Program death-1 (PD-1) receptor pathway inhibition in cancer medicine: a perspective on clinical efficacy and associated toxicities
Cancer immunotherapy
Inducing specific recognition and destruction of tumors by the host’s immune system has been a promising but elusive treatment strategy for several decades. Historical observations of striking immune mediated anti-tumor responses among cancer patients have stimulated substantial research efforts aimed at identifying the factors involved in these processes. Clinical observations of patients who attained substantial tumor responses after episodes of systemic infections caused by Streptococcus pyogenes, led Dr. Coley to design a series of experiments injecting streptococcal cultures to patients with sarcoma to evaluate the potential to induce immune cross-reactivity and eradication of tumor cells (1,2). Subsequent efforts culminated with the approval of intracavitary administration of Bacillus Calmette-Guerin (BCG) to treat patients with superficial non-muscle invasive urothelial carcinomas of ther bladder (3), interferon for several tumor types (4-6), and interleukin-2 for melanoma and renal cell carcinoma (7,8), among others.
Immunological tolerance
Normal individuals are tolerant to their own antigens and discriminate against foreign antigens. During the maturation process in lymphoid organs—usually before birth, all lymphocytes undergo a phase, in which antigen exposure results in tolerance instead of activation. Clones of lymphocytes that become active when exposed to self-antigens are suppressed to avoid responses against self-antigens. This process is known as central tolerance. Alternatively, peripheral tolerance is induced by the recognition of antigens without adequate levels of co-stimulators—which are necessary for the activation of lymphocytes or by the repeated and persistent stimulation by self-antigens in peripheral tissues.
The term immunological tolerance was first described by Sir Frank Burnet in 1949 and later confirmed by Billingham, Brent, and Medawar in 1953 through experiments with different strains of CBA and A strain mice and Rhode Island Red and White Leghorn chicken (9). In these experiments, the investigators, demonstrated: (I) that immunological tolerance develops in utero. Mice and chicken never or very limitedly develop strong immunological reactions against foreign antigens inoculated in utero. These animals become tolerant to the inoculated tissue and to re-exposure to the same antigen in their adult life; (II) acquired immunological tolerance is highly specific: The inoculated animals maintained their tolerance for the originally exposed antigens, while rejecting other foreign tissues; (III) acquired immunological tolerance is due to a host’s specific acceptance of foreign antigens rather than a modification in the inoculated tissues. These pivotal discoveries made Sirs Medawar and Burnet the recipients of the Nobel prize of Medicine in 1960 and served as cornerstone for the development of organ transplantation and modern immunological therapies (10). Moreover, the role of immunological tolerance in the etiology of cancer became widely recognized (11).
“Programmed death-1” (PD-1) receptor and its ligands
PD-1 (CD279) is an Immunoglobulin superfamily member expressed in a subpopulation of CD4−CD8− normal thymocytes and induced in peripheral lymphocytes following activation. Ishida, Honjo and others discovered the receptor searching for genes associated with programmed cell death, or apoptosis in 1992 (12). Subsequently, Nishimura and colleagues developed the PD-1 knockout mouse model (13). These mice grow normally but develop moderate splenomegaly. Unlike the CTLA-4 knockout mouse model, the PD-1 knockout mouse survives (14). Their proliferative B cell response is augmented along with increased serum levels of certain immunoglobulins (13). PD-1 deficient mice also develop a number of autoimmune diseases, suggesting the very important role of this receptor in immunologic tolerance through negative regulation of proliferation and differentiation of B cells. Multiple subsequent studies confirmed the importance of the B7-H1/CD80 pathway in the induction and maintenance of tolerance in T cells (15).
Characteristics of PD-1 and PD-L1
The PD-1 protein is a co-inhibitor receptor of T cells with a similar structure to that of CTLA-4 but with different biologic function and specificity for ligands. PD-1 has two known ligands: PD-L1 (B7-H1 or CD274) and PD-L2 (B7-DC or CD273). There is greater affinity for PD-L1. Unlike the ligands of CTLA-4 CD80 (B7-1) and CD86 (B7-2), PD-L1 is selectively expressed and inducible in lymphoid, and non-lymphoid tissues; in different tumors (16) and in other cells of the tumor microenvironment, in response to inflammatory stimuli (17). The expression of PD-L2 is more limited (18). Latchman and colleagues described PD-L2, a second ligand for PD-1 and proved that inhibition of PD-L2, substantially inhibits T cell receptor (TCR)-mediated proliferation and cytokine production by CD4+ T cells. These researchers also demonstrated redundancy in the activity of these two receptors (19). The PD-1/PD-L1 pathway can be used by tumor cells for their own protection from immunological responses mediated by T cells (20,21). In fact, an increased regulation of PD-L1 is associated with decreased immunological activation and adverse clinical results. The increased regulation of PD-L1 in tumor cells can inhibit the production of cytokines and cytolytic activity of PD-1(+) and tumor-infiltrating T cells with CD4(+) and CD8(+) surface expression. Hence, the inhibition of PD1 and PD-L1 is known to enhance the immune responses in vitro and mediate anti-tumor activity in animals (22) and humans (Figure 1).
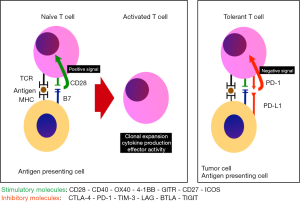
Expression of PD-L1 in human malignancies
The immunohistochemistry expression of PD-L1 by cancer cells varies substantially (23). PD-L1 is overexpressed on the surface of non-small cell lung cancer (NSCLC) cells between 21–95%; melanoma 38–100%; kidney cancer 14–44%; bladder 20–28%; head and neck 31–66%; breast 18–50%; thymic carcinoma 88-100%; multiple myeloma 93% (23). Several groups of investigators have reported worse clinical outcomes among a variety of patients with PD-L1(+) expressing malignancies (24-28). Recently, Zhang and others reported a meta-analysis confirming an adverse prognosis associated with the expression of PD-L1 on tumor cells and PD-1 on tumor-infiltrating lymphocytes (TIL) by immunohistochemistry in patients with epithelial-originating malignancies (29). The investigators found a significantly poorer survival among patients with PD-L1(+) epithelial malignancies compared with those with PD-L1(−) tumor tissues (HR 1.81; 95% CI, 1.33–2.46; P<0.001). Similarly, patients with PD-1(+) TILs had significantly shorter overall survival than the PD-1(−) group (HR 2.53; 95% CI, 1.22–5.21; P=0.012). Furthermore, all subgroups with PD-L1(+) tumors showed consistent trends toward unfavorable prognoses regardless of the assay utilized for the evaluation of PD-L1. The expression of PD-L1 has also been studied in hematological malignancies including Hodgkin’s disease, non-Hodgkin’s lymphomas, and multiple myeloma (30-33). In classic Hodgkin’s lymphoma, alterations in chromosome 9p24.1 increase the abundance of the PD-1 ligands, PD-L1 and PDL2, and promote their induction through Janus kinase (JAK) signal transducer and activator of transcription (STAT) signaling. Early responses in patients with Hodgkin’s disease led to a clinical trial for patients with relapsed or refractory disease (ClinicalTrials.gov number, NCT01592370). Twenty of 23 patients attained objective responses (4 complete responses and 16 partial responses). The rate of progression-free survival at 24 weeks was 86% (95% CI, 62% to 95%). The median survival for responders had not been reached after 40 weeks of follow-up (34).
PD1 and PD-L1 inhibition as cancer therapies
Ten monoclonal antibodies with high affinity for PD-1 or its ligands are under development (Table 1). Extensive basic and clinical research has demonstrated important signals of anti-tumor activity in several tumor types. As of July 2016, this group of agents has received approval in combination with ipilimumab for the treatment of patients with BRAF V600 wild-type, unresectable or metastatic melanoma (nivolumab) or unresectable or metastatic melanoma and disease progression following ipilimumab and, if BRAF V600 mutation positive, a BRAF inhibitor (pembrolizumab) (36-41), metastatic squamous NSCLC with progression on or after platinum-based chemotherapy (nivolumab) (42,43), or metastatic NSCLC whose tumors express PD-L1 as determined by an FDA-approved test, with disease progression on or after platinum-containing chemotherapy (pembrolizumab) (44), advanced renal cell carcinoma (nivolumab), in patients who have received prior anti-angiogenic therapy (45,46), locally advanced or metastatic urothelial carcinoma (atezolizumab) who have experienced disease progression during or following platinum-containing chemotherapy or have disease progression within 12 months of neoadjuvant or adjuvant treatment with platinum-containing chemotherapy (47), and patients with Hodgkin’s disease (nivolumab) that have relapsed or progressed after autologous hematopoietic stem cell transplantation (HSCT) and post-transplantation treatment with brentuximab vedotin (Adcetris) (34). Approval for recurrent head and neck carcinoma is expected before the end of 2016 and submissions for other indications are underway. While a major advance in cancer medicine, treatment with this group of agents is associated with major objective responses in a limited group of patients—similar to traditional cytotoxics; and much like chemotherapy, the combination of these agents result in greater toxicity (38). Nonetheless, in contrast with traditional cytotoxic chemotherapy, the responses induced by checkpoint inhibitors can be long lasting, and occasionally, major anti-tumor responses may follow long-term stabilization of tumor growth (48).
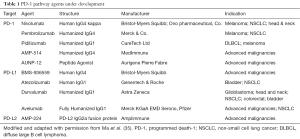
Full table
Biological markers
The U. S. Food and Drug Administration approved 18 new cancer agents in 2015, and most of them corresponded to targeted therapies. Predictive biomarkers for these therapies are aimed at selecting subgroups of patients with the greatest likelihood of benefit while sparing others from unnecessary expenditures and toxicities. Hence, the development of specific biomarkers concomitantly with these agents has become more important than ever before (49). Ascertaining biological markers for immune modulating therapies are particularly challenging due to our limited knowledge of the immune system and its dynamic interactions with the microenvironment and other cellular structures. These limitations have led to a lack of uniform standardization, quantification, and interpretation of predictive biomarkers in immunology (50).
As previously discussed, while PD-L1 is widely expressed among different tumor tissues, PD-L2 is more often restricted to immune cells. Therefore, the immunohistochemical evaluation of PD-L1 expression on tumor cells has become the most accepted predictive biomarker for PD-1/PD-L1 blocking therapies. It is generally accepted that high tumor expression of PD-L1 correlates with greater response rates, duration of response, and overall survival (44). However, a considerable proportion of PD-L1(−) patients experience substantial anti-tumor responses and significant differences in survival according to PD-L1 status have not been uniformly observed across all clinical trials. Hence, a universal acceptance and application of this marker remains controversial (42,45,51). In an attempt to further evaluate the prognostic value of PD-L1 expression, Aguiar and collaborators studied the records of 1979 patients with NSCLC enrolled in 13 clinical trials using Cochrane methodology (52). The investigators found a 29% response rate among 652 PD-L1(+) patients. In contrast, a 13% response rate was found among 915 patients with PD-L1(−) tumor samples (RR 2.08; 95% CI, 1.49–2.91; P<0.01). In addition to confirming an association between overall response rate (ORR) and PD-L1 status, there was an association with the intensity of PD-L1 expression independently of the immunohistochemistry assay utilized in the study (i.e., DAKO 28-8, VENTANA SP142, DAKO 22C3). The 24-week progression free survival was also evaluated in 6 of the studies included in the analysis. Among them, the ORR was 35% for 358 PD-L1(+) patients and 26% for 409 PD-L1(−) patients. This difference was also statistically significant (RR 0.79; 95% CI, 0.71–0.89; P<0.01). Interestingly, the 1-year survival rates were not different. The survival rate for 617 PD-L1(+) positive patients was 28% versus 27% for 779 PD-L1(−) patients. The heterogeneity of the groups was substantial and the difference did not reach statistical difference (RR 0.96; 95% CI, 0.87–1.06; P=0.39).
To add further complexity to the evaluation of PD-L1 expression as a biomarker, the biologicals currently under development have adopted different methodologies and cut-off points. An example of the conflicting preliminary results in this respect, is the FDA approval of nivolumab for the treatment of patients with metastatic NSCLC regardless of their PD-L1 status whereas the approval of pembrolizumab for the same patient population was limited to those with positive PD-L1 expression on their tumor tissues based on data submitted by the sponsor of the trial demonstrating superior efficacy among patients who expressed PD-L1 >50% using a tumor proportion score (53). Some of the challenges posed by this biomarker have been described and include: technical differences challenges related to assay performance, intra-tumoral heterogeneity of biomarker expression, and dynamic changes in PD-L1 expression related to previous therapies (51,54). Several groups of investigators continue to explore other biomarkers at a cellular (CD8+ T cells) and genomic (mismatch repairs in colorectal carcinoma) to be utilized alone or in combination with PD-L1 tumor expression (55).
Safety profile
The blockade of co-stimulatory receptors/ligands involved in inhibition of T cell activation is critical to overcome immunological tolerance. Therefore, it is intuitive to find a myriad of immune related adverse events associated with the safety profile associated with these agents. Moreover, these toxicities are of greater incidence and intensity in regimens combining checkpoint inhibitors such as an anti-CTLA4 antibody (ipilimumab) with PD-1 or PD-L1 antibodies (38).
Eigentler, Hassel, et al. comprehensively reviewed the safety profile and current recommendations for the treatment of immune related adverse events associated with PD-1 and PD-L1 blockade (56). Their manuscript is a comprehensive description of toxicities observed during the development of PD1 and PD-L1 inhibitors, and thoroughly visits each major group of endocrinopathies describing the time to presentation after treatment, their incidence in different clinical trials, and most importantly, the current treatment recommendations according to the type and severity of the adverse event.
For the most part, the toxicities associated with PD-1/PD-L1 inhibitors are grade 1 or 2 and easily manageable. Grade 3 or 4 toxicities are observed in approximately 1–3% of all patients. It is common practice to treat all grade 2 adverse events with corticosteroids (prednisone or methylprednisolone 1 mg/kg) until improvement to grade 1 or complete resolution. Therapy with the PD-1/PD-L1 inhibitor may continue thereafter once the steroids are tapered. Grade 3 or 4 toxicities also require systemic steroid therapy. However, on occasion, different types of adverse events may require higher doses of steroids, or the use of more potent immune suppressants. In these cases, and with the exception of hypothyroidism—in which thyroid supplementation is implemented, permanent discontinuation of the PD-1 inhibitor is recommended.
Discussion
The discovery of Immune checkpoint inhibitors constitutes the greatest historical advancement in the field of immunotherapy. Their importance is several folds. By renewing enthusiasm in the field of immunotherapy, immune checkpoint inhibitors have attracted major attention of researchers, clinicians, patients, and public in general. Such attention has resulted in large financial investments and established immuno-oncology as a solid field of cancer medicine.
Approximately ten different agents are being developed against different malignancies with promising preliminary results. The U.S. FDA has granted approval for five different tumor types including melanoma, NSCLC, renal cell carcinoma, bladder cancer, and Hodgkin’s disease. Several other indications are in the process of completing their developing pathways and await or are under review by U.S. Federal authorities for approval. Current data available demonstrate improved safety and efficacy over selected traditional cytotoxics (43) and excellent efficacy in front line, or as second (39) or third line options (34) in different tumor types. Furthermore, fewer patients experience grade 3 or 4 toxicities when compared with traditional chemotherapy. In a majority of patients, toxicities are mild and manageable. Patients with moderate to severe toxicities require corticosteroids and their outcome is good for the most part (56). The development of other novel therapeutics with different mechanisms of action in immuno-oncology will rapidly demonstrate toxicity and safety signals allowing us to incorporate them to the management of patients with different types of solid and hematological malignancies. This rapid explosion of available options will soon lead to training programs in the field of immuno-oncology around the globe and accelerate future discoveries.
Acknowledgments
The author thanks all the patients and families who have participated in clinical trials developing immune checkpoint inhibitors. It is because of them that many more patients are receiving benefit.
Funding: None.
Footnote
Provenance and Peer Review: This article was commissioned and reviewed by the Section Editor Xia Fang (Department of hematology, Shanghai Tongji Hospital, Tongji University School of Medicine, Shanghai, China).
Conflicts of Interest: Dr. Camacho is a member of the bureau of speakers for Merck Inc. and has served as member in several advisory boards for Merck Inc., Bristol Myers Squibb, and Amgen. Dr. Camacho also served as principal investigator for Pembrolizumab, Avelumab, and MDX-1105.
Ethical Statement: The authors are accountable for all aspects of the work in ensuring that questions related to the accuracy or integrity of any part of the work are appropriately investigated and resolved.
Open Access Statement: This is an Open Access article distributed in accordance with the Creative Commons Attribution-NonCommercial-NoDerivs 4.0 International License (CC BY-NC-ND 4.0), which permits the non-commercial replication and distribution of the article with the strict proviso that no changes or edits are made and the original work is properly cited (including links to both the formal publication through the relevant DOI and the license). See: https://creativecommons.org/licenses/by-nc-nd/4.0/.
References
- Parish CR. Cancer immunotherapy: the past, the present and the future. Immunol Cell Biol 2003;81:106-13. [Crossref] [PubMed]
- Coley WB. The treatment of malignant tumors by repeated inoculations of erysipelas: with a report of ten original cases. Am J Med Sci 1893;105:487-511. [Crossref]
- Morales A, Eidinger D, Bruce AW. Intracavitary Bacillus Calmette-Guerin in the treatment of superficial bladder tumors. J Urol 1976;116:180-3. [PubMed]
- Talpaz M, Kantarjian HM, McCredie K, et al. Hematologic remission and cytogenetic improvement induced by recombinant human interferon alpha A in chronic myelogenous leukemia. N Engl J Med 1986;314:1065-9. [Crossref] [PubMed]
- Kirkwood JM, Strawderman MH, Ernstoff MS, et al. Interferon alfa-2b adjuvant therapy of high-risk resected cutaneous melanoma: the Eastern Cooperative Oncology Group Trial EST 1684. J Clin Oncol 1996;14:7-17. [PubMed]
- Einhorn N, Ling P, Einhorn S, et al. A phase II study on escalating interferon doses in advanced ovarian carcinoma. Am J Clin Oncol 1988;11:3-6. [Crossref] [PubMed]
- Atkins MB, Lotze MT, Dutcher JP, et al. High-dose recombinant interleukin 2 therapy for patients with metastatic melanoma: analysis of 270 patients treated between 1985 and 1993. J Clin Oncol 1999;17:2105-16. [PubMed]
- Rosenberg SA, Yang JC, Topalian SL, et al. Treatment of 283 consecutive patients with metastatic melanoma or renal cell cancer using high-dose bolus interleukin 2. JAMA 1994;271:907-13. [Crossref] [PubMed]
- Billingham RE, Brent L, Medawar PB. Actively acquired tolerance of foreign cells. Nature 1953;172:603-6. [Crossref] [PubMed]
- Brent L. The 50th anniversary of the discovery of immunologic tolerance. N Engl J Med 2003;349:1381-3. [Crossref] [PubMed]
- Anderson MR, Green HN. Immunological implications of acquired tolerance to heterologous tumours. Nature 1963;198:861-2. [Crossref] [PubMed]
- Ishida Y, Agata Y, Shibahara K, et al. Induced expression of PD-1, a novel member of the immunoglobulin gene superfamily, upon programmed cell death. EMBO J 1992;11:3887-95. [PubMed]
- Nishimura H, Minato N, Nakano T, et al. Immunological studies on PD-1 deficient mice: implication of PD-1 as a negative regulator for B cell responses. Int Immunol 1998;10:1563-72. [Crossref] [PubMed]
- Tivol EA, Borriello F, Schweitzer AN, et al. Loss of CTLA-4 leads to massive lymphoproliferation and fatal multiorgan tissue destruction, revealing a critical negative regulatory role of CTLA-4. Immunity 1995;3:541-7. [Crossref] [PubMed]
- Park JJ, Omiya R, Matsumura Y, et al. B7-H1/CD80 interaction is required for the induction and maintenance of peripheral T-cell tolerance. Blood 2010;116:1291-8. [Crossref] [PubMed]
- Dong H, Strome SE, Salomao DR, et al. Tumor-associated B7-H1 promotes T-cell apoptosis: a potential mechanism of immune evasion. Nat Med 2002;8:793-800. [Crossref] [PubMed]
- Curiel TJ, Wei S, Dong H, et al. Blockade of B7-H1 improves myeloid dendritic cell-mediated antitumor immunity. Nat Med 2003;9:562-7. [Crossref] [PubMed]
- Flies DB, Sandler BJ, Sznol M, et al. Blockade of the B7-H1/PD-1 pathway for cancer immunotherapy. Yale J Biol Med 2011;84:409-21. [PubMed]
- Latchman Y, Wood CR, Chernova T, et al. PD-L2 is a second ligand for PD-1 and inhibits T cell activation. Nat Immunol 2001;2:261-8. [Crossref] [PubMed]
- Hirano F, Kaneko K, Tamura H, et al. Blockade of B7-H1 and PD-1 by monoclonal antibodies potentiates cancer therapeutic immunity. Cancer Res 2005;65:1089-96. [PubMed]
- Jin HT, Ahmed R, Okazaki T. Role of PD-1 in regulating T-cell immunity. Curr Top Microbiol Immunol 2011;350:17-37. [Crossref] [PubMed]
- Brahmer JR, Tykodi SS, Chow LQ, et al. Safety and activity of anti-PD-L1 antibody in patients with advanced cancer. N Engl J Med 2012;366:2455-65. [Crossref] [PubMed]
- Patel SP, Kurzrock R. PD-L1 Expression as a Predictive Biomarker in Cancer Immunotherapy. Mol Cancer Ther 2015;14:847-56. [Crossref] [PubMed]
- Gao Q, Wang XY, Qiu SJ, et al. Overexpression of PD-L1 significantly associates with tumor aggressiveness and postoperative recurrence in human hepatocellular carcinoma. Clin Cancer Res 2009;15:971-9. [Crossref] [PubMed]
- Thoma C. Prostate cancer: PD-L1 expression is common and indicates poor prognosis. Nat Rev Urol 2016;13:5. [Crossref] [PubMed]
- Lin YM, Sung WW, Hsieh MJ, et al. High PD-L1 Expression Correlates with Metastasis and Poor Prognosis in Oral Squamous Cell Carcinoma. PLoS One 2015;10:e0142656 [Crossref] [PubMed]
- Leite KR, Reis ST, Junior JP, et al. PD-L1 expression in renal cell carcinoma clear cell type is related to unfavorable prognosis. Diagn Pathol 2015;10:189. [Crossref] [PubMed]
- Mu CY, Huang JA, Chen Y, et al. High expression of PD-L1 in lung cancer may contribute to poor prognosis and tumor cells immune escape through suppressing tumor infiltrating dendritic cells maturation. Med Oncol 2011;28:682-8. [Crossref] [PubMed]
- Zhang Y, Kang S, Shen J, et al. Prognostic significance of programmed cell death 1 (PD-1) or PD-1 ligand 1 (PD-L1) Expression in epithelial-originated cancer: a meta-analysis. Medicine (Baltimore) 2015;94:e515 [Crossref] [PubMed]
- Yang ZZ, Grote DM, Ziesmer SC, et al. PD-1 expression defines two distinct T-cell sub-populations in follicular lymphoma that differentially impact patient survival. Blood Cancer J 2015;5:e281 [Crossref] [PubMed]
- Tonino SH, van de Berg PJ, Yong SL, et al. Expansion of effector T cells associated with decreased PD-1 expression in patients with indolent B cell lymphomas and chronic lymphocytic leukemia. Leuk Lymphoma 2012;53:1785-94. [Crossref] [PubMed]
- Krishnan C, Warnke RA, Arber DA, et al. PD-1 expression in T-cell lymphomas and reactive lymphoid entities: potential overlap in staining patterns between lymphoma and viral lymphadenitis. Am J Surg Pathol 2010;34:178-89. [Crossref] [PubMed]
- Armand P. Immune checkpoint blockade in hematologic malignancies. Blood 2015;125:3393-400. [Crossref] [PubMed]
- Ansell SM, Lesokhin AM, Borrello I, et al. PD-1 blockade with nivolumab in relapsed or refractory Hodgkin's lymphoma. N Engl J Med 2015;372:311-9. [Crossref] [PubMed]
- Ma W, Gilligan BM, Yuan J, et al. Current status and perspectives in translational biomarker research for PD-1/PD-L1 immune checkpoint blockade therapy. J Hematol Oncol 2016;9:47. [Crossref] [PubMed]
- Robert C, Long GV, Brady B, et al. Nivolumab in previously untreated melanoma without BRAF mutation. N Engl J Med 2015;372:320-30. [Crossref] [PubMed]
- Larkin J, Chiarion-Sileni V, Gonzalez R, et al. Combined Nivolumab and Ipilimumab or Monotherapy in Untreated Melanoma. N Engl J Med 2015;373:23-34. [Crossref] [PubMed]
- Wolchok JD, Kluger H, Callahan MK, et al. Nivolumab plus ipilimumab in advanced melanoma. N Engl J Med 2013;369:122-33. [Crossref] [PubMed]
- Robert C, Schachter J, Long GV, et al. Pembrolizumab versus Ipilimumab in Advanced Melanoma. N Engl J Med 2015;372:2521-32. [Crossref] [PubMed]
- Robert C, Ribas A, Wolchok JD, et al. Anti-programmed-death-receptor-1 treatment with pembrolizumab in ipilimumab-refractory advanced melanoma: a randomised dose-comparison cohort of a phase 1 trial. Lancet 2014;384:1109-17. [Crossref] [PubMed]
- Ribas A, Puzanov I, Dummer R, et al. Pembrolizumab versus investigator-choice chemotherapy for ipilimumab-refractory melanoma (KEYNOTE-002): a randomised, controlled, phase 2 trial. Lancet Oncol 2015;16:908-18. [Crossref] [PubMed]
- Brahmer J, Reckamp KL, Baas P, et al. Nivolumab versus Docetaxel in Advanced Squamous-Cell Non-Small-Cell Lung Cancer. N Engl J Med 2015;373:123-35. [Crossref] [PubMed]
- Borghaei H, Paz-Ares L, Horn L, et al. Nivolumab versus Docetaxel in Advanced Nonsquamous Non-Small-Cell Lung Cancer. N Engl J Med 2015;373:1627-39. [Crossref] [PubMed]
- Garon EB, Rizvi NA, Hui R, et al. Pembrolizumab for the treatment of non-small-cell lung cancer. N Engl J Med 2015;372:2018-28. [Crossref] [PubMed]
- Motzer RJ, Rini BI, McDermott DF, et al. Nivolumab for Metastatic Renal Cell Carcinoma: Results of a Randomized Phase II Trial. J Clin Oncol 2015;33:1430-7. [Crossref] [PubMed]
- Motzer RJ, Escudier B, McDermott DF, et al. Nivolumab versus Everolimus in Advanced Renal-Cell Carcinoma. N Engl J Med 2015;373:1803-13. [Crossref] [PubMed]
- Rosenberg JE, Hoffman-Censits J, Powles T, et al. Atezolizumab in patients with locally advanced and metastatic urothelial carcinoma who have progressed following treatment with platinum-based chemotherapy: a single-arm, multicentre, phase 2 trial. Lancet 2016;387:1909-20. [Crossref] [PubMed]
- Hamid O, Robert C, Daud A, et al. Safety and tumor responses with lambrolizumab (anti-PD-1) in melanoma. N Engl J Med 2013;369:134-44. [Crossref] [PubMed]
- Lyman GH, Moses HL. Biomarker Tests for Molecularly Targeted Therapies: Laying the Foundation and Fulfilling the Dream. J Clin Oncol 2016;34:2061-6. [Crossref] [PubMed]
- Butterfield LH, Disis ML, Fox BA, et al. A systematic approach to biomarker discovery; preamble to "the iSBTc-FDA taskforce on immunotherapy biomarkers". J Transl Med 2008;6:81. [Crossref] [PubMed]
- Sacher AG, Gandhi L. Biomarkers for the Clinical Use of PD-1/PD-L1 Inhibitors in Non-Small-Cell Lung Cancer: A Review. JAMA Oncol 2016; [Epub ahead of print]. [Crossref] [PubMed]
- Aguiar PN Jr, Santoro IL, Tadokoro H, et al. The role of PD-L1 expression as a predictive biomarker in advanced non-small-cell lung cancer: a network meta-analysis. Immunotherapy 2016;8:479-88. [Crossref] [PubMed]
- FDA. Hematology/Oncology (Cancer) Approvals & Safety Notifications (2016). Listing of all hematology and oncology approvals between 2012 and 2016, U.S. FDA website.
- McLaughlin J, Han G, Schalper KA, et al. Quantitative Assessment of the Heterogeneity of PD-L1 Expression in Non-Small-Cell Lung Cancer. JAMA Oncol 2016;2:46-54. [Crossref] [PubMed]
- Spencer KR, Wang J, Silk AW, et al. Biomarkers for Immunotherapy: Current Developments and Challenges. Am Soc Clin Oncol Educ Book 2016;35:e493-503. [Crossref] [PubMed]
- Eigentler TK, Hassel JC, Berking C, et al. Diagnosis, monitoring and management of immune-related adverse drug reactions of anti-PD-1 antibody therapy. Cancer Treat Rev 2016;45:7-18. [Crossref] [PubMed]