Novel toxicology challenges in the era of chimeric antigen receptor T-cells therapies
Introduction
T-lymphocytes are fine executioners of adaptive immune-responses, however, quantitative and qualitative immunological defects in patients with cancer (1), together with tumor immune-evasion strategies can result in inefficient anti-tumor surveillance (2). Genetically engineered T-cells, redirected towards the CD19 antigen with chimeric antigen receptors (CARs), have proven successful in treating patients with relapsed/refractory acute lymphoblastic leukemia (ALL). In addition, CAR T-cells redirected towards tumor associated antigens are actively being investigated in a variety of malignancies, including solid cancers (3). This novel form of treatment has been considered one of the recent revolutions in the treatment of CD19+ ALL. Immunotherapies are also appealing to patients, as they are considered a ‘milder’ form of treatment when compared to cytotoxic drugs. The latter are often used as lympho-depleting agents in order to create immunological space resulting in the production of homeostatic cytokines that favor the expansion of the infused T-cells. However, immunological therapies are not without risks and there is rapid recognition of their unique and challenging toxicities.
CAR T-cell toxicities can be broadly classified into: (I) “on-target off-tumor” effects, due to the targeted antigen expression not only on tumor cells but on normal tissues as well, the most common example is hypogammaglobulinemia from targeting normal B cells with CAR CD19 (managed with intravenous immunoglobulin replacement), albeit a case of fatal lung toxicity has also been reported (4); (II) anaphylactic reactions due to the development of anti-mouse antibodies; (III) tumor lysis syndrome (TLS); or (IV) cytokine release syndrome (CRS) (3).
In the May 2016 issue of Blood, Brudno and Kochenderfer (5) presented a clear and exhaustive review of the toxicities observed in clinical trials employing CAR T-cells redirected against CD19 with a focus on the most common acute toxicity observed in patients receiving CAR T-cells, the so-called CRS, with the currently accepted management strategies.
CRS
CRS is related to the in vivo expansion and activation of the infused cells resulting in a systemic inflammatory response. CRS manifests primarily with fever, tachycardia, hypotension, hypoxia, renal injury, hematologic abnormalities including disseminated intravascular coagulation, cytopenias with increased risk of infection, liver function abnormalities, gastrointestinal symptoms, myalgia/weakness, and neurologic manifestations (5). Neurologic toxicity may also occur as a distinct entity after CRS has resolved.
The exact pathogenesis of CRS is not completely understood, however it results from an amplification cascade of cytokine production from activated T-cells and other cells of the immune system, with a constellation of symptoms sometimes indistinguishable from hemophagocytic lymphohistiocytosis or macrophage activation syndrome.
Upregulation of several cytokines has been reported in the blood of patients with CRS, especially interleukin-6 (IL-6), IL-6 receptor IL-6R (CD126), interferon-gamma (IFN-gamma), tumor necrosis factor alpha (TNF-alpha), IL-2, IL-2R-alpha, IL-8, and IL-10 (5).
IL-6 is a pleiotropic cytokine with anti-inflammatory and proinflammatory properties that has been recognized as one of the principal mediators of CRS toxicity. IL-6 exerts anti-inflammatory effects when present at low levels, signaling through the IL-6 receptor (IL-6R) (CD126) expressed on macrophages, neutrophils, hepatocytes, and some T cells subsets (classic signaling). This leads to homodimerization of the gp130 molecule (CD130) with activation of downstream pathways only on cells expressing the IL-6R. However, at high levels (as in CRS), IL-6 exerts pro-inflammatory effects due to binding to soluble IL-6R and cross-signaling with activation of gp130 on a wider array of target cells with ubiquitous expression (6).
Based on the fact that CARs endowed with IgG1 Fc spacer domain bind to IgG Fc gamma receptors (Fc-gamma-Rs), they can unintentionally activate innate immune cells with consequent secretion of pro-inflammatory cytokines. In order to reduce Fc-gamma-Rs binding, Hombach et al. (7) modified the PELLGG ISR Fc-gamma-R binding domain to PPVA-G IAR domain and demonstrated lack of activation in presence of Fc-gamma-Rs cells. Since engineered T cells are likewise activated by Fc-gamma-Rs binding with resulting cytokines secretion and lysis of monocytes and NK cells independently of the redirected specificity, this strategy could minimize the risk of off-target activation without affecting CAR expression or their redirected targeting specificity. Based on those findings, we implemented similar modifications in the CAR constructs that we are currently validating in the pre-clinical setting. Alternatively to using an IgG1 spacer, Memorial Sloan Kettering Cancer Center (8) and University of Pennsylvania (9) used human CD8-alpha hinge and transmembrane (TM) domains, whereas the National Cancer Institute (NCI) used CD28 hinge and TM domains (10), neither of which are expected to bind to Fc-gamma-Rs.
Management of CRS
CRS manifestations have been reported in approximately two thirds of patients treated with CAR CD19 T-cells, ranging from milder forms requiring only symptomatic support, to severe forms (in up to 50% of cases), requiring intensive care unit monitoring, pressor support, intubation, and even cardiac resuscitation. Thus, not every patient is suitable to receive CAR T-cells administration. In fact, as Brudno and Kochenderfer report, the NCI has established recommended eligibility criteria for patients receiving CAR-T as part of clinical trials, to ensure enrollment of patients with adequate functional reserve who could tolerate the eventual adverse events.
Prompt recognition and severity grading of clinical manifestations necessitating clinical support are paramount. The Common Terminology Criteria for Adverse Events Version 4 (CTCAE) includes a grading scale of CRS-related adverse events caused by immunotherapies. But this scale was created for toxicity grading of acute infusional toxicities of monoclonal antibodies; therefore, ad hoc grading criteria have been developed by the major centers involved in CAR T-cells administration studies (6,11). Although subdivided into different criteria, all scales range from a milder, grade 1 CRS that requires only symptomatic management to grade 4 toxicities that are potentially life threatening.
With the report of severe and fatal toxicities after CAR T-cells infusion, the Recombinant DNA Advisory Committee of the National Institute of Health developed clinical recommendations including implementing careful dose-escalation plans, especially in patients with higher disease burden who are at increased risks of developing CAR-related complications, such as TLS and CRS (12) (https://videocast.nih.gov/summary.asp?Live=16420&bhcp=1).
The recommendations differentiated between clinical trials based on the infusion of first generation CAR redirected T-cells [excluding Epstein-Barr virus (EBV)-specific-cytotoxic-T-cells], versus clinical trials based on the infusion of second/third generation CAR redirected T-cells, providing guidance on dosing, administration of cytokines or preconditioning treatment, as well as proposing split-dose infusion schedules (Table 1).
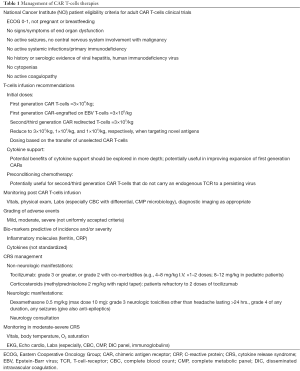
Full table
Although some centers perform CAR T-cells infusion in an outpatient setting, others follow strict inpatient monitoring. In either case, patients should be educated on the potential side effects that warrant prompt communication to the clinic, and close monitoring of vital signs, complete blood count, complete metabolic panel, microbiology studies, and imaging, as appropriate, with provisions to escalate care if needed.
The mostly common used therapeutic approach for patients developing CRS originally included the administration of corticosteroids which are able to exert an immune-suppressive and cytotoxic effect on T-lymphocytes. However, the observation that patients receiving corticosteroids for severe CRS experienced a reduction/disappearance of CAR T-cells followed by leukemia relapse, led the investigators to seek alternative treatments. Based on the observation that that serum IL-6 levels peaked during CRS, the use of the anti-IL-6R monoclonal antibody tocilizumab, a humanized immunoglobulin G1k (IgG1k) monoclonal antibody approved for the treatment of some rheumatologic/autoimmune diseases, has been proposed and utilized as first-line treatment for amelioration of CRS symptoms, with reduction in serum biomarkers including IL-6, (6,13,14). Tocilizumab administration it is usually indicated for patients with CRS of severe grade, or moderate grade but with co-morbidities.
In the review paper of Brudno and Kochenderfer, the authors report on the successful response to tocilizumab in two patients at their institution, one with CRS manifesting 4 hours after the infusion of CAR T-cells targeting the B-cell maturation antigen, and another who developed CRS with left ventricular dysfunction, resembling stress cardiomyopathy (Takotsubo cardiomyopathy), following CAR CD19 T-cells infusion for ALL. Patient 1 received tocilizumab infusion at 25 hours and at 5 days after T-cells infusion with sustained decrease in body temperature and heart rate, and patient 2 received tocilizumab on day 4 after T-cells infusion with decrease in respiratory rate, thereby avoiding an impending intubation, as well as decrease in heart rate and C-reactive protein (CRP). Corticosteroids (rapidly tapered methylprednisolone) are reserved for CRS toxicity cases refractory to tocilizumab. One exception is made for neurologic manifestations of CAR T-cell toxicity, where there is a general consensus that steroids are preferred because: (I) central nervous system (CNS) penetration of tocilizumab is poor; and (II) a concern that saturation of the IL-6 receptor by tocilizumab would lead to an increase of the IL-6 levels in the cerebrospinal fluid with subsequent worsening neurologic toxicity. Dexamethasone is the steroids of choice for CNS symptoms by virtue of its excellent CNS penetration. Table 1 summarizes the keypoints adopted in the management of CAR T-cells therapies.
Predicting CRS or its severity
Current research efforts aim at identifying serum bio-markers to predict the development and/or severity of CRS. In a recent publication, Teachey et al. (13) measured the levels of cytokines and biomarkers in 51 patients (39 of whom were pediatrics), treated with CAR CD19 T-cells for ALL. The authors reported peak levels of 24 cytokines (including IFN-gamma, IL-6, sgp30, and sIL-6R) in the first month after infusion that were associated with the development of severe CRS.
Tumor burden was associated with CRS severity, but inclusion of tumor burden in the prediction model was not able to improve the accuracy of the prediction based on cytokines alone (13), a finding that conflicts with two previous reports (11,15).
Furthermore, Teachey et al. did not find an appreciable rise in IL-6 prior to the development of CRS, as previously reported by Turtle and colleagues (15). With conflicting data, there is no current consensus on the pre-emptive administration of tocilizumab. Additionally, it is unclear if the cytokine elevation is an integral part of the anti-tumor response; pre-emptive administration of tocilizumab in this case, would dampen the anti-tumor effect of CAR T-cells.
Although CAR CD19 T-cells trials performed in ALL offer invaluable information, it is currently unclear whether the prediction models and therapeutic strategies adopted in patients with CAR CD19 T-cells toxicities, would apply to CAR targeting different antigens, and would apply to CAR T-cells clinical trials performed in both pediatric and adult patients.
Cytokine release platforms need consolidated assays design and format (6), ideally with uniform protocols for CRS testing as currently proposed by the International Life Science Institute-Health and Environmental Sciences Institute Immunotoxicology Technical Committee (ILSI-HESI ITC) workshop on cytokine assays (16).
It needs to be kept in mind that baseline inflammatory levels can be already elevated in patients with cancer, and a fold or net increase or rate of change in cytokine levels may be more informative than absolute cytokine levels. Since the use of currently identified biomarkers requires clinical laboratory improvement amendments (CLIA)-certified assays, which are not routinely available at many centers, some authors have proposed the use of surrogate CRS makers such as ferritin and CRP. Unfortunately, there are conflicting results regarding the utility of such surrogate markers to predict the development of severe CRS, and further validation studies are needed (11,13,15). CRP is an acute phase reactant produced by the liver largely in response to IL-6, however CRP levels (as well as ferritin levels) cannot be used to distinguish between infection-associated and noninfectious inflammation. Furthermore, CRS can result in pancytopenia, predisposing the patients to infectious complications, manifesting with a spectrum of signs and symptoms indistinguishable from sepsis. Since some authors found elevated levels of serum IFN-γ patients with CRS decreasing after treatment of CRS (13,15), this marker could be helpful in differentiating between the two, perhaps in combination with other early markers of sepsis, such as pro-calcitonin (17).
Other variables that might affect the inference of these approaches include, for example, the different targeted antigens, T-cell manufacturing protocols, the vector system utilized, the CAR design, the affinity of the single chain variable fragments (scFv), the choice of the co-stimulatory domain(s), the presence and type of preconditioning regimens, and/or cytokines administered before CAR T-cell infusion.
Utility of suicide genes in CRS
The Recombinant DNA Advisory Committee of the National Institute of Health recommendations also included the co-expression of the CAR with a suicide gene for switching-off unpredicted or controlling long-term toxicities (12). However, aside pre-clinical models (18), suicide gene strategies in combination with CARs have not yet been reported from the clinical setting, and it is unclear whether the activation of a suicide gene would abate the inflammatory response when already clinically manifest. Data from clinical trials of inducible Caspase9 (iC9) suicide gene modified T-cells infused after haploidentical hematopoietic stem cell transplantation showed, that after administration of the AP1903 dimerizer to activate the safety switch in patients with inflammatory responses during GVHD, the elimination of the infused cells resulted in defervescence and reduction of inflammatory cytokines, including serum IL-6. Additionally, some indirect preliminary evidence suggested a reduction in iC9-T-cells in the CNS following dimerizer administration (19). Other strategies to increase the safety of CAR T-cells by enhancing the activation of the CAR only towards the tumor targets, involve combinatorial antigen recognition (3), or controlling transgene expression via inducible promoters (20); however, also these strategies have not yet entered into clinical trials.
A potential concern for the development of neutralizing antibodies or even anaphylaxis arises if repeated administration of CAR T cells are employed after implementation of CRS treatments or suicide genes are activated. Humanization of murine monoclonal antibodies consists of the replacement of mouse constant regions and variable framework regions for human sequences, resulting in a significantly less immunogenic product. However, some humanized and even fully human sequence-derived antibody molecules still carry immunological risk, as evidenced by the detection of CD4+ T-cell epitopes in complementarity determining regions (CDR) (21). The authors of this report incorporated up to two amino acid modifications in a single epitope on the affinity-determining regions reducing the immunogenic potential while maintaining the bioactivity of the antibody molecule, and similar strategies could be applied to CAR scFv as well.
Conclusions
CAR T-cells based therapeutic clinical trials are increasingly performed worldwide with high expectations from scientists, clinicians and patients alike. The targeting of tumor associated antigens, often also expressed on normal tissues, and the activation of the immune system, is creating a new chapter in the science of toxicology. Multiple research questions are open in regard to the optimal CAR strategy design, which may vary according to the targeted antigen, but especially, in regard to prevention, prediction, diagnosis, and treatment of those emerging novel CAR-related toxicities. Finally, the Foundation for the Accreditation of Cellular Therapy (FACT) has published a draft of the 1st edition FACT Standards for Immune Effector Cell Administration for inspection and public comment, intended to promote quality in administration of immune effector cells. These standards will be incorporated into a voluntary FACT accreditation in this field (http://www.factwebsite.org/ImmuneEffectorRequest/).
Acknowledgments
We are grateful to Dr. Donna Salzman for editing the final version of the manuscript.
Funding: None
Footnote
Provenance and Peer Review: This article was commissioned and reviewed by the Section Editor Xia Fang (Department of hematology, Shanghai Tongji Hospital, Tongji University School of Medicine, Shanghai, China).
Conflicts of Interest: Both authors have completed the ICMJE uniform disclosure form (available at http://dx.doi.org/10.21037/tcr.2016.09.06). The authors have no conflicts of interest to declare.
Ethical Statement: The authors are accountable for all aspects of the work in ensuring that questions related to the accuracy or integrity of any part of the work are appropriately investigated and resolved.
Open Access Statement: This is an Open Access article distributed in accordance with the Creative Commons Attribution-NonCommercial-NoDerivs 4.0 International License (CC BY-NC-ND 4.0), which permits the non-commercial replication and distribution of the article with the strict proviso that no changes or edits are made and the original work is properly cited (including links to both the formal publication through the relevant DOI and the license). See: https://creativecommons.org/licenses/by-nc-nd/4.0/.
References
- Meeus M, Mistiaen W, Lambrecht L, et al. Immunological similarities between cancer and chronic fatigue syndrome: the common link to fatigue? Anticancer Res 2009;29:4717-26. [PubMed]
- Vinay DS, Ryan EP, Pawelec G, et al. Immune evasion in cancer: Mechanistic basis and therapeutic strategies. Semin Cancer Biol 2015;35:S185-98. [Crossref] [PubMed]
- Minagawa K, Zhou X, Mineishi S, et al. Seatbelts in CAR therapy: How Safe Are CARS? Pharmaceuticals (Basel) 2015;8:230-49. [Crossref] [PubMed]
- Morgan RA, Yang JC, Kitano M, et al. Case report of a serious adverse event following the administration of T cells transduced with a chimeric antigen receptor recognizing ERBB2. Mol Ther 2010;18:843-51. [Crossref] [PubMed]
- Brudno JN, Kochenderfer JN. Toxicities of chimeric antigen receptor T cells: recognition and management. Blood 2016;127:3321-30. [Crossref] [PubMed]
- Lee DW, Gardner R, Porter DL, et al. Current concepts in the diagnosis and management of cytokine release syndrome. Blood 2014;124:188-95. [Crossref] [PubMed]
- Hombach A, Hombach AA, Abken H. Adoptive immunotherapy with genetically engineered T cells: modification of the IgG1 Fc 'spacer' domain in the extracellular moiety of chimeric antigen receptors avoids 'off-target' activation and unintended initiation of an innate immune response. Gene Ther 2010;17:1206-13. [Crossref] [PubMed]
- Brentjens RJ, Latouche JB, Santos E, et al. Eradication of systemic B-cell tumors by genetically targeted human T lymphocytes co-stimulated by CD80 and interleukin-15. Nat Med 2003;9:279-86. [Crossref] [PubMed]
- Milone MC, Fish JD, Carpenito C, et al. Chimeric receptors containing CD137 signal transduction domains mediate enhanced survival of T cells and increased antileukemic efficacy in vivo. Mol Ther 2009;17:1453-64. [Crossref] [PubMed]
- Kochenderfer JN, Feldman SA, Zhao Y, et al. Construction and preclinical evaluation of an anti-CD19 chimeric antigen receptor. J Immunother 2009;32:689-702. [Crossref] [PubMed]
- Davila ML, Riviere I, Wang X, et al. Efficacy and toxicity management of 19-28z CAR T cell therapy in B cell acute lymphoblastic leukemia. Sci Transl Med 2014;6:224ra25 [Crossref] [PubMed]
- Ertl HC, Zaia J, Rosenberg SA, et al. Considerations for the clinical application of chimeric antigen receptor T cells: observations from a recombinant DNA Advisory Committee Symposium held June 15, 2010. Cancer Res 2011;71:3175-81. [Crossref] [PubMed]
- Teachey DT, Lacey SF, Shaw PA, et al. Identification of Predictive Biomarkers for Cytokine Release Syndrome after Chimeric Antigen Receptor T-cell Therapy for Acute Lymphoblastic Leukemia. Cancer Discov 2016;6:664-79. [Crossref] [PubMed]
- Grupp SA, Kalos M, Barrett D, et al. Chimeric antigen receptor-modified T cells for acute lymphoid leukemia. N Engl J Med 2013;368:1509-18. [Crossref] [PubMed]
- Turtle CJ, Hanafi LA, Berger C, et al. CD19 CAR-T cells of defined CD4+:CD8+ composition in adult B cell ALL patients. J Clin Invest 2016;126:2123-38. [Crossref] [PubMed]
- Grimaldi C, Finco D, Fort MM, et al. Cytokine release: A workshop proceedings on the state-of-the-science, current challenges and future directions. Cytokine 2016;85:101-8. [Crossref] [PubMed]
- Brunkhorst FM, Wegscheider K, Forycki ZF, et al. Procalcitonin for early diagnosis and differentiation of SIRS, sepsis, severe sepsis, and septic shock. Intensive Care Med 2000;26:S148-52. [Crossref] [PubMed]
- Jones BS, Lamb LS, Goldman F, et al. Improving the safety of cell therapy products by suicide gene transfer. Front Pharmacol 2014;5:254. [Crossref] [PubMed]
- Zhou X, Dotti G, Krance RA, et al. Inducible caspase-9 suicide gene controls adverse effects from alloreplete T cells after haploidentical stem cell transplantation. Blood 2015;125:4103-13. [Crossref] [PubMed]
- Sakemura R, Terakura S, Watanabe K, et al. A Tet-On Inducible System for Controlling CD19-Chimeric Antigen Receptor Expression upon Drug Administration. Cancer Immunol Res 2016;4:658-68. [Crossref] [PubMed]
- Harding FA, Stickler MM, Razo J, et al. The immunogenicity of humanized and fully human antibodies: residual immunogenicity resides in the CDR regions. MAbs 2010;2:256-65. [Crossref] [PubMed]