Another important step towards understanding tumor immune evasion—novel mechanisms of PD-L1 overexpression
Overview
Recent advancements in the search for new treatment strategies for cancer have led to the development of therapeutic agents that target specific molecules that are critical to cancer development and/or expansion. The inhibition of the programmed cell death-1 (PD-1)/programmed cell death ligand-1 (PD-L1) pathway is one of such upcoming strategies that is being extensively explored in the field of oncology. Immunotherapy agents targeting the PD-1/PD-L1 signaling pathway have demonstrated promising anti-tumor efficacy in several malignancies, including non-small cell lung cancer (NSCLC) and melanoma, among others (1-6). Tumor cells are known to overexpress PD-L1, which aids in immune evasion by inducing T cell anergy and exhaustion within the tumor microenvironment (7). Several chromosomal alterations, most notably 9p24.1 amplification [identified in Hodgkin’s lymphoma (HL) cell lines], are reported to result in overexpression of PD-1 ligands (8,9). In addition, endogenous molecules such as interferon-γ (IFNγ) have also been identified to induce PD-L1 overexpression (10,11). The ability of tumors to suppress the host immune response is considered to be central to the clinical benefit observed with immunotherapy agents targeting the PD-1/PD-L1 signaling pathway (8,12-17). The mechanistic relationship between evasion of endogenous immunity by cancer cells and overexpression of PD-1 ligands, however, is not fully understood.
Background
Several chromosomal alterations have been identified that result in overexpression of PD-1 ligands (Table 1). Analysis of HL cell lines revealed major histocompatibility complex (MHC) class II trans-activator CIITA gene fusions that resulted in PD-L1 and PD-L2 overexpression, along with decreased surface human leucocyte antigen (HLA) class II expression (20). Studies on B cell lymphoma noted a direct association between a decrease in tumor immune-surveillance and loss of MHC class II gene expression (23). The significance of chromosomal amplifications was appreciated in a study evaluating primary mediastinal large B cell lymphoma and nodular sclerosing Hodgkin lymphoma (NSHL) cell lines. It was observed that 9p24.1 amplification both directly and indirectly increased PD-L1 expression, through gene copy number gain and by amplification of janus kinase 2 (JAK2), respectively (8,9). PD-L1 overexpression has also been noted in Epstein Barr virus (EBV) positive HL without 9p24.1 amplification, thus suggesting an alternative pathway for PD-L1 overexpression in a subset of cases (8,21).
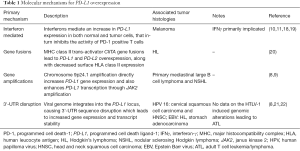
Full table
In addition to chromosomal aberrations, innate mechanisms have also been implicated in influencing PD-L1 expression in tumor cells (Table 1). According to the adaptive immune resistance theory, tumor cells adapt to the endogenous anti-tumor immune response, specifically the IFNγ cytokine, with increased PD-L1 expression (10,11). A study evaluating melanoma cells noted an association between intra-tumoral levels of tumor infiltration lymphocytes (TILs) and IFNγ with PD-L1 on tumor cells. The presence of a negative feedback mechanism has been speculated that downregulates PD1 positive T cells through IFNγ mediated an increase in PD-L1 expression (18). Our knowledge of various pathways that mediate immune escape of tumor cells has provided a foundation in the formulation of treatment strategies for various malignancies.
Understanding the biology that influence cancer immune evasion has been pivotal in the identification of PD-L1 as a target molecule for immunotherapy (16). It has also led to the development of diagnostic tests predictive of treatment outcomes with specific immunotherapy agents, such as PD-L1 IHC for pembrolizumab therapy in NSCLC (16,24). As we continue to elucidate additional mechanisms of cancer immune escape, it may be possible to accurately assess cancer immune biology on an individual basis that would in turn allow for a personalized anti-cancer immuno-oncology treatment regimen.
New advances
Recently, Kataoka and colleagues described a novel mechanism of cancer immune escape (Table 1). They utilized adult T cell leukemia/lymphoma (ATL) samples to study genomic structural variations that disrupted the 3' region of PD-L1 using a new platform (Genomon-SV) (22). These structural variations caused a significant increase in PD-L1 transcripts which were stabilized by 3'-untranslated region (3'-UTR) truncation. Further, in vivo experiments on murine models functionally demonstrated that PD-L1 3'-UTR disruption EG7-OVA tumor growth with increased PD-L1 expression and decreased CD8+ TILs. Thus, it was reckoned that specific structural variations, specifically those involved in disrupting the 3'-UTR of PD-L1, could potentially mediate clonal selection of tumor cell populations through immune escape and serve as genetic markers for tumors to target with checkpoint inhibitors.
This study made a number of noteworthy observations. Genome-wide mapping for breakpoints associated with structural variations identified several recurrent breakpoint clusters. Many of these significant breakpoints were located in a 3.1 kilobase segment at the 3' region of the PD-L1 locus in chromosome 9p24.1. Although several types of structural variations were noted, all of them resulted in an anomalous PD-L1 allele. However, the aberrant PD-L1 proteins generated from these abnormal transcripts exhibited functional transmembrane and extracellular receptor binding domains. The cases with these structural variations also displayed significantly increased PD-L1 expression and protein levels. Interestingly, these structural variations had no effect on PD-L2 expression. The above findings suggest that ATL cell populations with these structural variations were clonally selected through immune escape.
Encouraged by the evidence gathered in ATL, Kataoka and colleagues investigated if immune escape mediated clonal selection occurred in other tumor types (22). A total of 10,210 specimens from 33 tumor panels [RNA sequence data retrieved from The Cancer Genome Atlas (TCGA)] were evaluated for structural variations that involved the PD-L1 3'-UTR. Thirty-one samples from 13 types of tumors (including ATL) were reported to be positive for PD-L1 3'-UTR disruption and demonstrated overexpression of anomalous PD-L1 transcripts. This disruption significantly correlated with increased PD-L1 expression independent of PD-L1 gene copy number. Unlike PD-L1, the expression of JAK2 (also located on chromosome 9p24.1) showed no significant changes secondary to these structural variations. In order to assess the anti-tumor response, the samples positive for structural variation were further evaluated for cytolytic activity score, a marker of anti-tumor immune activity and cytotoxic T-cell infiltration (25). Cytolytic activity was revealed to have a prominent association with PD-L1 expression for every tumor type, and was similarly found to be elevated in cases with structural variations. Additionally, a comparison of samples with similar PD-L1 expression revealed that those positive for these structural variations had a considerably lower cytolytic activity, thereby indicating diminution of anti-tumor immunity. These findings further supported the primary hypothesis that tumor cells positive for specific structural variations undergo clonal selection attributable to PD-L1 overexpression and the resulting immune evasion.
This study has generated intriguing data on the effects of viral infection on PD-L1 expression. The authors reported on three TCGA cases in which integration of the viral genome resulted in aberrant PD-L1 transcripts. The viral DNA were identified to be from human papilloma virus (HPV) 16 in cervical squamous cell carcinoma and head and neck squamous cell carcinoma (HNSC), and EBV in stomach adenocarcinoma. In view of the above, it was argued that PD-L1 overexpression in these cases may have stemmed from the loss of 3'-UTR genome sequence. To validate this hypothesis, they utilized the clustered regulatory interspaced short palindromic repeats (CRISPR)-Cas9 system to introduce deletions and inversions in the PD-L1 3'-UTR sequence in several murine and human cell lines. As expected, these cells displayed increased PD-L1 levels. Additionally, these 3'-UTR truncated cells displayed delayed clearance of PD-L1 mRNA (after inhibiting de novo transcription with actinomycin D), suggesting that the PD-L1 3'-UTR may have a negative regulatory effect on the stability of mRNA. Further, it was observed that PD-L1 3'-UTR truncation had a stronger impact on PD-L1 overexpression than IFNγ, yet the combination of stimulating 3'-UTR-disrupted cells with IFNγ still resulted in a synergistic increase in PD-L1 expression.
The study assessed the outcomes of disrupting the PD-L1 3'-UTR sequence. Co-culturing PC-9 cells (human lung cancer cells with or without PD-L1 3'-UTR disruption) with Jurkat cells (PD-1 positive T cells) resulted in a significantly higher degree of T cell apoptosis when the cancer cells contained the 3'-UTR disruption. A tumor regression model (EGA-OVA cells with/without disrupted PD-L1 3'-UTR disruption inoculated in syngeneic C57BL/6 mice) was adopted to evaluate the effects of this structural variation on the anti-tumor immune response. Treatment with immunostimulatory RNA polyinosinic-polycytidylic acid [poly(I:C)] in mice with EGA-OVA cells with the disrupted PD-L1 3'-UTR sequence resulted in a weak CD8+ T cells response and, consequently, minimal tumor regression. On the other hand, mice with EGA-OVA cells bearing the intact PD-L1 3'-UTR sequence exhibited tumor regression with a high degree of CD8+ TILs when subjected to poly(I:C). These results demonstrate that increased activation of PD-1/PD-L1 pathway secondary to PD-L1 3'-UTR disruption can mediate immune escape of tumor cells from CD8+ cytotoxic T cells.
Discussion
Kataoka and colleagues have elaborated upon several significant hypotheses in this paper. Their study noted a direct association between PD-L1 overexpression and viral infections, wherein the latter was hypothesized to cause a loss of 3'-UTR sequence. Of note, the validation of this particular hypothesis was achieved using the CRISPR-Cas9 system. Additional studies are warranted in many other histologies where this novel mechanism may be relevant as well, especially in virally driven cancers. In this study, the authors have not shown a direct link between HTLV-1 infection and disruption of the 3'-UTR sequence in ATL. A study demonstrating whether or not HTLV-1 infection can cause PD-L1 3'-UTR sequence disruption in ATL can be considered.
The study also investigated the possibility of clonal selection of PD-L1 overexpressing tumor cells in presence of anti-tumor immunity. In vivo murine experiments demonstrated that disruption of the PD-L1 3'-UTR sequence induced immune escape from CD8+ cytotoxic T cells, thus highlighting the significance of certain structural variations in mediating clonal selection of tumor cells. Considering the above, it may be prudent to further investigate the disruption of the PD-L1 3'-UTR as a potential marker for tumors that exhibit greater clinical response to anti-PD-1/PD-L1 blocking therapies. Building upon our current knowledge, we believe that the observations made in this study will contribute towards realization of the full potential of PD-L1 targeted therapy agents in the treatment of cancer.
In summary, the study identified another novel mechanism for immune evasion by cancer cell via PD-L1 overexpression relevant in various cancer types. Furthermore, PD-L1 3'-UTR disruption that invariably leads to PD-L1 overexpression across different tumor types can be developed as a robust biomarker not only to identify tumors that utilize PD-L1 as a main mechanism for immune evasion but also to better predict outcomes to any T cell medicated immuno-oncology treatments including immune modulatory treatments and genetically engineered T cell therapy.
Acknowledgments
Funding: None.
Footnote
Provenance and Peer Review: This article was commissioned and reviewed by the Section Editor Lichao Sun [State Key Laboratory of Molecular Oncology, National Cancer Center (NCC)/ Cancer Hospital, Chinese Academy of Medical Sciences (CAMS), Peking Union Medical College, Beijing, China].
Conflicts of Interest: All authors have completed the ICMJE uniform disclosure form (available at http://dx.doi.org/10.21037/tcr.2016.08.29). The authors have no conflicts of interest to declare.
Ethical Statement: The authors are accountable for all aspects of the work in ensuring that questions related to the accuracy or integrity of any part of the work are appropriately investigated and resolved.
Open Access Statement: This is an Open Access article distributed in accordance with the Creative Commons Attribution-NonCommercial-NoDerivs 4.0 International License (CC BY-NC-ND 4.0), which permits the non-commercial replication and distribution of the article with the strict proviso that no changes or edits are made and the original work is properly cited (including links to both the formal publication through the relevant DOI and the license). See: https://creativecommons.org/licenses/by-nc-nd/4.0/.
References
- Rizvi NA, Chow LQ, Borghaei H, et al. Safety and response with nivolumab (anti-PD-1; BMS-936558, ONO-4538) plus erlotinib in patients (pts) with epidermal growth factor receptor mutant (EGFR MT) advanced NSCLC. J Clin Oncol 2014;32:abstr 8022.
- Postow MA, Chesney J, Pavlick AC, et al. Nivolumab and ipilimumab versus ipilimumab in untreated melanoma. N Engl J Med 2015;372:2006-17. [Crossref] [PubMed]
- Robert C, Schachter J, Long GV, et al. Pembrolizumab versus Ipilimumab in Advanced Melanoma. N Engl J Med 2015;372:2521-32. [Crossref] [PubMed]
- Borghaei H, Paz-Ares L, Horn L, et al. Nivolumab versus Docetaxel in Advanced Nonsquamous Non-Small-Cell Lung Cancer. N Engl J Med 2015;373:1627-39. [Crossref] [PubMed]
- Brahmer J, Reckamp KL, Baas P, et al. Nivolumab versus Docetaxel in Advanced Squamous-Cell Non-Small-Cell Lung Cancer. N Engl J Med 2015;373:123-35. [Crossref] [PubMed]
- Weber JS, D'Angelo SP, Minor D, et al. Nivolumab versus chemotherapy in patients with advanced melanoma who progressed after anti-CTLA-4 treatment (CheckMate 037): a randomised, controlled, open-label, phase 3 trial. Lancet Oncol 2015;16:375-84. [Crossref] [PubMed]
- Pardoll DM. The blockade of immune checkpoints in cancer immunotherapy. Nat Rev Cancer 2012;12:252-64. [Crossref] [PubMed]
- Ansell SM, Lesokhin AM, Borrello I, et al. PD-1 blockade with nivolumab in relapsed or refractory Hodgkin's lymphoma. N Engl J Med 2015;372:311-9. [Crossref] [PubMed]
- Green MR, Monti S, Rodig SJ, et al. Integrative analysis reveals selective 9p24.1 amplification, increased PD-1 ligand expression, and further induction via JAK2 in nodular sclerosing Hodgkin lymphoma and primary mediastinal large B-cell lymphoma. Blood 2010;116:3268-77. [Crossref] [PubMed]
- Wilke CM, Wei S, Wang L, et al. Dual biological effects of the cytokines interleukin-10 and interferon-γ. Cancer Immunol Immunother 2011;60:1529-41. [Crossref] [PubMed]
- Kim J, Myers AC, Chen L, et al. Constitutive and inducible expression of b7 family of ligands by human airway epithelial cells. Am J Respir Cell Mol Biol 2005;33:280-9. [Crossref] [PubMed]
- Kim JM, Chen DS. Immune escape to PD-L1/PD-1 blockade: seven steps to success (or failure). Ann Oncol 2016;27:1492-504. [Crossref] [PubMed]
- Ji M, Liu Y, Li Q, et al. PD-1/PD-L1 pathway in non-small-cell lung cancer and its relation with EGFR mutation. J Transl Med 2015;13:5. [Crossref] [PubMed]
- Hamanishi J, Mandai M, Matsumura N, et al. PD-1/PD-L1 blockade in cancer treatment: perspectives and issues. Int J Clin Oncol 2016;21:462-73. [Crossref] [PubMed]
- Iwai Y, Ishida M, Tanaka Y, et al. Involvement of PD-L1 on tumor cells in the escape from host immune system and tumor immunotherapy by PD-L1 blockade. Proc Natl Acad Sci U S A 2002;99:12293-7. [Crossref] [PubMed]
- Topalian SL, Hodi FS, Brahmer JR, et al. Safety, activity, and immune correlates of anti-PD-1 antibody in cancer. N Engl J Med 2012;366:2443-54. [Crossref] [PubMed]
- Brahmer JR, Tykodi SS, Chow LQ, et al. Safety and activity of anti-PD-L1 antibody in patients with advanced cancer. N Engl J Med 2012;366:2455-65. [Crossref] [PubMed]
- Taube JM, Anders RA, Young GD, et al. Colocalization of inflammatory response with B7-h1 expression in human melanocytic lesions supports an adaptive resistance mechanism of immune escape. Sci Transl Med 2012;4:127ra37 [Crossref] [PubMed]
- Taube JM, Anders RA, Xu H, et al. B7-H1 expression co-localizes with inflammatory infiltrates in benign and malignant melanocytic lesions: Implications for immunotherapy. Sci Transl Med 2012;4:127ra37-ra37.
- Steidl C, Shah SP, Woolcock BW, et al. MHC class II transactivator CIITA is a recurrent gene fusion partner in lymphoid cancers. Nature 2011;471:377-81. [Crossref] [PubMed]
- Green MR, Rodig S, Juszczynski P, et al. Constitutive AP-1 activity and EBV infection induce PD-L1 in Hodgkin lymphomas and posttransplant lymphoproliferative disorders: implications for targeted therapy. Clin Cancer Res 2012;18:1611-8. [Crossref] [PubMed]
- Kataoka K, Shiraishi Y, Takeda Y, et al. Aberrant PD-L1 expression through 3'-UTR disruption in multiple cancers. Nature 2016;534:402-6. [Crossref] [PubMed]
- Rimsza LM, Roberts RA, Miller TP, et al. Loss of MHC class II gene and protein expression in diffuse large B-cell lymphoma is related to decreased tumor immunosurveillance and poor patient survival regardless of other prognostic factors: a follow-up study from the Leukemia and Lymphoma Molecular Profiling Project. Blood 2004;103:4251-8. [Crossref] [PubMed]
- U.S. Food & Drug Administration. List of Cleared or Approved Companion Diagnostic Devices (In Vitro and Imaging Tools). Available online: http://www.fda.gov/MedicalDevices/ProductsandMedicalProcedures/InVitroDiagnostics/ucm301431.htm
- Rooney MS, Shukla SA, Wu CJ, et al. Molecular and genetic properties of tumors associated with local immune cytolytic activity. Cell 2015;160:48-61. [Crossref] [PubMed]