Alpha-fetoprotein-to-PIVKA-II ratio as a potential biomarker for hepatocellular carcinoma differentiation, imaging characteristics, and patient survival
Highlight box
Key findings
• Hepatocellular carcinoma (HCC) patients with a high (>0.175) alpha-fetoprotein-to-PIVKA-II ratio (APR) have a worse prognosis.
What is known and what is new?
• The World Health Organization (WHO) distinguishes 3 grades of differentiation of HCC in immunohistochemistry: well-differentiated (WD-HCC), moderately differentiated (MD-HCC) and poorly differentiated (PD-HCC).
• Patients with PD-HCC have a poorer prognosis.
• APR showed a high sensitivity (91%) and specificity (90%) in differentiating WD-HCC/MD-HCC from PD-HCC and may predict prognosis in patients with HCC.
What is the implication, and what should change now?
• APR can be considered as a biomarker for differentiation of HCC, imaging and prognosis prediction for HCC. It could be incorporated into clinical practice for diagnosis, management and prognosis prediction in patients with HCC.
Introduction
Hepatocellular carcinoma (HCC) is the sixth most common cancer in the world, accounting for over 90% of primary liver cancers, and is the second leading cause of cancer death in men and the sixth leading cause in women worldwide (1,2). HCC is the second most aggressive cancer after pancreatic cancer, with a poor prognosis and high mortality. Its lethality and poor prognosis are due to its late diagnosis, intrinsic heterogeneity, and limited treatment options in intermediate and advanced stages of the disease (3-8).
There is an ongoing interest in prognostic factors for cancer, such as immunohistochemical biomarkers, which can be used to stratify patients into different groups with different prognoses. The use of specific biomarkers in immunohistochemistry allows the distinction of three HCC subtypes: well-differentiated HCC (WD-HCC), moderately differentiated HCC (MD-HCC), and poorly differentiated HCC (PD-HCC) (9,10). The identification of better prognostic biomarkers remains an urgent priority, particularly serum biomarkers that do not require invasive sampling and that would help clinicians select more appropriate therapeutic strategies for patients in poor prognosis groups.
Protein induced by vitamin K absence or antagonist-II (PIVKA-II), also known as Des-γ-carboxy-prothrombin (DCP), is a non-functional variant of coagulation factor II (prothrombin) that is elevated in certain liver diseases, particularly HCC. As such, PIVKA-II is a useful biomarker for the diagnosis, monitoring and management of HCC (11-13). Alpha-fetoprotein (AFP) is another protein normally produced during fetal development, but elevated levels are also associated with liver disease, including HCC (14). PIVKA-II and AFP are independently produced by HCC tissues without any association with each other (15,16). Studies have reported a direct correlation between HCC size and prognosis and PIVKA-II levels. Higher PIVKA-II levels are associated with larger HCC size and poorer prognosis (17-21). Similarly, AFP levels in patients with HCC are proportional to the degree of tumor cell proliferation. Liu et al. (22) demonstrated that AFP promotes HCC growth and AFP-positive HCC patients have a higher cell proliferation rate than AFP-negative patients. However, no correlation between AFP level and prognosis was found by Tian et al. (21).
The combination of PIVKA-II and AFP increases the sensitivity and specificity for the detection and prognosis of HCC at an early stage (11,12,23-25). AFP and PIVKA-II as a ratio can combine the complementary information of the two biomarkers, improving the diagnostic and prognostic performance of both for HCC, thereby mitigating the limitations of each individual biomarker. Thus, alpha-fetoprotein-to-PIVKA-II ratio (APR) may serve as a new marker to predict the grade of differentiation and prognosis of HCC. Therefore, our study aims to demonstrate the prognostic significance of high APR for PD-HCC and overall survival (OS) in patients after intra-arterial therapies, and further to evaluate the predictive value of APR in predicting histologic grade and imaging patterns of HCC. This article is presented in accordance with the REMARK reporting checklist (available at https://tcr.amegroups.com/article/view/10.21037/tcr-24-958/rc).
Methods
Study population
This study was conducted at The First Affiliated Hospital of Kunming Medical University in China between October 2022 and June 2024, and was a single-center, prospective, fully randomized study. The inclusion criteria were: (I) patients with HCC diagnosed by clinical symptoms, imaging examinations, laboratory tests; (II) those who underwent liver biopsy for histopathology and immunohistochemistry for stratification of HCCs into subgroups according to the degree of tumor differentiation; (III) those who underwent intra-arterial therapies; (IV) and performed laboratory tests and medical imaging after treatment. The exclusion criteria were: (I) patients who did not undergo liver biopsy; (II) those who did not undergo intra-arterial therapies; (III) and those who did not undergo post-treatment evaluation including laboratory tests and medical imaging.
All data were collected from the hospital database. We recruited 59 patients who underwent liver biopsy for pathologic and immunohistochemical analysis of liver nodules. A total of 42 patients were enrolled after excluding those who did not meet the inclusion criteria. During enrollment, we assigned alphanumeric codes to each patient to identify them for randomization. The 42 patients were divided into three groups and then randomized. Randomization was stratified according to the degree of tumor differentiation by immunohistochemistry, with WD-HCC, MD-HCC, and PD-HCC groups.
Ethical issues and informed consent
The study was conducted in accordance with the Declaration of Helsinki (as revised in 2013) and was approved by the local research ethics committee of The First Affiliated Hospital of Kunming Medical University, Kunming, China (approval No. 2.0; Date: 23 December, 2023). Informed consent was obtained from each of the individuals who were enrolled in this study.
Data collection
We prospectively collected data on patient demographics, blood tests (such as blood counts, liver and kidney function tests) and medical imaging studies performed in the week prior to treatment, and immunohistochemistry results. PIVKA-II and AFP were measured by chemiluminescent immunoassay (Cobas E 601 Electrochemiluminescence). Samples were collected after an overnight fast of at least 8 hours. The cut-off values for PIVKA-II and AFP for HCC at The First Affiliated Hospital of Kunming Medical University were 40 mAU/mL and 400 ng/mL, respectively. Serum levels of both markers up to these reference values were considered positive. The APR is defined as the serum level of AFP divided by the serum level of PIVKA-II. The calculated APR was used to plot a receiver operating characteristic (ROC) curve for postoperative median survival time. The APR was 0.175 at the maximum of the Youden index. We used this value as the optimal cut-off point to divide patients into two groups, one with a high APR and the other with a low APR. The Youden index is a measure of the power of a dichotomous diagnostic test. It is defined as the sum of sensitivity and specificity of the test, minus one, and is often used in conjunction with ROC analysis. The index is defined for all points on an ROC curve, and the maximum value of the index can be used as a criterion for selecting the optimal cut-off point when a diagnostic test gives a numeric rather than a dichotomous result (26). The optimal cut-off point is therefore the one that achieves this maximum value, as it is the cut-off point that optimizes the biomarker’s ability to discriminate when sensitivity and specificity are given equal weight (27,28).
Imaging analysis
Thirty-five (83.3%) of the 42 patients underwent magnetic resonance imaging (MRI) alone or in combination with computed tomography (CT), while 16 (38.1%) patients underwent CT with or without MRI. All MRI examinations were performed on a clinical 1.5-T and 3-T whole-body system with an 8-channel body phased array coil. All CT scans were performed on 16- or 64-MDCT scanners. Both non-contrast and intravenous contrast images were used in all patients. The triple phase (arterial phase: 35–45 s; portal venous phase: 60–80 s; and delayed phase: 3–5 minutes) were used. Images were reviewed separately by three board-certified radiologists experienced in abdominal and liver imaging. A fourth radiologist collaborated with each of the three radiologists to ensure concordance in image analysis and confidence in interpretation. For each lesion, we evaluated the following characteristics, among others: tumor size and number, presence of vascular invasion, intra-tumor necrosis, rim enhancement in arterial phase, nodule-in-nodule appearance, mosaic architecture, and lesion shape, etc. Using the MRI enhancement patterns, we calculated the percentage signal ratio (PSR) in the arterial phase to evaluate the change in the vasculature between the two APR groups. We used the following formula: PSRA = 100× (ASA/LSA), as calculated by Kloeckner et al. (29), where ASA is the adjacent signal intensity (SI) corresponding to the average of the two areas adjacent to the lesion and LSA is the SI of the lesion on arterial (A) phase. In each patient, we evaluated the nodule that underwent pathological confirmation.
Pathologic analysis and histologic grade of tumors
Specimens were obtained by percutaneous biopsy performed by interventional radiologists assisted by interventional radiology residents using 14-G coaxial needles. Specimens were sent to pathology for analysis by pathologists blinded to the radiologic results. The pathology report included tumor tissue markers and histologic grade of HCC. HCC lesions were categorized into subgroups according to the degree of tumor differentiation based on immunohistochemistry according to the World Health Organization (WHO) Classification of Tumors, 5th edition [2019] (10), including WD-HCC, MD-HCC, and PD-HCC groups. The highest grade was reported when the tumor fell between two grades. WD-HCC was diagnosed when tumor cells resembled mature benign hepatocytes with minimal to mild atypia. MD-HCC was diagnosed when there was evidence of malignancy on hematoxylin and eosin (H&E) staining with morphology strongly suggesting hepatocellular differentiation. However, PD-HCC was diagnosed when there was evidence of malignancy on H&E staining while the morphology was consistent with a broad spectrum of poorly differentiated carcinomas (10).
Treatment methods
Patients in each group received intra-arterial therapies [drug-eluting bead transarterial chemoembolization (DEB-TACE) or conventional transarterial chemoembolization (c-TACE)] with or without hepatic arterial infusion chemotherapy (HAIC) based on mFOLFOX6-HAIC (oxaliplatin, calcium leucovorin, 5-fluorouracil). All patients received anti-programmed death-1 (anti-PD-1) and anti-programmed cell death ligand 1 (anti-PD-L1) intravenously once every 3 weeks, which was stopped for 3 days before and after TACE.
Follow-up
As soon as the patient was able to walk after the procedure, a non-contrast CT scan was performed to assess the distribution of lipiodol in the liver and its degree of distribution in the tumor (for patients receiving lipiodol). Patients who underwent DEB-TACE had an early CT scan or MRI with contrast injection to look for tumor enhancement after the procedure. Images were analyzed by experienced radiologists blinded to patient histology. The objective response rate (ORR) was assessed using the modified RECIST (mRECIST) classification (30). In the absence of contraindications, retreatment was scheduled 4–8 weeks after the initial treatment. Patients with a satisfactory response were followed by telephone until June 19, 2024 or death. Follow-up lasted up to 596 days, and the median follow-up was 209.0 (range, 45–596) days.
Statistical analyses
Continuous variables were non-normal and expressed as median [interquartile range (IQR)]. Multi-numeric variables were compared using the Kruskal-Wallis test, and the non-parametric Mann-Whitney test was used to compare differences between two numeric variables. Categorical variables were expressed as numbers (%), and differences were compared using the χ2 test or Fisher’s exact test. Spearman’s rank test was used to detect correlations between variables.
ROC curves were constructed and areas under the curve (AUCs) were calculated to evaluate the predictive ability of APR to discriminate subgroup(s) of HCC with good or poor prognosis. The cut-off point of APR (0.175) was selected to provide optimal sensitivity and specificity. Kaplan-Meier survival analysis was performed to evaluate the effect of tumor differentiation and change in APR on overall patient survival. Kaplan-Meier curves were used to illustrate the cumulative incidence of various outcomes and Cox regression model was performed to identify prognostic factors based on the variables selected by univariate analysis. Data processing and analysis were performed using IBM SPSS Statistics version 27.0 (IBM, Chicago, IL, USA). A P<0.05 was considered statistically significant with a confidence interval (CI) of 95%. The endpoint of this study concerned the OS rate after a follow-up of 596 days.
Results
Baseline characteristics
A total of 42 patients with HCC who underwent liver biopsy for histologic analysis were enrolled in this study. Among them, 12 (28.6%), 19 (45.2%), and 11 (26.2%) had WD-HCC, MD-HCC, and PD-HCC, respectively (P=0.41). The median age of the patients was 54 (range, 37–82) years, and 38 (90.5%) patients were male. Only WD-HCC group presented cases (2/12, or 16.7%) with PIVKA-II level ≤40 mAU/mL, while none of the patients in MD-HCC and PD-HCC groups presented low PIVKA-II level (P=0.07). The rate of patients with high serum AFP levels (>400 ng/mL) increased with the degree of tumor differentiation: 4/12 (or 33.3%), 7/19 (or 36.8%), and 9/11 (or 81.8%) of patients in the WD-HCC, MD-HCC, and PD-HCC groups, respectively (P=0.03). However, patients in the MD-HCC group (9 of 16 patients with usable data, or 56.3%) had more cases of alanine aminotransferase (ALAT) >50 IU/mL (P=0.02). Table 1 compares the baseline characteristics of patients in the WD-HCC, MD-HCC, and PD-HCC groups.
Table 1
Characteristics | Total (n=42) | WD-HCC (n=12) | MD-HCC (n=19) | PD-HCC (n=11) | F/χ2 | P |
---|---|---|---|---|---|---|
Gender, male/female | 38/4 | 11/1 | 17/2 | 10/1 | 0.044 | 0.97 |
Age, ≤54/>54 years | 22/20 | 6/6 | 8/11 | 8/3 | 2.657 | 0.26 |
Etiology, HBV/HCV/others | 33/5/4 | 9/2/1 | 14/2/3 | 10/1/0 | 2.471 | 0.65 |
BCLC stage, A/B/C/D | 2/21/17/2 | 1/9/2/0 | 1/9/7/2 | 0/3/8/0 | 10.508 | 0.10 |
Child-Pugh class, A/B/C | 24/16/2 | 8/4/0 | 11/6/2 | 5/6/0 | 3.941 | 0.41 |
PIVKA-II, ≤40/>40 mAU/mL | 2/40 | 2/10 | 0/19 | 0/11 | 5.250 | 0.07 |
AFP, ≤400/>400 ng/mL | 22/20 | 8/4 | 12/7 | 2/9 | 7.024 | 0.03 |
CA19-9, ≤43/>43 U/mL | 32/4 | 11/1 | 13/2 | 8/1 | 0.169 | 0.91 |
ALAT, ≤50/>50 IU/mL | 25/12 | 11/1 | 7/9 | 7/2 | 7.750 | 0.02 |
ASAT, ≤40/>40 IU/mL | 11/26 | 5/7 | 4/12 | 2/7 | 1.233 | 0.52 |
Albumin, ≤40/>40 g/L | 11/26 | 5/7 | 4/12 | 2/7 | 1.233 | 0.54 |
TBil, ≤23/>23 µmol/L | 26/11 | 9/3 | 11/5 | 6/3 | 0.202 | 0.90 |
Intra-arterial therapies | ||||||
DEB-TACE, yes/no | 32/10 | 11/1 | 12/7 | 9/2 | 3.555 | 0.16 |
HAIC, yes/no | 19/23 | 4/8 | 9/10 | 6/5 | 1.106 | 0.57 |
c-TACE, yes/no | 4/38 | 1/11 | 2/17 | 1/10 | 0.044 | 0.97 |
Anti-PD-1 ± anti-PD-L1, yes/no | 24/18 | 5/7 | 10/9 | 9/2 | 4.066 | 0.13 |
Note: CA19-9, ALAT, ASAT, albumin and TBil were not available in some patients. This explains why the sum of these items was affected. Data are presented as numbers. HCC, hepatocellular carcinoma; WD-HCC, well-differentiated HCC; MD-HCC, moderately differentiated HCC; PD-HCC, poorly differentiated HCC; HBV, hepatitis B virus; HCV, hepatitis C virus; BCLC, Barcelona Clinic Liver Cancer; PIVKA-II, protein induced by vitamin K absence or antagonist-II; AFP, alpha-fetoprotein; CA19-9, carbohydrate antigen 19-9; ALAT, alanine aminotransferase; ASAT, aspartate aminotransferase; TBil, total bilirubin; DEB-TACE, drug-eluting bead transarterial chemoembolization; HAIC, hepatic arterial infusion chemotherapy; c-TACE, conventional TACE; PD-1, programmed cell death protein-1; PD-L1, programmed cell death ligand 1.
Change in PIVKA-II and AFP and HCC differentiation
The median levels of PIVKA-II and AFP were 3,300.56 (range, 15.07–30,000) mAU/mL and 368.13 (range, 1.89–72,000) ng/mL, respectively. Within groups, the median serum levels of PIVKA-II increased non-significantly with increasing tumor grade, with 412.06 (range, 15.07–30,000), 3,523.79 (range, 273.98–30,000), and 7,493.13 (range, 44.12–30,000) mAU/mL in the WD-HCC, MD-HCC, and PD-HCC subgroups, respectively (Z=0.477 each, all P=0.50). Similarly, median serum AFP levels increased proportionally with tumor grade, with 8.29 (range, 1.89–2,000), 46.34 (range, 4.04–2,000), and 2,000 (range, 101.73–72,000) ng/mL in the WD-HCC, MD-HCC, and PD-HCC subgroups, respectively. The difference was significant between WD-HCC and PD-HCC groups and between MD-HCC and PD-HCC groups (Z=5.239 and 3.589; P<0.001 and P=0.008, respectively), but the difference was not significant between WD-HCC and MD-HCC groups (Z=0.595, P=0.44). The change in serum PIVKA-II and AFP levels according to the degree of tumor differentiation is summarized in Figure 1.
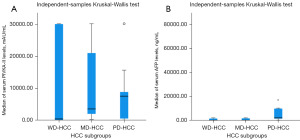
ROC analysis
ROC analysis was used to estimate whether APR is reliable in differentiating PD-HCC from WD-HCC/MD-HCC. We calculated the AUC, cut-off values, sensitivity and specificity of APR as shown in Table 2. The ROC curve for this APR value is shown in Figure 2.
Table 2
Characteristic | AUC (95% CI) | Cut-off value | Sensitivity (%) | Specificity (%) | P value |
---|---|---|---|---|---|
APR | 0.974 (0.933–1.000) | 0.175 | 91 | 90 | <0.001 |
P<0.001 for comparison within HCC subtypes. PD-HCC, poorly differentiated HCC; WD-HCC, well-differentiated HCC; MD-HCC, moderately differentiated HCC; HCC, hepatocellular carcinoma; APR, alpha-fetoprotein-to-PIVKA-II ratio; PIVKA-II, protein induced by vitamin K absence or antagonist-II; AUC, area under the curve; CI, confidence interval.
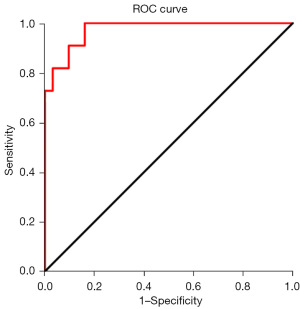
Correlation between APR and clinical characteristics
We found that none of the patients in the WD-HCC group had a high APR value, whereas most of the patients (90.9%) in the PD-HCC group had a high APR value (P<0.001) (Figure 3). We also found a significant linear correlation between APR and the degree of HCC differentiation, with a correlation coefficient of 0.773 (P<0.001). No significant difference was found between patients with low (≤0.175) and high (>0.175) APR values in terms of gender, underlying etiology, Barcelona Clinic Liver Cancer (BCLC) stage, and Child-Pugh class. The clinical characteristics of the two groups are compared in Table 3.
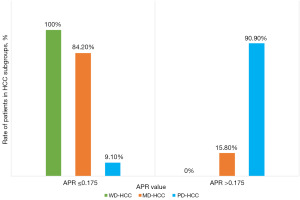
Table 3
Characteristics | Total (n=42) | APR ≤0.175 (n=29) | APR >0.175 (n=13) | F/χ2 | P value |
---|---|---|---|---|---|
Gender, male/female | 38/4 | 27/2 | 11/2 | 0.751 | 0.38 |
Age, ≤54/>54 years | 22/20 | 14/15 | 8/5 | 0.633 | 0.42 |
Etiology, HBV/HCV/others | 33/5/4 | 22/4/3 | 11/1/1 | 0.434 | 0.80 |
BCLC stage, A/B/C/D | 2/21/17/2 | 2/17/8/2 | 0/4/9/0 | 7.032 | 0.07 |
Child-Pugh class, A/B/C | 24/16/2 | 17/10/2 | 7/6/0 | 1.253 | 0.53 |
Data are presented as numbers. HCC, hepatocellular carcinoma; APR, alpha-fetoprotein-to-PIVKA-II ratio; PIVKA-II, protein induced by vitamin K absence or antagonist-II; HBV, hepatitis B virus; HCV, hepatitis C virus; BCLC, Barcelona Clinic Liver Cancer.
Association between imaging features and APR levels
Median HCC nodule size on MRI and CT was 5.8 (range, 2.0–20.0) and 6.7 (range, 2.7–22.0) cm, respectively, and overall median size was 6.6 (range, 2.0–22.0) cm. Overall and on MRI, median tumor size tended to be smaller in patients with low APR [5.5 (2.0–22.0) and 5.4 (range, 2.0–15.5) cm, respectively] than in those with high APR [7.0 (2.9–20.0) cm], but the difference was not significant (Z=0.735 and 0.693, respectively; P>0.05). However, on CT, patients with low APR tended to have slightly larger lesions [7.2 (range, 2.9–22.0) cm] than those with high APR [6.2 (range, 2.7–17.0) cm] (Z=−0.453, P=0.65). Most patients in high APR group had more than one HCC lesion (MRI, P=0.02; CT, P=0.07). We found more cases of tumor vascular invasion in patients with high APR than in those with low APR on MRI (P=0.03), while no difference was found on CT (P=0.71). PSR was calculated and the median value was 89.9% (IQR, 74.2–113.3%). This value allowed as to classify patients with high or low PSR, and we found that patients (62.5%) with low APR have a low PSR (≤89.9) in contrast to the patients with high APR value (P=0.053) (Table 4, Figure 4).
Table 4
Characteristics | Total (n=42) | APR ≤0.175 (n=29) | APR >0.175 (n=13) | P value |
---|---|---|---|---|
Tumor size (cm) | ||||
Overall | 6.6 (2.0–22.0) | 5.5 (2.0–22.0) | 7.0 (2.9–20.0) | 0.46 |
MRI | 5.8 (2.0–20.0) | 5.4 (2.0–15.5) | 7.0 (2.9–20.0) | 0.63 |
CT | 6.7 (2.7–22.0) | 7.2 (2.9–22.0) | 6.2 (2.7–17.0) | 0.59 |
Tumor number | ||||
MRI, 1/2/≥3 | 17/7/11 (48.6/20.0/31.4) | 14/6/4 (58.3/25.0/16.7) | 3/1/7 (27.3/9.1/63.6) | 0.02 |
CT, 1/2/≥3 | 10/0/6 (62.5/0.0/37.5) | 9/0/3 (75.0/0.0/25.0) | 1/0/3 (25.0/0.0/75.0) | 0.07 |
Vascular invasion | ||||
MRI, yes/no | 16/19 (45.7/54.3) | 8/16 (33.3/66.7) | 8/3 (72.7/27.3) | 0.03 |
CT, yes/no | 8/8 (50.0/50.0) | 6/6 (50.0/50.0) | 2/2 (50.0/50.0) | 0.71 |
Intratumoral necrosis | ||||
MRI, yes/no | 28/7 (80.0/20.0) | 18/6 (75.0/25.0) | 10/1 (90.9/9.1) | 0.27 |
CT, yes/no | 16/0 (100/0.0) | 12/0 (100/0.0) | 4/0 (100/0.0) | 0.33 |
Shape | ||||
MRI (Ig/Lb/Rg) | 27/5/3 (77.1/14.3/8.6) | 19/5/0 (79.2/20.8/0.0) | 8/0/3 (72.7/0.0/27.3) | 0.01 |
CT (Ig/Lb/Rg) | 11/3/2 (68.8/18.8/12.5) | 9/2/0 (81.8/18.2/0.0) | 2/1/2 (40.0/20.0/40.0) | 0.07 |
Rim in the arterial phase | ||||
MRI, yes/no | 20/15 (57.1/42.9) | 16/8 (66.7/33.3) | 4/7 (36.4/63.6) | 0.09 |
CT, yes/no | 8/8 (50.0/50.0) | 4/7 (36.4/63.6) | 4/1 (80.0/20.0) | 0.10 |
Nod. in nod./MRI, yes/no | 5/30 (14.3/85.7) | 3/21 (12.5/87.5) | 2/9 (18.2/81.8) | 0.65 |
Mosaic Ar./MRI, yes/no | 15/20 (42.9/57.1) | 10/14 (41.7/58.3) | 5/6 (45.5/54.5) | 0.83 |
PSR (≤89.9/>89.9) | 18/17 (51.4/48.6) | 15/9 (62.5/37.5) | 3/8 (27.3/72.7) | 0.053 |
Continuous variables (tumor size) were expressed as median (interquartile range) while the remaining variables (categorical variables) were expressed as numbers (%). Note: 35 of the 42 patients underwent MRI alone or in combination with CT, and only 16 of the 42 patients underwent CT alone or in combination with MRI, therefore the sum of variables such as tumor number, vascular invasion, intra-tumoral necrosis, nodule-in-nodule, mosaic aspect, and PSR depended on the number of patients who underwent MRI and/or CT. APR, alpha-fetoprotein-to-PIVKA-II ratio; PIVKA-II, protein induced by vitamin K absence or antagonist-II; MRI, magnetic resonance imaging; CT, computed tomography; Ig, irregular; Lb, lobular; Rg, regular; nod., nodule; Ar., architecture; PSR, percentage signal ratio.
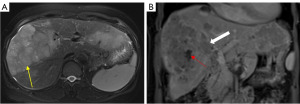
Survival analysis
After intra-arterial therapy, patients were followed for up to 596 days. During follow-up, 18 patients (42.86%) died, and the median OS was 379.8 (95% CI: 306.3–453.3) days. We found that 25.0% (3/12), 42.1% (8/19), and 63.6% (7/11) of patients in the WD-HCC, MD-HCC, and PD-HCC groups, respectively, died (P=0.09), and the OS time was slightly longer in the WD-HCC group at 459.1 (95% CI: 332.8–585.5) days, followed by the MD-HCC and PD-HCC groups with 375.3 (95% CI: 280.7–469.9) and 276.5 (95% CI: 136.3–416.8) days, respectively. The difference from the hypothesis test results was not significant (log-rank P=0.09) (Figure 5A).
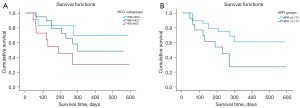
Interestingly, we found that the mortality rate was significantly higher in the group of patients with a high (>0.175) APR (9/13; 69.2%) than in the group of patients with a low (≤0.175) APR (9/29; 31.0%), so that the group of patients with a low APR has better survival results [429.2 (95% CI: 343.3–515.1) days] than the group of patients with a high APR [277.1 (95% CI: 161.4–392.5) days], with a significant difference from the hypothesis test results (log-rank P=0.03) (Figure 5B).
Factors affecting OS time
First, univariate Cox survival analysis was performed, and the results showed that HCC stage, Child-Pugh score, number of tumors, APR, lesion rim arterial phase hyperenhancement on MRI and lesion shape on CT were prognostic factors for OS. Second, multivariate analysis was performed and showed that Child-Pugh score and number of tumors were independent risk factors for OS (P<0.05, Table 5). HCC stage and tumor shape on CT were converted into two binary categories (WD-HCC and MD-HCC stages grouped together, and PD-HCC as a separate group; regular and lobular shape grouped together, while irregular shape remained a separate group).
Table 5
Variables | Univariate | Multivariate | |||||
---|---|---|---|---|---|---|---|
HR | 95% CI | P value | HR | 95% CI | P value | ||
HCC stage | – | – | 0.046* | – | – | – | |
WD-HCC/MD-HCC | 0.267 | 0.069–1.039 | 0.05* | – | – | – | |
PD-HCC | 0.457 | 0.165–1.266 | 0.13 | – | – | – | |
Child-Pugh score, B vs. A | 2.402 | 1.19–4.86 | 0.01* | 2.395 | 1.10–5.20 | 0.02* | |
No. of tumors, multiple vs. ≤2 | 0.257 | 0.101–0.656 | 0.004* | 2.650 | 0.97–7.26 | 0.05* | |
APR, high vs. low | 2.658 | 1.05–6.710 | 0.03* | – | – | – | |
Rim APHE, yes vs. no | 0.285 | 0.09–0.86 | 0.02* | – | – | – | |
Tumor shape (CT) | – | – | 0.05* | – | – | – | |
Regular/lobular | 0.048 | 0.004–0.594 | 0.01* | – | – | – | |
Irregular | 0.073 | 0.004–1.356 | 0.07 | – | – | – |
–: not applicable. *, P≤0.05. HR, hazard ratio; CI, confidence interval; HCC, hepatocellular carcinoma; WD, well-differentiated; MD, moderately differentiated; PD, poorly differentiated; APR, alpha-fetoprotein-to-PIVKA-II ratio; PIVKA-II, protein induced by vitamin K absence or antagonist-II; APHE, arterial phase hyperenhancement; CT, computed tomography.
Discussion
To the best of our knowledge, no studies have been performed on the APR. AFP is a glycoprotein produced by the liver and is considered a tumor marker for HCC, while PIVKA-II is another marker that may reflect the severity of the disease. Elevated AFP levels are often associated with the presence of HCC, and different thresholds are used to define a positive or negative test. Villalba-López et al. [2023] (31) reported that serum PIVKA-II levels may play an important role in predicting disease severity, and monitoring PIVKA-II levels in liver transplant patients may reflect early tumor recurrence. AFP and PIVKA-II may reflect different biological aspects of HCC.
AFP is often associated with active cell proliferation (21,22), whereas PIVKA-II is associated with the absence of vitamin K or the presence of vitamin K antagonists, which may indicate abnormal coagulation and liver function, as well as poorer prognosis and more aggressive tumors (32). Therefore, we believe that using these two markers in combination would provide a better diagnostic assessment of HCC than using them individually and would allow a more accurate prediction of the degree of tumor differentiation and the prognosis of patients after therapy. It is important to note that research is constantly evolving and new combinations of biomarkers may be explored in the future to further improve the management of HCC.
In this study, ROC curve analysis demonstrated that APR could discriminate PD-HCC from WD-HCC/MD-HCC. The cut-off value of APR used in the diagnostic setting was 0.175. The value of APR was mainly higher (>0.175) in patients with PD-HCC, while none of the patients with WD-HCC had an APR value higher than the cut-off value (0.175) (Figure 3). The mechanism underlying the correlation between high APR value and PD-HCC should be clarified. We suggest that the metabolic profile of tumor cells might be determined by the tumor cell deterioration from WD-HCC to PD-HCC. Our results showed that the median serum levels of both AFP and PIVKA-II increased from the first stage of differentiation (WD-HCC) to the last stage (PD-HCC). However, the median serum level of AFP was significantly higher in the PD-HCC group than in the two first stages of differentiation, in contrast to the median serum level of PIVKA-II, which did not show a significant difference between groups (Figure 1A,1B). This change may explain why all patients with WD-HCC in this study had an APR below the cut-off, while almost all patients (90.9%) with PD-HCC had an APR above the cut-off. We suggest that PIVKA-II production is inhibited when the tumor is poorly differentiated, while AFP production continues to increase as the tumor cells deteriorate from grade I to grade III. These findings are consistent with Si et al. [2020] who reported a positive correlation between both serum levels and tumor differentiation (33). Feng et al. [2021] (34) also reported a correlation between the degree of HCC differentiation and serum PIVKA-II levels, with a decrease in tumor cell differentiation being associated with an increase in serum PIVKA-II levels.
Several studies in the past have demonstrated a correlation between enhancement pattern and histologic differentiation of HCC on various medical imaging modalities (35-37). Studies have linked this to the various hemodynamic changes that occur during the multistep process of hepatocarcinogenesis (38-40), such as the replacement of normal vascularization (portal and hepatic arteries) by tumor neo angiogenesis in the early phase of HCC as an example of atypical enhancement in WD-HCC (40). In the present study, we observed a significant correlation between the PSR value and that of the APR. The majority of patients with low APR (62.5%) had a low PSR, whereas the majority of patients with high APR (72.7%) had a high PSR (P=0.053) (Table 4). Our results therefore suggest a correlation between the degree of tumor vascularization and the profile of tumor biomarkers (especially APR) in the arterial phase. We believe that these changes in the intra-tumor vasculature during differentiation of HCC nodules would, to some extent, influence the production and serum levels of tumor markers such as PIVKA-II and AFP.
Studies have reported hypoxia in the central zones of several types of solid tumors during tumor growth due to changes in vascularization that result in poor access to oxygen-supplying blood vessels (41,42). Suzuki et al. in 2011 (43) showed that in the face of severe hypoxia, nutrient deprivation and hypoxia-inducible factor (HIF)-1α transfection, hypo phosphorylation of the mammalian target of rapamycin (mTOR) pathway and effector proteins occurs, corresponding to the additional phenotypic change of HCC cells acquiring mesenchymal properties. This leads to an alteration in the synthesis of liver-specific proteins such as DCP, called PIVKA-II. Thus, Suzuki et al. concluded that PIVKA-II is not only a tumor marker of HCC, but also a marker of the phenotypic state of HCC, which would help to understand the ecology of HCC progression. In addition, intra-tumor hypoxia induces the expression of growth factors such as transforming growth factor-β (TGF-β) and platelet-derived growth factor (PDGF) and allows cell proliferation via HIF-1α (41). While PIVKA-II is inhibited by hypoxia, studies suggest that AFP plays a role in tumor growth by suppressing the anti-tumor immune response, for example, by inhibiting apoptotic signaling mediated by caspase-3, leading to drug resistance and metastasis (44-46). These studies clarify the underlying mechanism that justifies the correlation between high APR and PD-HCC and explain why PIVKA-II levels decrease in advanced HCC while AFP levels tend to increase.
In the present study, the sensitivity (91%) and specificity (90%) of APR in discriminating PD-HCCs from WD-HCCs/MD-HCCs suggest that APR could be used as a potential marker for HCC staging after medical imaging diagnosis, and it can predict the prognosis in patients with HCC after intra-arterial therapies, sparing patients the need for invasive procedures such as liver biopsy. We have shown that the mortality rate was high in patients with high APR, and therefore patients with PD-HCC have shorter survival. In other words, patients with high APR have a poor prognosis. Our results are consistent with several studies that have reported the prognosis of HCC according to the degree of differentiation, with PD-HCCs showing a poor prognosis compared with other subtypes (47,48).
In this study, Child-Pugh score and number of tumors on imaging were found to be prognostic factors for OS on multivariate analysis (Table 5). This suggests that these factors are associated with the degree of tumor cell deterioration, and AFP and PIVKA-II would discriminate the different underlying mechanisms that explain the role of these factors in the prognosis of patients. We demonstrated that patients in the high APR group were characterized by vascular invasion and were more likely to have more than one HCC lesion, and tumor size tended to be larger in this group. Studies have reported an association between HCC and markers of poor prognosis, such as tumor size, number of tumors, and vascular invasion (49-53). This supports our conclusion that patients with a high APR have a poor prognosis. We showed that tumor size tended to be smaller in patients with low APR than in those with high APR. We also showed that most patients in the high APR group tended to have more than one HCC lesion, in contrast to patients with low APR who tended to have a single lesion (Table 4). In addition, we found that patients with high APR had more cases of tumor vascular invasion than patients with low APR (Table 4, Figure 4). This supports our conclusion that patients with a high APR have a poor prognosis, and suggests that APR can be considered as a predictive marker of lesion size and number on CT and MRI.
Hwang et al. (54) reported an association between elevated levels of the biomarkers PIVKA-II and AFP and vascular invasion. Since vascular invasion is both a diagnostic marker of HCC, a predictive value for tumor differentiation and a prognostic marker (55), we extrapolate to say that the APR could be used as a predictive value for tumor differentiation and as a diagnostic and prognostic marker for HCC.
There are several limitations in this study. First, there is a lack of research on APR. Second, it was a single-center study. Third, the sample size was relatively small. The APR may be influenced by multiple factors. Large-scale and multicenter studies may be useful to further understand and validate our findings and to incorporate APR into clinical practice for diagnosis, management, and prognosis prediction.
Conclusions
This study showed that PD-HCC has a worse prognosis than WD-HCC and MD-HCC, and APR could be a significant noninvasive biomarker for predicting the degree of tumor differentiation and prognosis of patients. In addition, APR could predict the imaging characteristics of HCC. Validation of the present results in a larger randomized and prospective study is needed to confirm their usefulness.
Acknowledgments
This work was completed with the help of Drs. Cheng Wan, Haoqin Zhao, Ruchun Li and Chen Yue (all from The First Affiliated Hospital of Kunming Medical University, China).
Funding: None.
Footnote
Reporting Checklist: The authors have completed the REMARK reporting checklist. Available at https://tcr.amegroups.com/article/view/10.21037/tcr-24-958/rc
Data Sharing Statement: Available at https://tcr.amegroups.com/article/view/10.21037/tcr-24-958/dss
Peer Review File: Available at https://tcr.amegroups.com/article/view/10.21037/tcr-24-958/prf
Conflicts of Interest: All authors have completed the ICMJE uniform disclosure form (available at https://tcr.amegroups.com/article/view/10.21037/tcr-24-958/coif). The authors have no conflicts of interest to declare.
Ethical Statement: The authors are accountable for all aspects of the work in ensuring that questions related to the accuracy or integrity of any part of the work are appropriately investigated and resolved. The study was conducted in accordance with the Declaration of Helsinki (as revised in 2013) and was approved by the local research ethics committee of the First Affiliated Hospital of Kunming Medical University, Kunming, China (approval No. 2.0; Date: 23 December, 2023). Informed consent was obtained from each of the individuals who were enrolled in this study.
Open Access Statement: This is an Open Access article distributed in accordance with the Creative Commons Attribution-NonCommercial-NoDerivs 4.0 International License (CC BY-NC-ND 4.0), which permits the non-commercial replication and distribution of the article with the strict proviso that no changes or edits are made and the original work is properly cited (including links to both the formal publication through the relevant DOI and the license). See: https://creativecommons.org/licenses/by-nc-nd/4.0/.
References
- Global Burden of Disease Liver Cancer Collaboration. The Burden of Primary Liver Cancer and Underlying Etiologies From 1990 to 2015 at the Global, Regional, and National Level: Results From the Global Burden of Disease Study 2015. JAMA Oncol 2017;3:1683-91. [Crossref] [PubMed]
- The International Agency for Research on Cancer (IARC). (n.d.). Global Cancer Observatory. [retrieved August 15, 2024]. Available online: https://gco.iarc.fr/today
- Yang JD, Hainaut P, Gores GJ, et al. A global view of hepatocellular carcinoma: trends, risk, prevention and management. Nat Rev Gastroenterol Hepatol 2019;16:589-604. [Crossref] [PubMed]
- Guo C, Tang Y, Yang Z, et al. Hallmark-guided subtypes of hepatocellular carcinoma for the identification of immune-related gene classifiers in the prediction of prognosis, treatment efficacy, and drug candidates. Front Immunol 2022;13:958161. [Crossref] [PubMed]
- Zhang Q, Lou Y, Yang J, et al. Integrated multiomic analysis reveals comprehensive tumour heterogeneity and novel immunophenotypic classification in hepatocellular carcinomas. Gut 2019;68:2019-31. [Crossref] [PubMed]
- Ho DW, Tsui YM, Sze KM, et al. Single-cell transcriptomics reveals the landscape of intra-tumoral heterogeneity and stemness-related subpopulations in liver cancer. Cancer Lett 2019;459:176-85. [Crossref] [PubMed]
- Li L, Wang H. Heterogeneity of liver cancer and personalized therapy. Cancer Lett 2016;379:191-7. [Crossref] [PubMed]
- Farmer DG, Rosove MH, Shaked A, et al. Current treatment modalities for hepatocellular carcinoma. Ann Surg 1994;219:236-47. [Crossref] [PubMed]
- Nguyen T, Phillips D, Jain D, et al. Comparison of 5 Immunohistochemical Markers of Hepatocellular Differentiation for the Diagnosis of Hepatocellular Carcinoma. Arch Pathol Lab Med 2015;139:1028-34. [Crossref] [PubMed]
- International Agency for Research on Cancer (IARC), World Health Organization, International Academy of Pathology. Digestive System Tumours. WHO Classification of Tumours. Volume 1. 1 edition. IARC; 2019.
- Viggiani V, Palombi S, Gennarini G, et al. Protein induced by vitamin K absence or antagonist-II (PIVKA-II) specifically increased in Italian hepatocellular carcinoma patients. Scand J Gastroenterol 2016;51:1257-62. [Crossref] [PubMed]
- Wang X, Zhang W, Liu Y, et al. Diagnostic value of prothrombin induced by the absence of vitamin K or antagonist-II (PIVKA-II) for early stage HBV related hepatocellular carcinoma. Infect Agent Cancer 2017;12:47. [Crossref] [PubMed]
- Yu R, Tan Z, Xiang X, et al. Effectiveness of PIVKA-II in the detection of hepatocellular carcinoma based on real-world clinical data. BMC Cancer 2017;17:608. [Crossref] [PubMed]
- Bruix J, Sherman MAmerican Association for the Study of Liver Diseases. Management of hepatocellular carcinoma: an update. Hepatology 2011;53:1020-2. [Crossref] [PubMed]
- Meguro M, Mizuguchi T, Nishidate T, et al. Prognostic roles of preoperative α-fetoprotein and des-γ-carboxy prothrombin in hepatocellular carcinoma patients. World J Gastroenterol 2015;21:4933-45. [Crossref] [PubMed]
- Parfitt JR, Marotta P, Alghamdi M, et al. Recurrent hepatocellular carcinoma after transplantation: use of a pathological score on explanted livers to predict recurrence. Liver Transpl 2007;13:543-51. [Crossref] [PubMed]
- Cui SX, Zhang YS, Chu JH, et al. Des-gamma-carboxy prothrombin (DCP) antagonizes the effects of gefitinib on human hepatocellular carcinoma cells. Cell Physiol Biochem 2015;35:201-12. [Crossref] [PubMed]
- Ma XL, Zhu J, Wu J, et al. Significance of PIVKA-II levels for predicting microvascular invasion and tumor cell proliferation in Chinese patients with hepatitis B virus-associated hepatocellular carcinoma. Oncol Lett 2018;15:8396-404. [Crossref] [PubMed]
- Shirabe K, Toshima T, Kimura K, et al. New scoring system for prediction of microvascular invasion in patients with hepatocellular carcinoma. Liver Int 2014;34:937-41. [Crossref] [PubMed]
- Tarao K, Nozaki A, Komatsu H, et al. Real impact of tumor marker AFP and PIVKA-II in detecting very small hepatocellular carcinoma (≤ 2 cm, Barcelona stage 0) - assessment with large number of cases. World J Hepatol 2020;12:1046-54. [Crossref] [PubMed]
- Tian S, Chen Y, Zhang Y, et al. Clinical value of serum AFP and PIVKA-II for diagnosis, treatment and prognosis of hepatocellular carcinoma. J Clin Lab Anal 2023;37:e24823. [Crossref] [PubMed]
- Liu G, Ouyang Q, Xia F, et al. Alpha-fetoprotein response following transarterial chemoembolization indicates improved survival for intermediate-stage hepatocellular carcinoma. HPB (Oxford) 2019;21:107-13. [Crossref] [PubMed]
- Caviglia GP, Ribaldone DG, Abate ML, et al. Performance of protein induced by vitamin K absence or antagonist-II assessed by chemiluminescence enzyme immunoassay for hepatocellular carcinoma detection: a meta-analysis. Scand J Gastroenterol 2018;53:734-40. [Crossref] [PubMed]
- Seo SI, Kim HS, Kim WJ, et al. Diagnostic value of PIVKA-II and alpha-fetoprotein in hepatitis B virus-associated hepatocellular carcinoma. World J Gastroenterol 2015;21:3928-35. [Crossref] [PubMed]
- Wu J, Xiang Z, Bai L, et al. Diagnostic value of serum PIVKA-II levels for BCLC early hepatocellular carcinoma and correlation with HBV DNA. Cancer Biomark 2018;23:235-42. [Crossref] [PubMed]
- Ruopp MD, Perkins NJ, Whitcomb BW, et al. Youden Index and optimal cut-point estimated from observations affected by a lower limit of detection. Biom J 2008;50:419-30. [Crossref] [PubMed]
- Reiser B. Measuring the effectiveness of diagnostic markers in the presence of measurement error through the use of ROC curves. Stat Med 2000;19:2115-29. [Crossref] [PubMed]
- Youden WJ. Index for rating diagnostic tests. Cancer 1950;3:32-5. [Crossref] [PubMed]
- Kloeckner R, Pinto Dos Santos D, Kreitner KF, et al. Quantitative assessment of washout in hepatocellular carcinoma using MRI. BMC Cancer 2016;16:758. [Crossref] [PubMed]
- Lencioni R, Llovet JM. Modified RECIST (mRECIST) assessment for hepatocellular carcinoma. Semin Liver Dis 2010;30:52-60. [Crossref] [PubMed]
- Villalba-López F, Sáenz-Mateos LF, Sánchez-Lorencio MI, et al. Usefulness of PIVKA-II for monitoring after liver transplantation in patients with hepatocellular carcinoma. Sci Rep 2023;13:5621. [Crossref] [PubMed]
- Park MS, Lee KW, Kim H, et al. Usefulness of PIVKA-II After Living-donor Liver Transplantation for Hepatocellular Carcinoma. Transplant Proc 2017;49:1109-13. [Crossref] [PubMed]
- Si YQ, Wang XQ, Fan G, et al. Value of AFP and PIVKA-II in diagnosis of HBV-related hepatocellular carcinoma and prediction of vascular invasion and tumor differentiation. Infect Agent Cancer 2020;15:70. [Crossref] [PubMed]
- Feng H, Li B, Li Z, et al. PIVKA-II serves as a potential biomarker that complements AFP for the diagnosis of hepatocellular carcinoma. BMC Cancer 2021;21:401. [Crossref] [PubMed]
- Lee JH, Lee JM, Kim SJ, et al. Enhancement patterns of hepatocellular carcinomas on multiphasicmultidetector row CT: comparison with pathological differentiation. Br J Radiol 2012;85:e573-83. [Crossref] [PubMed]
- Ogihara Y, Kitazume Y, Iwasa Y, et al. Prediction of histological grade of hepatocellular carcinoma using quantitative diffusion-weighted MRI: a retrospective multivendor study. Br J Radiol 2018;91:20170728. [Crossref] [PubMed]
- Yang T, Wei H, Wu Y, et al. Predicting histologic differentiation of solitary hepatocellular carcinoma up to 5 cm on gadoxetate disodium-enhanced MRI. Insights Imaging 2023;14:3. [Crossref] [PubMed]
- Honda H, Tajima T, Kajiyama K, et al. Vascular changes in hepatocellular carcinoma: correlation of radiologic and pathologic findings. AJR Am J Roentgenol 1999;173:1213-7. [Crossref] [PubMed]
- Kudo M. Multistep human hepatocarcinogenesis: correlation of imaging with pathology. J Gastroenterol 2009;44:112-8. [Crossref] [PubMed]
- Matsui O. Imaging of multistep human hepatocarcinogenesis by CT during intra-arterial contrast injection. Intervirology 2004;47:271-6. [Crossref] [PubMed]
- Harris AL. Hypoxia--a key regulatory factor in tumour growth. Nat Rev Cancer 2002;2:38-47. [Crossref] [PubMed]
- Brown JM. The hypoxic cell: a target for selective cancer therapy--eighteenth Bruce F. Cain Memorial Award lecture. Cancer Res 1999;59:5863-70. [PubMed]
- Suzuki H, Murata K, Gotoh T, et al. Phenotype-dependent production of des-γ-carboxy prothrombin in hepatocellular carcinoma. J Gastroenterol 2011;46:1219-29. [Crossref] [PubMed]
- Galle PR, Foerster F, Kudo M, et al. Biology and significance of alpha-fetoprotein in hepatocellular carcinoma. Liver Int 2019;39:2214-29. [Crossref] [PubMed]
- Głowska-Ciemny J, Szymański M, Kuszerska A, et al. The Role of Alpha-Fetoprotein (AFP) in Contemporary Oncology: The Path from a Diagnostic Biomarker to an Anticancer Drug. Int J Mol Sci 2023;24:2539. [Crossref] [PubMed]
- Gotsman I, Israeli D, Alper R, et al. Induction of immune tolerance toward tumor-associated-antigens enables growth of human hepatoma in mice. Int J Cancer 2002;97:52-7. [Crossref] [PubMed]
- Tsujikawa H, Masugi Y, Yamazaki K, et al. Immunohistochemical molecular analysis indicates hepatocellular carcinoma subgroups that reflect tumor aggressiveness. Hum Pathol 2016;50:24-33. [Crossref] [PubMed]
- Shinkawa H, Tanaka S, Kabata D, et al. The Prognostic Impact of Tumor Differentiation on Recurrence and Survival after Resection of Hepatocellular Carcinoma Is Dependent on Tumor Size. Liver Cancer 2021;10:461-72. [Crossref] [PubMed]
- Kwon SK, Yun SS, Kim HJ, et al. The risk factors of early recurrence after hepatectomy in hepatocellular carcinoma. Ann Surg Treat Res 2014;86:283-8. [Crossref] [PubMed]
- Zhou L, Rui JA, Wang SB, et al. Early recurrence in large hepatocellular carcinoma after curative hepatic resection: prognostic significance and risk factors. Hepatogastroenterology 2014;61:2035-41. [PubMed]
- Hu J, Gong N, Li D, et al. Identifying hepatocellular carcinoma patients with survival benefits from surgery combined with chemotherapy: based on machine learning model. World J Surg Oncol 2022;20:377. [Crossref] [PubMed]
- Ding J, Wen Z. Survival improvement and prognosis for hepatocellular carcinoma: analysis of the SEER database. BMC Cancer 2021;21:1157. [Crossref] [PubMed]
- Colecchia A, Schiumerini R, Cucchetti A, et al. Prognostic factors for hepatocellular carcinoma recurrence. World J Gastroenterol 2014;20:5935-50. [Crossref] [PubMed]
- Hwang YJ, Bae JS, Lee Y, et al. Classification of microvascular invasion of hepatocellular carcinoma: correlation with prognosis and magnetic resonance imaging. Clin Mol Hepatol 2023;29:733-46. [Crossref] [PubMed]
- Cho ES, Choi JY. MRI features of hepatocellular carcinoma related to biologic behavior. Korean J Radiol 2015;16:449-64. [Crossref] [PubMed]