PARP1 promotes tumor proliferation in lenalidomide-resistant multiple myeloma via the downregulation of microRNA-192-5p-AKT signaling
Highlight box
Key findings
• In lenalidomide-resistant cell lines, the expression level of poly(ADP-ribose) polymerase 1 (PARP1) is higher, the proliferation is more rapid, and the apoptosis rate is lower. Additionally, the activated AKT pathway is suppressed by downregulating the expression of microRNA-192-5p (miR-192-5p). The resistance of multiple myeloma (MM) can be saved to some extent by inhibiting PARP1.
What is known, and what is new?
• The expression of PARP1 is increased in MM cells. PARP1 inhibitors can induce the apoptosis of MM cells.
• In lenalidomide-resistant MM, PARP1 promotes tumor proliferation via the downregulation of miRNA-192-5p-AKT signaling.
What is the implication, and what should change now?
• The results of this study provide a theoretical basis for the treatment of lenalidomide-resistant MM patients, such as PARP1 inhibitor therapy. In subsequent clinical trials, we will obtain key data supporting the use of PARP1 inhibitors in lenalidomide-resistant MM patients.
Introduction
Multiple myeloma (MM) is a hematological malignant disease originating from plasma cells, and is the second most common hematological malignancy (1,2). Despite recent advances over the past two decades, including proteasome inhibitors (PIs), chimeric antigen receptor T-cell (CAR-T) therapy, monoclonal antibodies, and immune modulators, MM remains incurable. Dexamethasone is widely administered to patients with MM, but resistance to this drug develops after prolonged exposure (3,4). Notably, patients with MM can experience drug resistance to one or multiple medications, which leads to poor prognosis and reduced overall survival (5). Therefore, it is imperative to explore and understand the mechanism of drug resistance in MM.
Immunomodulatory drugs (IMiDs), such as lenalidomide, thalidomide, and pomalidomide, provide encouraging therapeutic effects for those with MM (6). However, as with other anti-MM medications, patients who receive IMiDs will eventually develop drug resistance (5) and thus experience poor outcomes. In addition to MM, lenalidomide has been widely administered to treat many disorders, such as mantle cell lymphoma, chronic lymphocytic leukemia, and myelodysplastic syndrome with del(5q). Lenalidomide is an immune modulator and common component of first-line treatment of MM patients (7-9). Lenalidomide has multiple mechanisms of action. As a type of IMiD, lenalidomide exerts its pharmacological effects by increasing the production of IL-2 in T cells and decreasing the secretion of pro-inflammatory cytokines (10). Recent research has shown that lenalidomide binds to an E3 ubiquitin ligase complex, modulating the enzyme’s substrate specificity, which in turn leads to the degradation of certain proteins. In lymphocytes, lenalidomide also induces the degradation of Ikaros (IKZF1) and Aiolos (IKZF3), two zinc finger transcription factors (11). For the treatment of MM, lenalidomide takes advantage of the degradation of IKZF1 and IKZF3, which is crucial for B-cell differentiation and MM cell survival (11,12). IKZF1 and IKZF3 regulate a critical network of transcription factors in MM cells, including interferon regulatory factor 4 (IRF4), which is a master regulatory factor of oncogenes in MM cells (13). IKZF3 is a transcription inhibitor of the IL-2 gene. The increase in IL-2 is induced through the degradation of IKZF3 by lenalidomide, and IL-2 plays an immunomodulatory role to some extent (11).
Genomic instability is an important characteristic of MM (14,15). Resistance to lenalidomide often develops in patients on therapy. The prevalence of lenalidomide resistance is increasing in those with MM, and only a small portion of patients with refractory MM receive four or more lines of therapy. Thus, there is an urgent need for new therapeutic options. Novel therapies, like CAR-T therapy, have been shown to produce benefits in therapy responsiveness in lenalidomide-resistant MM patients; however, such patients also experience treatment-related side effects, such as cytokine release syndrome (16). Thus, researchers need to further understand the mechanism of lenalidomide resistance to develop new therapies for MM patients.
The abnormal repair of DNA damage is an important cause of genomic instability in tumors. Targeting DNA damage repair to induce tumor cell death is an important direction in current cancer treatment (17). Poly(ADP-ribose) polymerase 1 (PARP1) is an important member of the DNA damage repair pathway, which plays an important role in the process of DNA damage replacement repair, and promotes the survival of tumor cells (18,19). The enhancement of the DNA damage repair process in tumor cells is significantly associated with the generation of chemotherapy resistance, but the specific molecular mechanisms have not yet been fully elucidated (20). Studies have shown that PARP1 plays an important role in maintaining genomic stability and the transcription process (21,22). Further, several studies have shown that the expression levels of PARP1 are abnormally elevated in various tumor tissues, including melanoma, breast cancer, and lung cancer (23-26). However, the role of PARP1 in MM is not yet clear, and it is not yet known whether it is involved in the pathogenesis of lenalidomide resistance, nor whether inhibiting PARP1 can reverse resistance of MM. Therefore, we sought to explore the molecular mechanism of PARP1 resistance to lenalidomide in MM patients.
MicroRNA (miRNA or miR) is a family of non-coding RNAs with a length between 21 and 25 bases. MiRNAs play important roles in gene expression regulation by binding other messenger RNAs (mRNAs) (27,28). A great number of miRNAs have been reported to be involved in cancer cell survival and drug resistance (29,30). Notably, miR-192-5p downregulates the activation of AKT to suppress refractory cancer cell apoptosis (31). However, it is unclear whether the miR-1925p/AKT axis exists in lenalidomide refractory MM cells and plays a role in drug resistance. The current study showed that this miR-192-5p/AKT mechanism is also involved in lenalidomide-resistant MM cells. Therefore, new therapies targeting the miR-192-5p/AKT axis in drug refractory MM cells should be considered and actively investigated. We present this article in accordance with the MDAR reporting checklist (available at https://tcr.amegroups.com/article/view/10.21037/tcr-24-1543/rc).
Methods
Collection of MM samples and clinical data
Bone marrow samples, whole-blood samples, and the clinical data of patients with MM who were admitted to the Yuyao People’s Hospital of Zhejiang Province from 2018 to 2022 were collected. Patients treated with the bortezomib, lenalidomide, and dexamethasone (RVd) regimen were selected, with 41.18% receiving RVd as first-line treatment, while 58.82% receiving RVd as second-line therapy due to economic conditions. Then patients were divided into the lenalidomide-sensitive group and the lenalidomide-resistant group based on their responses to treatment. MM cells were isolated from bone marrow samples using the cell-sorting technique. The study was conducted in accordance with the Declaration of Helsinki (as revised in 2013). This study protocol was reviewed and approved by the Ethics Committee of the Yuyao People’s Hospital of Zhejiang Province (No. YS2024-07-003), and informed consent was taken from all the patients.
Cell lines
The MM cell line NCI-H929 (H929) was purchased from ATCC (Manassas, VA, USA). The H929 cells were cultured in Roswell Park Memorial Institute medium 1640 (Gibco, Thermo Fisher Scientific, Waltham, MA, USA) with 10% heat-inactivated fetal bovine serum (Gibco) in a humidified atmosphere containing 5% carbon dioxide at 37 ℃.
Establishment of lenalidomide-resistant H929 cells
Lenalidomide-resistant H929 cells were generated by culturing naïve H929 cells in a gradient of concentrations of lenalidomide. Briefly, naïve H929 cells, which are sensitive to lenalidomide (referred to as lenalidomide-sensitive cells) were cultured in gradually increasing concentrations of lenalidomide (from 0.5 to 5 µM) to select lenalidomide refractory cells (referred to as lenalidomide-resistant cells). All the copy number variant (CNV) fingerprints of the H929 cells remained the same before and after selection. All the cells tested negative for mycoplasma.
MTT assay
Cell growth was measured using a 3-(4,5-dimethylthiazole)-2,5-diphenyltetrazolium (MTT) commercial kit (Boehring, Manheim, Germany) in accordance with the manufacturer’s instructions. The untreated cells served as the controls. Each experiment was performed three times. In brief, in a 96-well plate, a total of 8×103 cells were inoculated per well. The cells were cultured under different conditions. At the end of culturing, MTT (5 mg/mL) was added to each well. The culture dish was incubated for 4 h, the supernatant was aspirated, and dimethyl sulfoxide was added. Absorbance was measured at a wavelength of 570 nm.
Apoptosis
MM cells (2×106) were planted into each well of a 6-well plate. After a culture period of 48 h, the cells were collected and stained with annexin-V and propidium iodide for 15 min at room temperature in the dark. After washing, the cells underwent flow cytometry (Attune) to obtain data.
Cell-cycle analysis
The cells were collected by centrifuge and washed with phosphate-buffered saline. The cells were then fixed with 75% ethanol for 12 h. After washing, the cells were treated with ribonuclease to remove RNA contamination. Subsequently, the cells were stained with propidium iodide for 30 min at room temperature in the dark. The cells underwent flow cytometry to acquire data.
Western blot
The cells were collected at the end of the experiment and lysed using radio-immunoprecipitation assay buffer. The proteins were collected by centrifuge. A total of 40 µg of protein from each sample was used to load the sodium dodecyl sulfate-polyacrylamide gel electrophoresis gels. After separation by electrophoresis, the proteins were transferred onto polyvinylidene fluoride membranes. The membranes were blocked with 5% slim milk and incubated with primary antibodies at 4 ℃ overnight. Secondary antibodies were applied for 1 h at room temperature. Blots were developed and visualized using a commercial enhanced chemiluminescence kit (Tanon, Shanghai, China).
qPCR
The cells (5×103/well) were seeded in 96-well plates. Total RNA was extracted using TRizol (Invitrogen). After concentration measurements using NanoRop (Thermo Fisher Scientific), 1 µg of total RNA was used for reverse transcription. A TaqMan miRNA assay kit (Applied Biosystems, Foster City, CA), was applied cDNA synthesis using a PrimeScript RT kit (Takara, Shiga, Japan). The complementary DNA synthesis was performed using a PrimeScript RT kit (Takara, Shiga, Japan). Quantitative polymerase chain reaction (qPCR) was performed using the SYBR-Green PCR Master Mix (Takara, Shiga, Japan). The following miR-192-5p primers were used: F, 5'-GGACTTTCTTCATTCACACCG-3'; R, 5'-GACCACTGAGGTTAGAGCCA-3'. U6 served as the housekeeping control: F, 5'-CTCGCTTCGGCAGCACATATACT-3'; R, 5'-ACGCTTCACGAATTTGCGTGTC-3'. The PCR conditions are as follows: initial denaturation at 95 ℃ for 5 minutes, followed by 40 cycles of denaturation at 95 ℃ for 5 seconds, annealing at 60 ℃ for 30 seconds, and extension at 72 ℃ (duration adjusted based on the target fragment length).
MiRNA mimic transfection
MiR-192-5p, anti-MiR-192-5p, and control mimics (miR-NC) were customized from a commercial vendor (Sangon Biotech, Shanghai, China). The cells were seeded in 6-well plates and grown to 50% confluence upon transfection. The miRNA mimics were transfected with Lipofectamine 2000 (Invitrogen) in accordance with the manufacturer’s instructions.
Enzyme-linked immunosorbent assay (ELISA)
The serum specimens from the MM patients were achieved by centrifuge after collecting whole-blood samples. The serum samples were stored at –80 ℃ until use. The concentrations of PARP1 in the serum were determined using a commercial human PARP1 ELISA kit (Kemmer BioTECH) in accordance with the manufacturer’s instructions.
Statistical analysis
Each experiment was carried out at least in triplicate to confirm reproducibility. The data are presented as the mean ± standard deviation. Differences between two groups were compared using Students’ t-tests. Differences among more than two groups were compared using a one-way analysis of variance (ANOVA) or a two-way ANOVA. A P value of less than 0.05 was considered statistically significant. All the statistical analyses were conducted using GraphPad Prism 8.0.
Results
Acquisition of lenalidomide-resistant MM cell lines by increasing the concentration of lenalidomide in the cell culture
First, we examined the concentration of PARP1 in patients with resistant MM. Patients with lenalidomide-resistant MM harbored increased levels of PARP1 in the serum (Figure 1A). Subsequently, we established lenalidomide-resistant MM cells by culturing naïve cells in media with escalating concentrations of lenalidomide. After selecting the surviving cells (the final concentration for selection was 5 µM), a cell line that was resistant to lenalidomide was established. Lenalidomide-resistant cells survived when lenalidomide was present in the culture medium (Figure 1B). The CNV signature checks indicated that the lenalidomide-resistant cells had identical signatures to the lenalidomide-sensitive cells (data not provided). More importantly, the lenalidomide-resistant MM cells expressed more PARP1 in the mRNA levels (Figure 1C), indicating that PARP1 might be involved in drug resistance in MM.
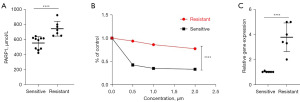
Lenalidomide-resistant MM cell lines have increased growth rates compared to parent cell lines
Next, we sought to characterize the lenalidomide-resistant cells. Lenalidomide-sensitive cells and their resistant counterparts were parallel cultured to compare their growth abilities. Those that were resistant to lenalidomide grew significantly faster (Figure 2). These results indicate that the lenalidomide-resistant H929 cells had a more rapid growth rate in the culture media.
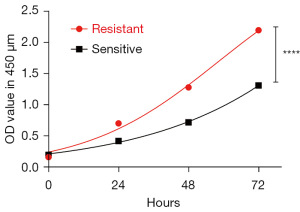
Lenalidomide-resistant MM cell lines have increased rates of proliferation and decreased rates of apoptosis, and altered cell cycles
We also explored the parameters related to cell growth, including proliferation, apoptosis, and the cell cycle. Using Ki67 staining, the lenalidomide-resistant H929 cells showed increased numbers of Ki67 positive cells (Figure 3A). These results showed that the lenalidomide-resistant cells had an increased level of proliferation compared to the naïve lenalidomide-sensitive cells. Moreover, those that were resistant to lenalidomide harbored reduced numbers of apoptotic cells as measured by flow cytometry (Figure 3B). The flow cytometry cell-cycle analysis indicated that the lenalidomide-resistant H929 cells had altered cell cycles, favoring quicker cell growth (Figure 3C). These results showed that the lenalidomide-resistant H929 cells had different characteristics in terms of cell growth compared to the lenalidomide-sensitive cells.
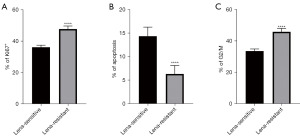
Levels of miRNA-192-5p decrease and levels of p-AKT are elevated in lenalidomide-resistant H929 cells
As it has been reported that miRNA-192-5p and AKT modulate cell growth in the context of drug-resistant MM, we explored the levels of these two factors. The lenalidomide-resistant H929 cells had decreased levels of miRNA-192-5p measured by qPCR, while the lenalidomide-resistant H929 cells had increased levels of p-AKT (the activated form of AKT) in these cells (Figure 4). These findings suggest that the miRNA-192-5p/AKT axis might be involved in the process of lenalidomide resistance.
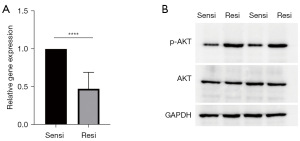
Transfection of miRNA-192-5p mimics reversal lenalidomide resistance in H929 cells
Finally, we sought to study the role of low levels of miRNA-192-5p in lenalidomide-resistant H929 cells. The transfection of miRNA-192-5p in the lenalidomide-resistant cells restored their ability to respond to the presence of lenalidomide in the culture media (Figure 5A). Notably, the transfection of miRNA-192-5p had no bearing on the responsiveness of the naïve H929 cells to lenalidomide. More importantly, the transfection of miRNA-192-5p decreased the activation of AKT (p-AKT) to the level of the naïve lenalidomide-sensitive cells (Figure 5B). Moreover, the transfection of miRNA-192-5p decreased the levels of PARP1 at the mRNA level (Figure 5C). These results highlight the importance of the miRNA-192-5p/AKT/PARP1 axis in regulating drug sensitivity in MM cells.
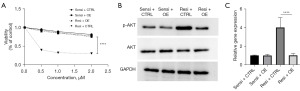
Discussion
In this study, we examined the importance of the miRNA-192-5p/AKT axis in lenalidomide resistance in MM cells. Our established lenalidomide-resistant H929 cells showed different characteristics to naïve cells, including faster growth rates, increased proliferation cells, decreased apoptotic cells, and an altered cell cycle. More importantly, miRNA-192-5p was decreased in the lenalidomide-resistant H929 cells, and AKT was overactivated in these cells. Mechanically, the transfection of miRNA-192-5p restored the responsiveness of the resistant cells to lenalidomide and reduced the levels of p-AKT. All these results indicate that the miRNA-192-5p/AKT axis modulates drug resistance in MM cells.
MM is a common malignant neoplasm of plasma cells that accumulate in bone marrow. MM originates from monoclonal plasma cells accumulating in the bone marrow where these cells produce M-proteins. MM can also cause systemic damage, resulting in hypercalcemia, renal dysfunction, anemia, and bone destruction (32). MM is the second most common hematological malignancy in western countries, with an incidence of 4.5–6 per 100,000 people per year. The median age for the initial diagnosis of MM is approximately 70 years old (33). The prevalence of MM is steadily increasing globally (3).
Currently, the treatment strategies for MM include novel medications, such as PIs, IMiDs, and monoclonal antibodies against the surface targets of MM cells (2). Autologous stem cell transplantation can also be considered in young patients. Despite these advances, patients with MM experience many episodes of relapses in their life time. Each recurrence has a shorter period of remission, and the relapses eventually lead to the death of patients. Therefore, it is imperative to explore drug resistance in MM, among which resistance to lenalidomide is of particular importance, as this is a first-line drug for MM patients.
In the current experimental setting, lenalidomide-resistant MM cells had decreased levels of miRNA-192-5p, which subsequently increased the activation of AKT. AKT controls cell apoptosis, as it induces increased levels of cell death. Therefore, in lenalidomide-resistant MM cells resistance to apoptosis due to decreased levels of AKT activation is a key therapeutic target. The current findings also have clinical applications. The transfection of miR-192-5p mimics restored the responsiveness of cells to lenalidomide. Thus, this strategy could be used in patients with MM that are resistant to lenalidomide. However, this approach warrants further research and clinical trials.
Conclusions
In conclusion, the miR-192-5p/AKT axis plays a crucial role in lenalidomide resistance in MM cells.
Acknowledgments
The authors would like to thank Dr. Huadan Ye for her technical support.
Funding: This work was supported by funding from
Footnote
Reporting Checklist: The authors have completed the MDAR reporting checklist. Available at https://tcr.amegroups.com/article/view/10.21037/tcr-24-1543/rc
Data Sharing Statement: Available at https://tcr.amegroups.com/article/view/10.21037/tcr-24-1543/dss
Peer Review File: Available at https://tcr.amegroups.com/article/view/10.21037/tcr-24-1543/prf
Conflicts of Interest: All authors have completed the ICMJE uniform disclosure form (available at https://tcr.amegroups.com/article/view/10.21037/tcr-24-1543/coif). The authors have no conflicts of interest to declare.
Ethical Statement: The authors are accountable for all aspects of the work in ensuring that questions related to the accuracy or integrity of any part of the work are appropriately investigated and resolved. The study was conducted in accordance with the Declaration of Helsinki (as revised in 2013). This study protocol was reviewed and approved by the Ethics Committee of the Yuyao People’s Hospital of Zhejiang Province (No. YS2024-07-003), and informed consent was taken from all the patients
Open Access Statement: This is an Open Access article distributed in accordance with the Creative Commons Attribution-NonCommercial-NoDerivs 4.0 International License (CC BY-NC-ND 4.0), which permits the non-commercial replication and distribution of the article with the strict proviso that no changes or edits are made and the original work is properly cited (including links to both the formal publication through the relevant DOI and the license). See: https://creativecommons.org/licenses/by-nc-nd/4.0/.
References
- Palumbo A, Anderson K. Multiple myeloma. N Engl J Med 2011;364:1046-60. [Crossref] [PubMed]
- van de Donk NWCJ, Pawlyn C, Yong KL. Multiple myeloma. Lancet 2021;397:410-27. [Crossref] [PubMed]
- Cowan AJ, Green DJ, Kwok M, et al. Diagnosis and Management of Multiple Myeloma: A Review. JAMA 2022;327:464-77. [Crossref] [PubMed]
- Chen Q, Zhang M, Zheng S, et al. Therapeutic progress in relapsed/refractory multiple myeloma. Ann Hematol 2024;103:1833-41. [Crossref] [PubMed]
- Bird S, Pawlyn C. IMiD resistance in multiple myeloma: current understanding of the underpinning biology and clinical impact. Blood 2023;142:131-40. [Crossref] [PubMed]
- Callander NS, Baljevic M, Adekola K, et al. NCCN Guidelines® Insights: Multiple Myeloma, Version 3.2022. J Natl Compr Canc Netw 2022;20:8-19. [Crossref] [PubMed]
- Durie BGM, Hoering A, Abidi MH, et al. Bortezomib with lenalidomide and dexamethasone versus lenalidomide and dexamethasone alone in patients with newly diagnosed myeloma without intent for immediate autologous stem-cell transplant (SWOG S0777): a randomised, open-label, phase 3 trial. Lancet 2017;389:519-27. [Crossref] [PubMed]
- Facon T, Kumar SK, Plesner T, et al. Daratumumab, lenalidomide, and dexamethasone versus lenalidomide and dexamethasone alone in newly diagnosed multiple myeloma (MAIA): overall survival results from a randomised, open-label, phase 3 trial. Lancet Oncol 2021;22:1582-96. [Crossref] [PubMed]
- Facon T, Kumar S, Plesner T, et al. Daratumumab plus Lenalidomide and Dexamethasone for Untreated Myeloma. N Engl J Med 2019;380:2104-15. [Crossref] [PubMed]
- Anderson KC. Lenalidomide and thalidomide: mechanisms of action--similarities and differences. Semin Hematol 2005;42:S3-8. [Crossref] [PubMed]
- Krönke J, Udeshi ND, Narla A, et al. Lenalidomide causes selective degradation of IKZF1 and IKZF3 in multiple myeloma cells. Science 2014;343:301-5. [Crossref] [PubMed]
- Krönke J, Hurst SN, Ebert BL. Lenalidomide induces degradation of IKZF1 and IKZF3. Oncoimmunology 2014;3:e941742. [Crossref] [PubMed]
- Agnarelli A, Chevassut T, Mancini EJ. IRF4 in multiple myeloma-Biology, disease and therapeutic target. Leuk Res 2018;72:52-8. [Crossref] [PubMed]
- Hanahan D, Weinberg RA. Hallmarks of cancer: the next generation. Cell 2011;144:646-74. [Crossref] [PubMed]
- Neri P, Bahlis NJ. Genomic instability in multiple myeloma: mechanisms and therapeutic implications. Expert Opin Biol Ther 2013;13:S69-82. [Crossref] [PubMed]
- Zhang X, Zhang H, Lan H, et al. CAR-T cell therapy in multiple myeloma: Current limitations and potential strategies. Front Immunol 2023;14:1101495. [Crossref] [PubMed]
- Groelly FJ, Fawkes M, Dagg RA, et al. Targeting DNA damage response pathways in cancer. Nat Rev Cancer 2023;23:78-94. [Crossref] [PubMed]
- Hu Y, Lin J, Fang H, et al. Targeting the MALAT1/PARP1/LIG3 complex induces DNA damage and apoptosis in multiple myeloma. Leukemia 2018;32:2250-62. [Crossref] [PubMed]
- Chappidi N, Quail T, Doll S, et al. PARP1-DNA co-condensation drives DNA repair site assembly to prevent disjunction of broken DNA ends. Cell 2024;187:945-961.e18. [Crossref] [PubMed]
- Rose M, Burgess JT, O'Byrne K, et al. PARP Inhibitors: Clinical Relevance, Mechanisms of Action and Tumor Resistance. Front Cell Dev Biol 2020;8:564601. [Crossref] [PubMed]
- Alemasova EE, Lavrik OI. Poly(ADP-ribosyl)ation by PARP1: reaction mechanism and regulatory proteins. Nucleic Acids Res 2019;47:3811-27. [Crossref] [PubMed]
- Engbrecht M, Mangerich A. The Nucleolus and PARP1 in Cancer Biology. Cancers (Basel) 2020;12:1813. [Crossref] [PubMed]
- Mou K, Zhou Y, Mu X, et al. PARP1 Is a Prognostic Marker and Targets NFATc2 to Promote Carcinogenesis in Melanoma. Genet Test Mol Biomarkers 2022;26:503-11. [Crossref] [PubMed]
- Wang J, He G, Li H, et al. Discovery of novel PARP/PI3K dual inhibitors with high efficiency against BRCA-proficient triple negative breast cancer. Eur J Med Chem 2021;213:113054. [Crossref] [PubMed]
- Juncheng P, Lafarge A, Kroemer G, et al. PARP1 inhibition elicits immune responses against non-small cell lung cancer. Oncoimmunology 2022;11:2111915. [Crossref] [PubMed]
- Xiao D, Zeng T, Zhu W, et al. ANXA1 Promotes Tumor Immune Evasion by Binding PARP1 and Upregulating Stat3-Induced Expression of PD-L1 in Multiple Cancers. Cancer Immunol Res 2023;11:1367-83. [Crossref] [PubMed]
- Elkady MA, Yehia AM, Elsakka EGE, et al. miRNAs driving diagnosis, progression, and drug resistance in multiple myeloma. Pathol Res Pract 2023;248:154704. [Crossref] [PubMed]
- Bofill-De Ros X, Vang Ørom UA. Recent progress in miRNA biogenesis and decay. RNA Biol 2024;21:1-8. [Crossref] [PubMed]
- Vahabi M, Dehni B, Antomás I, et al. Targeting miRNA and using miRNA as potential therapeutic options to bypass resistance in pancreatic ductal adenocarcinoma. Cancer Metastasis Rev 2023;42:725-40. [Crossref] [PubMed]
- He H, Zhou J, Cheng F, et al. MiR-3677-3p promotes development and sorafenib resistance of hepatitis B-related hepatocellular carcinoma by inhibiting FOXM1 ubiquitination. Hum Cell 2023;36:1773-89. [Crossref] [PubMed]
- Ren FJ, Yao Y, Cai XY, et al. Emerging Role of MiR-192-5p in Human Diseases. Front Pharmacol 2021;12:614068. [Crossref] [PubMed]
- Gundesen MT, Lund T, Moeller HEH, et al. Plasma Cell Leukemia: Definition, Presentation, and Treatment. Curr Oncol Rep 2019;21:8. [Crossref] [PubMed]
- Kumar SK, Callander NS, Adekola K, et al. Multiple Myeloma, Version 2.2024, NCCN Clinical Practice Guidelines in Oncology. J Natl Compr Canc Netw 2023;21:1281-301. [Crossref] [PubMed]