine of anti-HIV-Based modulators was discovered as a substrate of BCRP, show the possibility to be the inhibitor of BCRP (120).
Aspergillus fumigatus toxin from the secretion of Aspergillus fumigatus can specifically inhibit BCRP, but has no effect on P-gp or MRP1. Meanwhile the synthetic analog like Ko134 can inhibit BCRP-mediated transportation, which may be a new direction of specific inhibitor (23). The group of flavonoids shows the potential function in modulation of BCRP. Although their selectivity is lower, it can be compensated by editing their carbon chain. For example, to gain more accuracy, the B-ring of chromone can be replaced to attenuate its toxicity (103).
Other BCRP inhibitors include albamycin, tamoxifen derivative TAG-11 and TAG-139, methylpiperidine derivative biricodar, etc. However, none of those was used in clinical treatment because of its high toxicity and side effects (25). The above-mentioned inhibitors were shown in Table 2.
Table 2
Drug | Pharmacological action | Clinical application | Reference |
---|---|---|---|
Resveratrol | Anti-oxidant, anti-inflammatory, cardioprotective, anti-cancer | (34) | |
Chromone derivative | Anti-inflammation, anti-microbial, anti-cancer, anti-AIDS, anti-oxidant, etc. | Autoimmune disease, cancer, diabetes, atypical psychosis, etc. | (121) |
Tamoxifen TAG-11 and TAG-139 | SERM | Breast cancer | (122,123) |
Novobiocin | Antibacterial, anticancer | Pneumonia, sepsis | (124) |
The pipecolinate derivatives VX-710 (Biricodar) | Inhibits VMAT2 | Cardiovascular diseases, cerebrovascular disorders, heart diseases, hypertension | (125) |
Tryprostatin A | Regulates P-glycoprotein and MRP-1, increases drug uptake and retention and reverses drug resistance mediated by wild-type BCRP | Lung cancer | (36) |
Chrysin | Blocks estrogen, inhibits tumor cell proliferation and metastasis | Anti-cancer, anti-cardiovascular disease, anti-bacterial, anti-inflammatory, etc. | (126) |
Biochanin A | Inhibits FAAH, inhibits EGFR tyrosine kinase, anti-inflammatory, anti-cancer | Anti-cancer, diabetes | (127) |
Pantoprazole | Inhibits H+/K+ ATPase proton pump irreversibly | Duodenal ulcer, gastric ulcer, gastroesophageal reflux | (128) |
SERM, selective estrogen receptor modulator; VMAT2, vesicular monoamine transporter 2; FAAH, fatty acid amide hydrolase; BCRP, breast cancer resistance protein; EGFR, epidermal growth factor receptor; MRP, multidrug resistance-associated protein; AIDS, acquired immune deficiency syndrome.
Conclusions
Breast cancer is the first cancer among women, and the occurrence of drug resistance keeps the mortality breast cancer high. The production of MDR in breast cancer is a multi-factor, multi-channel, multi-stage comprehensive result. As a therapeutic target, the mechanism of action of BCRP is multifaceted. Whether it is the transcription, expression, or function of BCRP itself, or the related resistance signaling pathways involved by BCRP, they may all be key factors mediating the development of cancer MDR. Although there are many reports between membrane transporters and tumor drug resistance, most of the subjects are mainly P-gp and MRP1. Compared with other transport proteins, BCRP has specificity in structural conformation, biochemical characteristics, physiological functions, and other aspects. However, there are a lot of problems about BCRP that need to be elucidated. What is the specific process mechanism for identifying, transporting, and discharging substrates? What are the key factors that substrates bind to BCRP? What are the guiding factors for the final localization of the functional form of BCRP protein on the cell membrane after translation modification? We believe that more in-depth understanding of the mechanism of BCRP in the development of MDR in breast cancer is needed.
In conclusion, the importance of BCRP in MDR in breast cancer is undoubted. It is hoped that the application of biological technology, optimization of model construction, and development of pharmacogenomics can help further elucidate the specific mechanism of BCRP transporter drugs, and provide theoretical basis for clinical treatment of breast cancer, evaluation of chemotherapy efficacy, and analysis of patient prognosis. At the same time, it can also provide new research directions and ideas for further discovery of highly effective and low-toxic BCRP inhibitors and MDR reversal drugs from natural medicine or traditional Chinese medicine.
Acknowledgments
Funding: None.
Footnote
Peer Review File: Available at https://tcr.amegroups.com/article/view/10.21037/tcr-24-1129/prf
Conflicts of Interest: All authors have completed the ICMJE uniform disclosure form (available at https://tcr.amegroups.com/article/view/10.21037/tcr-24-1129/coif). The authors have no conflicts of interest to declare.
Ethical Statement: The authors are accountable for all aspects of the work in ensuring that questions related to the accuracy or integrity of any part of the work are appropriately investigated and resolved.
Open Access Statement: This is an Open Access article distributed in accordance with the Creative Commons Attribution-NonCommercial-NoDerivs 4.0 International License (CC BY-NC-ND 4.0), which permits the non-commercial replication and distribution of the article with the strict proviso that no changes or edits are made and the original work is properly cited (including links to both the formal publication through the relevant DOI and the license). See: https://creativecommons.org/licenses/by-nc-nd/4.0/.
References
- Bray F, Laversanne M, Sung H, et al. Global cancer statistics 2022: GLOBOCAN estimates of incidence and mortality worldwide for 36 cancers in 185 countries. CA Cancer J Clin 2024;74:229-63. [Crossref] [PubMed]
- Kröger N, Achterrath W, Hegewisch-Becker S, et al. Current options in treatment of anthracycline-resistant breast cancer. Cancer Treat Rev 1999;25:279-91. [Crossref] [PubMed]
- Krishnamurthy P, Schuetz JD. Role of ABCG2/BCRP in biology and medicine. Annu Rev Pharmacol Toxicol 2006;46:381-410. [Crossref] [PubMed]
- Juliano RL, Ling V. A surface glycoprotein modulating drug permeability in Chinese hamster ovary cell mutants. Biochim Biophys Acta 1976;455:152-62. [Crossref] [PubMed]
- Sjöstedt N, Holvikari K, Tammela P, et al. Inhibition of Breast Cancer Resistance Protein and Multidrug Resistance Associated Protein 2 by Natural Compounds and Their Derivatives. Mol Pharm 2017;14:135-46. [Crossref] [PubMed]
- Orelle C, Mathieu K, Jault JM. Multidrug ABC transporters in bacteria. Res Microbiol 2019;170:381-91. [Crossref] [PubMed]
- Alam A, Locher KP. Structure and Mechanism of Human ABC Transporters. Annu Rev Biophys 2023;52:275-300. [Crossref] [PubMed]
- Rosenberg MF, Bikadi Z, Hazai E, et al. Three-dimensional structure of the human breast cancer resistance protein (BCRP/ABCG2) in an inward-facing conformation. Acta Crystallogr D Biol Crystallogr 2015;71:1725-35. [Crossref] [PubMed]
- Buechel ER, Pinkett HW. Transcription factors and ABC transporters: from pleiotropic drug resistance to cellular signaling in yeast. FEBS Lett 2020;594:3943-64. [Crossref] [PubMed]
- Mineiro R, Santos C, Gonçalves I, et al. Regulation of ABC transporters by sex steroids may explain differences in drug resistance between sexes. J Physiol Biochem 2023;79:467-87. [Crossref] [PubMed]
- Koshiba S, An R, Saito H, et al. Human ABC transporters ABCG2 (BCRP) and ABCG4. Xenobiotica 2008;38:863-88. [Crossref] [PubMed]
- Tian Y, Bian Y, Jiang Y, et al. Interplay of Breast Cancer Resistance Protein (BCRP) and Metabolizing Enzymes. Curr Drug Metab 2015;16:877-93. [Crossref] [PubMed]
- Dong J, Yuan L, Hu C, et al. Strategies to overcome cancer multidrug resistance (MDR) through targeting P-glycoprotein (ABCB1): An updated review. Pharmacol Ther 2023;249:108488. [Crossref] [PubMed]
- Choudhuri S, Klaassen CD. Structure, function, expression, genomic organization, and single nucleotide polymorphisms of human ABCB1 (MDR1), ABCC (MRP), and ABCG2 (BCRP) efflux transporters. Int J Toxicol 2006;25:231-59. [Crossref] [PubMed]
- George AM. ABC Transporters 45 Years On. Int J Mol Sci 2023;24:16789. [Crossref] [PubMed]
- Szakács G, Paterson JK, Ludwig JA, et al. Targeting multidrug resistance in cancer. Nat Rev Drug Discov 2006;5:219-34. [Crossref] [PubMed]
- Pote MS, Gacche RN. ATP-binding cassette efflux transporters and MDR in cancer. Drug Discov Today 2023;28:103537. [Crossref] [PubMed]
- Sarkadi B, Homolya L, Hegedűs T. The ABCG2/BCRP transporter and its variants - from structure to pathology. FEBS Lett 2020;594:4012-34. [Crossref] [PubMed]
- Ieiri I. Functional significance of genetic polymorphisms in P-glycoprotein (MDR1, ABCB1) and breast cancer resistance protein (BCRP, ABCG2). Drug Metab Pharmacokinet 2012;27:85-105. [Crossref] [PubMed]
- Mao Q, Unadkat JD. Role of the breast cancer resistance protein (BCRP/ABCG2) in drug transport--an update. AAPS J 2015;17:65-82. [Crossref] [PubMed]
- Tanaka Y, Slitt AL, Leazer TM, et al. Tissue distribution and hormonal regulation of the breast cancer resistance protein (Bcrp/Abcg2) in rats and mice. Biochem Biophys Res Commun 2005;326:181-7. [Crossref] [PubMed]
- Taylor NMI, Manolaridis I, Jackson SM, et al. Structure of the human multidrug transporter ABCG2. Nature 2017;546:504-9. [Crossref] [PubMed]
- Safar Z, Kis E, Erdo F, et al. ABCG2/BCRP: variants, transporter interaction profile of substrates and inhibitors. Expert Opin Drug Metab Toxicol 2019;15:313-28. [Crossref] [PubMed]
- Fukuda Y, Schuetz JD. ABC transporters and their role in nucleoside and nucleotide drug resistance. Biochem Pharmacol 2012;83:1073-83. [Crossref] [PubMed]
- Mehendale-Munj S, Sawant S. Breast Cancer Resistance Protein: A Potential Therapeutic Target for Cancer. Curr Drug Targets 2021;22:420-8. [Crossref] [PubMed]
- Doyle L, Ross DD. Multidrug resistance mediated by the breast cancer resistance protein BCRP (ABCG2). Oncogene 2003;22:7340-58. [Crossref] [PubMed]
- Khunweeraphong N, Stockner T, Kuchler K. The structure of the human ABC transporter ABCG2 reveals a novel mechanism for drug extrusion. Sci Rep 2017;7:13767. [Crossref] [PubMed]
- Zhang W, Ding W, Chen Y, et al. Up-regulation of breast cancer resistance protein plays a role in HER2-mediated chemoresistance through PI3K/Akt and nuclear factor-kappa B signaling pathways in MCF7 breast cancer cells. Acta Biochim Biophys Sin (Shanghai) 2011;43:647-53. [Crossref] [PubMed]
- Eckenstaler R, Benndorf RA. 3D structure of the transporter ABCG2-What's new? Br J Pharmacol 2020;177:1485-96. [Crossref] [PubMed]
- Ferreira RJ, Bonito CA, Cordeiro MNDS, et al. Structure-function relationships in ABCG2: insights from molecular dynamics simulations and molecular docking studies. Sci Rep 2017;7:15534. [Crossref] [PubMed]
- Juvale K, Wiese M. Design of inhibitors of BCRP/ABCG2. Future Med Chem 2015;7:1521-7. [Crossref] [PubMed]
- Natarajan K, Xie Y, Baer MR, et al. Role of breast cancer resistance protein (BCRP/ABCG2) in cancer drug resistance. Biochem Pharmacol 2012;83:1084-103. [Crossref] [PubMed]
- Hira D, Terada T. BCRP/ABCG2 and high-alert medications: Biochemical, pharmacokinetic, pharmacogenetic, and clinical implications. Biochem Pharmacol 2018;147:201-10. [Crossref] [PubMed]
- de Bruin M, Miyake K, Litman T, et al. Reversal of resistance by GF120918 in cell lines expressing the ABC half-transporter, MXR. Cancer Lett 1999;146:117-26. [Crossref] [PubMed]
- Qadir M, O'Loughlin KL, Fricke SM, et al. Cyclosporin A is a broad-spectrum multidrug resistance modulator. Clin Cancer Res 2005;11:2320-6. [Crossref] [PubMed]
- Minderman H, O'Loughlin KL, Pendyala L, et al. VX-710 (biricodar) increases drug retention and enhances chemosensitivity in resistant cells overexpressing P-glycoprotein, multidrug resistance protein, and breast cancer resistance protein. Clin Cancer Res 2004;10:1826-34. [Crossref] [PubMed]
- Robey RW, Steadman K, Polgar O, et al. Pheophorbide a is a specific probe for ABCG2 function and inhibition. Cancer Res 2004;64:1242-6. [Crossref] [PubMed]
- Fletcher JI, Williams RT, Henderson MJ, et al. ABC transporters as mediators of drug resistance and contributors to cancer cell biology. Drug Resist Updat 2016;26:1-9. [Crossref] [PubMed]
- Li W, Zhang H, Assaraf YG, et al. Overcoming ABC transporter-mediated multidrug resistance: Molecular mechanisms and novel therapeutic drug strategies. Drug Resist Updat 2016;27:14-29. [Crossref] [PubMed]
- Kater L, Claffey J, Hogan M, et al. The role of the intrinsic FAS pathway in Titanocene Y apoptosis: The mechanism of overcoming multiple drug resistance in malignant leukemia cells. Toxicol In Vitro 2012;26:119-24. [Crossref] [PubMed]
- Gibalová L, Sereš M, Rusnák A, et al. P-glycoprotein depresses cisplatin sensitivity in L1210 cells by inhibiting cisplatin-induced caspase-3 activation. Toxicol In Vitro 2012;26:435-44. [Crossref] [PubMed]
- Wang QP, Wang Y, Wang XD, et al. Survivin up-regulates the expression of breast cancer resistance protein (BCRP) through attenuating the suppression of p53 on NF-κB expression in MCF-7/5-FU cells. Int J Biochem Cell Biol 2013;45:2036-44. [Crossref] [PubMed]
- Zhang YK, Wang YJ, Gupta P, et al. Multidrug Resistance Proteins (MRPs) and Cancer Therapy. AAPS J 2015;17:802-12. [Crossref] [PubMed]
- Xiao JB, Leng AM, Zhang YQ, et al. CUEDC2: multifunctional roles in carcinogenesis. Front Biosci (Landmark Ed) 2019;24:935-46. [Crossref] [PubMed]
- Pan X, Zhou T, Tai YH, et al. Elevated expression of CUEDC2 protein confers endocrine resistance in breast cancer. Nat Med 2011;17:708-14. [Crossref] [PubMed]
- Wang Y, Wang X, Zhao H, et al. Clusterin confers resistance to TNF-alpha-induced apoptosis in breast cancer cells through NF-kappaB activation and Bcl-2 overexpression. J Chemother 2012;24:348-57. [Crossref] [PubMed]
- Hamilton G, Abraham AG, Morton J, et al. AKT regulates NPM dependent ARF localization and p53mut stability in tumors. Oncotarget 2014;5:6142-67. [Crossref] [PubMed]
- Yu ACY, Chern YJ, Zhang P, et al. Inhibition of nucleophosmin 1 suppresses colorectal cancer tumor growth of patient -derived xenografts via activation of p53 and inhibition of AKT. Cancer Biol Ther 2021;22:112-23. [Crossref] [PubMed]
- Chen CY, Lin YS, Chen CH, et al. Annexin A2-mediated cancer progression and therapeutic resistance in nasopharyngeal carcinoma. J Biomed Sci 2018;25:30. [Crossref] [PubMed]
- Gorczyca L, Aleksunes LM. Transcription factor-mediated regulation of the BCRP/ABCG2 efflux transporter: a review across tissues and species. Expert Opin Drug Metab Toxicol 2020;16:239-53. [Crossref] [PubMed]
- Zhang F, Zhang L, Zhang B, et al. Anxa2 plays a critical role in enhanced invasiveness of the multidrug resistant human breast cancer cells. J Proteome Res 2009;8:5041-7. [Crossref] [PubMed]
- Wang X, Wu X, Wang C, et al. Transcriptional suppression of breast cancer resistance protein (BCRP) by wild-type p53 through the NF-kappaB pathway in MCF-7 cells. FEBS Lett 2010;584:3392-7. [Crossref] [PubMed]
- Tommasi C, Airò G, Pratticò F, et al. Hormone Receptor-Positive/HER2-Positive Breast Cancer: Hormone Therapy and Anti-HER2 Treatment: An Update on Treatment Strategies. J Clin Med 2024;13:1873. [Crossref] [PubMed]
- Ee PL, Kamalakaran S, Tonetti D, et al. Identification of a novel estrogen response element in the breast cancer resistance protein (ABCG2) gene. Cancer Res 2004;64:1247-51. [Crossref] [PubMed]
- Zhang Y, Wang H, Wei L, et al. Transcriptional modulation of BCRP gene to reverse multidrug resistance by toremifene in breast adenocarcinoma cells. Breast Cancer Res Treat 2010;123:679-89. [Crossref] [PubMed]
- Pradhan M, Bembinster LA, Baumgarten SC, et al. Proinflammatory cytokines enhance estrogen-dependent expression of the multidrug transporter gene ABCG2 through estrogen receptor and NF{kappa}B cooperativity at adjacent response elements. J Biol Chem 2010;285:31100-6. [Crossref] [PubMed]
- Parise CA, Caggiano V. Breast Cancer Survival Defined by the ER/PR/HER2 Subtypes and a Surrogate Classification according to Tumor Grade and Immunohistochemical Biomarkers. J Cancer Epidemiol 2014;2014:469251. [Crossref] [PubMed]
- Wang H, Lee EW, Zhou L, et al. Progesterone receptor (PR) isoforms PRA and PRB differentially regulate expression of the breast cancer resistance protein in human placental choriocarcinoma BeWo cells. Mol Pharmacol 2008;73:845-54. [Crossref] [PubMed]
- Vore M, Leggas M. Progesterone acts via progesterone receptors A and B to regulate breast cancer resistance protein expression. Mol Pharmacol 2008;73:613-5. [Crossref] [PubMed]
- Kukal S, Bora S, Kanojia N, et al. Valproic Acid-Induced Upregulation of Multidrug Efflux Transporter ABCG2/BCRP via PPARα-Dependent Mechanism in Human Brain Endothelial Cells. Mol Pharmacol 2023;103:145-57. [Crossref] [PubMed]
- Lin Y, Bircsak KM, Gorczyca L, et al. Regulation of the placental BCRP transporter by PPAR gamma. J Biochem Mol Toxicol 2017;31: [Crossref] [PubMed]
- Albermann N, Schmitz-Winnenthal FH, Z'graggen K, et al. Expression of the drug transporters MDR1/ABCB1, MRP1/ABCC1, MRP2/ABCC2, BCRP/ABCG2, and PXR in peripheral blood mononuclear cells and their relationship with the expression in intestine and liver. Biochem Pharmacol 2005;70:949-58. [Crossref] [PubMed]
- Nagai K, Fukuno S, Yamamoto K, et al. Downregulation of organic cation transporter 1 and breast cancer resistance protein with the induction of Pregnane X receptor in rat kidney impaired by doxorubicin. Pharmazie 2019;74:744-6. [PubMed]
- Krishnamurthy P, Ross DD, Nakanishi T, et al. The stem cell marker Bcrp/ABCG2 enhances hypoxic cell survival through interactions with heme. J Biol Chem 2004;279:24218-25. [Crossref] [PubMed]
- He M, Wu H, Jiang Q, et al. Hypoxia-inducible factor-2α directly promotes BCRP expression and mediates the resistance of ovarian cancer stem cells to adriamycin. Mol Oncol 2019;13:403-21. [Crossref] [PubMed]
- Pinzón-Daza ML, Cuellar-Saenz Y, Nualart F, et al. Oxidative Stress Promotes Doxorubicin-Induced Pgp and BCRP Expression in Colon Cancer Cells Under Hypoxic Conditions. J Cell Biochem 2017;118:1868-78. [Crossref] [PubMed]
- Francois LN, Gorczyca L, Du J, et al. Down-regulation of the placental BCRP/ABCG2 transporter in response to hypoxia signaling. Placenta 2017;51:57-63. [Crossref] [PubMed]
- Tan KP, Wang B, Yang M, et al. Aryl hydrocarbon receptor is a transcriptional activator of the human breast cancer resistance protein (BCRP/ABCG2). Mol Pharmacol 2010;78:175-85. [Crossref] [PubMed]
- Neradugomma NK, Liao MZ, Mao Q. Buprenorphine, Norbuprenorphine, R-Methadone, and S-Methadone Upregulate BCRP/ABCG2 Expression by Activating Aryl Hydrocarbon Receptor in Human Placental Trophoblasts. Mol Pharmacol 2017;91:237-49. [Crossref] [PubMed]
- Kathawala RJ, Gupta P, Ashby CR Jr, et al. The modulation of ABC transporter-mediated multidrug resistance in cancer: a review of the past decade. Drug Resist Updat 2015;18:1-17. [Crossref] [PubMed]
- Kukal S, Guin D, Rawat C, et al. Multidrug efflux transporter ABCG2: expression and regulation. Cell Mol Life Sci 2021;78:6887-939. [Crossref] [PubMed]
- Verma K, Jaiswal R, Paliwal S, et al. An insight into PI3k/Akt pathway and associated protein-protein interactions in metabolic syndrome: A recent update. J Cell Biochem 2023;124:923-42. [Crossref] [PubMed]
- Glaviano A, Foo ASC, Lam HY, et al. PI3K/AKT/mTOR signaling transduction pathway and targeted therapies in cancer. Mol Cancer 2023;22:138. [Crossref] [PubMed]
- Liu R, Chen Y, Liu G, et al. PI3K/AKT pathway as a key link modulates the multidrug resistance of cancers. Cell Death Dis 2020;11:797. [Crossref] [PubMed]
- Jung KA, Choi BH, Kwak MK. The c-MET/PI3K signaling is associated with cancer resistance to doxorubicin and photodynamic therapy by elevating BCRP/ABCG2 expression. Mol Pharmacol 2015;87:465-76. [Crossref] [PubMed]
- Nakanishi T, Ross DD. Breast cancer resistance protein (BCRP/ABCG2): its role in multidrug resistance and regulation of its gene expression. Chin J Cancer 2012;31:73-99. [Crossref] [PubMed]
- Komeili-Movahhed T, Fouladdel S, Barzegar E, et al. PI3K/Akt inhibition and down-regulation of BCRP re-sensitize MCF7 breast cancer cell line to mitoxantrone chemotherapy. Iran J Basic Med Sci 2015;18:472-7. [PubMed]
- Takada T, Suzuki H, Gotoh Y, et al. Regulation of the cell surface expression of human BCRP/ABCG2 by the phosphorylation state of Akt in polarized cells. Drug Metab Dispos 2005;33:905-9. [Crossref] [PubMed]
- Drosten M, Barbacid M. Targeting the MAPK Pathway in KRAS-Driven Tumors. Cancer Cell 2020;37:543-50. [Crossref] [PubMed]
- Meyer zu Schwabedissen HE, Grube M, Dreisbach A, et al. Epidermal growth factor-mediated activation of the map kinase cascade results in altered expression and function of ABCG2 (BCRP). Drug Metab Dispos 2006;34:524-33. [Crossref] [PubMed]
- Tomiyasu H, Watanabe M, Sugita K, et al. Regulations of ABCB1 and ABCG2 expression through MAPK pathways in acute lymphoblastic leukemia cell lines. Anticancer Res 2013;33:5317-23. [PubMed]
- Xie J, Jin B, Li DW, et al. ABCG2 regulated by MAPK pathways is associated with cancer progression in laryngeal squamous cell carcinoma. Am J Cancer Res 2014;4:698-709. [PubMed]
- Tomiyasu H, Goto-Koshino Y, Fujino Y, et al. The regulation of the expression of ABCG2 gene through mitogen-activated protein kinase pathways in canine lymphoid tumor cell lines. J Vet Med Sci 2014;76:237-42. [Crossref] [PubMed]
- Jiang J. Hedgehog signaling mechanism and role in cancer. Semin Cancer Biol 2022;85:107-22. [Crossref] [PubMed]
- Bhateja P, Cherian M, Majumder S, et al. The Hedgehog Signaling Pathway: A Viable Target in Breast Cancer? Cancers (Basel) 2019;11:1126. [Crossref] [PubMed]
- Nguyen NM, Cho J. Hedgehog Pathway Inhibitors as Targeted Cancer Therapy and Strategies to Overcome Drug Resistance. Int J Mol Sci 2022;23:1733. [Crossref] [PubMed]
- Shahi MH, Rey JA, Castresana JS. The sonic hedgehog-GLI1 signaling pathway in brain tumor development. Expert Opin Ther Targets 2012;16:1227-38. [Crossref] [PubMed]
- Bian XH, Sun H, Xue H, et al. Expression and clinical significance of Shh/Gli-1 in papillary thyroid carcinoma. Tumour Biol 2014;35:10523-8. [Crossref] [PubMed]
- Ramaswamy B, Lu Y, Teng KY, et al. Hedgehog signaling is a novel therapeutic target in tamoxifen-resistant breast cancer aberrantly activated by PI3K/AKT pathway. Cancer Res 2012;72:5048-59. [Crossref] [PubMed]
- Ejendal KF, Diop NK, Schweiger LC, et al. The nature of amino acid 482 of human ABCG2 affects substrate transport and ATP hydrolysis but not substrate binding. Protein Sci 2006;15:1597-607. [Crossref] [PubMed]
- Noguchi K, Katayama K, Mitsuhashi J, et al. Functions of the breast cancer resistance protein (BCRP/ABCG2) in chemotherapy. Adv Drug Deliv Rev 2009;61:26-33. [Crossref] [PubMed]
- Hazai E, Bikádi Z. Homology modeling of breast cancer resistance protein (ABCG2). J Struct Biol 2008;162:63-74. [Crossref] [PubMed]
- Yanase K, Tsukahara S, Mitsuhashi J, et al. Functional SNPs of the breast cancer resistance protein-therapeutic effects and inhibitor development. Cancer Lett 2006;234:73-80. [Crossref] [PubMed]
- Sugimoto Y, Tsukahara S, Ishikawa E, et al. Breast cancer resistance protein: molecular target for anticancer drug resistance and pharmacokinetics/pharmacodynamics. Cancer Sci 2005;96:457-65. [Crossref] [PubMed]
- Cascorbi I. Role of pharmacogenetics of ATP-binding cassette transporters in the pharmacokinetics of drugs. Pharmacol Ther 2006;112:457-73. [Crossref] [PubMed]
- Deng F, Sjöstedt N, Santo M, et al. Novel inhibitors of breast cancer resistance protein (BCRP, ABCG2) among marketed drugs. Eur J Pharm Sci 2023;181:106362. [Crossref] [PubMed]
- Liu K, Zhu J, Huang Y, et al. Metabolism of KO143, an ABCG2 inhibitor. Drug Metab Pharmacokinet 2017;32:193-200. [Crossref] [PubMed]
- Shukla S, Zaher H, Hartz A, et al. Curcumin inhibits the activity of ABCG2/BCRP1, a multidrug resistance-linked ABC drug transporter in mice. Pharm Res 2009;26:480-7. [Crossref] [PubMed]
- Limtrakul P, Chearwae W, Shukla S, et al. Modulation of function of three ABC drug transporters, P-glycoprotein (ABCB1), mitoxantrone resistance protein (ABCG2) and multidrug resistance protein 1 (ABCC1) by tetrahydrocurcumin, a major metabolite of curcumin. Mol Cell Biochem 2007;296:85-95. [Crossref] [PubMed]
- Zhou Q, Ye M, Lu Y, et al. Curcumin Improves the Tumoricidal Effect of Mitomycin C by Suppressing ABCG2 Expression in Stem Cell-Like Breast Cancer Cells. PLoS One 2015;10:e0136694. [Crossref] [PubMed]
- Ozvegy-Laczka C, Hegedus T, Várady G, et al. High-affinity interaction of tyrosine kinase inhibitors with the ABCG2 multidrug transporter. Mol Pharmacol 2004;65:1485-95. [Crossref] [PubMed]
- Shi Z, Peng XX, Kim IW, et al. Erlotinib (Tarceva, OSI-774) antagonizes ATP-binding cassette subfamily B member 1 and ATP-binding cassette subfamily G member 2-mediated drug resistance. Cancer Res 2007;67:11012-20. [Crossref] [PubMed]
- Peña-Solórzano D, Stark SA, König B, et al. ABCG2/BCRP: Specific and Nonspecific Modulators. Med Res Rev 2017;37:987-1050. [Crossref] [PubMed]
- Houghton PJ, Germain GS, Harwood FC, et al. Imatinib mesylate is a potent inhibitor of the ABCG2 (BCRP) transporter and reverses resistance to topotecan and SN-38 in vitro. Cancer Res 2004;64:2333-7. [Crossref] [PubMed]
- Hegedüs C, Truta-Feles K, Antalffy G, et al. Interaction of the EGFR inhibitors gefitinib, vandetanib, pelitinib and neratinib with the ABCG2 multidrug transporter: implications for the emergence and reversal of cancer drug resistance. Biochem Pharmacol 2012;84:260-7. [Crossref] [PubMed]
- Dai CL, Tiwari AK, Wu CP, et al. Lapatinib (Tykerb, GW572016) reverses multidrug resistance in cancer cells by inhibiting the activity of ATP-binding cassette subfamily B member 1 and G member 2. Cancer Res 2008;68:7905-14. [Crossref] [PubMed]
- Sato H, Siddig S, Uzu M, et al. Elacridar enhances the cytotoxic effects of sunitinib and prevents multidrug resistance in renal carcinoma cells. Eur J Pharmacol 2015;746:258-66. [Crossref] [PubMed]
- Gupta A, Zhang Y, Unadkat JD, et al. HIV protease inhibitors are inhibitors but not substrates of the human breast cancer resistance protein (BCRP/ABCG2). J Pharmacol Exp Ther 2004;310:334-41. [Crossref] [PubMed]
- Weiss J, Rose J, Storch CH, et al. Modulation of human BCRP (ABCG2) activity by anti-HIV drugs. J Antimicrob Chemother 2007;59:238-45. [Crossref] [PubMed]
- Zhang Y, Gupta A, Wang H, et al. BCRP transports dipyridamole and is inhibited by calcium channel blockers. Pharm Res 2005;22:2023-34. [Crossref] [PubMed]
- Takara K, Matsubara M, Yamamoto K, et al. Differential effects of calcium antagonists on ABCG2/BCRP-mediated drug resistance and transport in SN-38-resistant HeLa cells. Mol Med Rep 2012;5:603-9. [PubMed]
- Chu X, Cai X, Cui D, et al. In vitro assessment of drug-drug interaction potential of boceprevir associated with drug metabolizing enzymes and transporters. Drug Metab Dispos 2013;41:668-81. [Crossref] [PubMed]
- Fujita Y, Noguchi K, Suzuki T, et al. Biochemical interaction of anti-HCV telaprevir with the ABC transporters P-glycoprotein and breast cancer resistance protein. BMC Res Notes 2013;6:445. [Crossref] [PubMed]
- Gupta A, Unadkat JD, Mao Q. Interactions of azole antifungal agents with the human breast cancer resistance protein (BCRP). J Pharm Sci 2007;96:3226-35. [Crossref] [PubMed]
- Weidner LD, Zoghbi SS, Lu S, et al. The Inhibitor Ko143 Is Not Specific for ABCG2. J Pharmacol Exp Ther 2015;354:384-93. [Crossref] [PubMed]
- Poirier A, Portmann R, Cascais AC, et al. The need for human breast cancer resistance protein substrate and inhibition evaluation in drug discovery and development: why, when, and how? Drug Metab Dispos 2014;42:1466-77. [Crossref] [PubMed]
- Han X, Deng S, Wang N, et al. Inhibitory effects and molecular mechanisms of tetrahydrocurcumin against human breast cancer MCF-7 cells. Food Nutr Res 2016;60:30616. [Crossref] [PubMed]
- Ni W, Fan H, Zheng X, et al. Cryptotanshinone Inhibits ERα-Dependent and -Independent BCRP Oligomer Formation to Reverse Multidrug Resistance in Breast Cancer. Front Oncol 2021;11:624811. [Crossref] [PubMed]
- Yamazaki R, Nishiyama Y, Furuta T, et al. Novel acrylonitrile derivatives, YHO-13177 and YHO-13351, reverse BCRP/ABCG2-mediated drug resistance in vitro and in vivo. Mol Cancer Ther 2011;10:1252-63. [Crossref] [PubMed]
- Pan G, Giri N, Elmquist WF. Abcg2/Bcrp1 mediates the polarized transport of antiretroviral nucleosides abacavir and zidovudine. Drug Metab Dispos 2007;35:1165-73. [Crossref] [PubMed]
- Kühnle M, Egger M, Müller C, et al. Potent and selective inhibitors of breast cancer resistance protein (ABCG2) derived from the p-glycoprotein (ABCB1) modulator tariquidar. J Med Chem 2009;52:1190-7. [Crossref] [PubMed]
- Valdameri G, Genoux-Bastide E, Peres B, et al. Substituted chromones as highly potent nontoxic inhibitors, specific for the breast cancer resistance protein. J Med Chem 2012;55:966-70. [Crossref] [PubMed]
- Wang Q, Cheng Y, Wang Y, et al. Tamoxifen reverses epithelial-mesenchymal transition by demethylating miR-200c in triple-negative breast cancer cells. BMC Cancer 2017;17:492. [Crossref] [PubMed]
- van Loevezijn A, Allen JD, Schinkel AH, et al. Inhibition of BCRP-mediated drug efflux by fumitremorgin-type indolyl diketopiperazines. Bioorg Med Chem Lett 2001;11:29-32. [Crossref] [PubMed]
- Yang CH, Chen YC, Kuo ML. Novobiocin sensitizes BCRP/MXR/ABCP overexpressing topotecan-resistant human breast carcinoma cells to topotecan and mitoxantrone. Anticancer Res 2003;23:2519-23. [PubMed]
- Woehlecke H, Osada H, Herrmann A, et al. Reversal of breast cancer resistance protein-mediated drug resistance by tryprostatin A. Int J Cancer 2003;107:721-8. [Crossref] [PubMed]
- Zhang S, Yang X, Morris ME. Flavonoids are inhibitors of breast cancer resistance protein (ABCG2)-mediated transport. Mol Pharmacol 2004;65:1208-16. [Crossref] [PubMed]
- Breedveld P, Zelcer N, Pluim D, et al. Mechanism of the pharmacokinetic interaction between methotrexate and benzimidazoles: potential role for breast cancer resistance protein in clinical drug-drug interactions. Cancer Res 2004;64:5804-11. [Crossref] [PubMed]