Pan-cancer analysis combined with experimental validation revealed that KTN1 is an immunological and prognostic biomarker
Highlight box
Key findings
• Kinectin 1 (KTN1) is upregulated in most cancers, knockdown experiment revealed that KTN1 promotes the proliferation and metastasis of head and neck squamous cell carcinoma (HNSC) cells, which provides a new marker for the diagnosis and prognosis of cancers.
What is known and what is new?
• KTN1 is a membrane protein involved in intracellular organelle motility.
• The first pan-cancer analysis of KTN1 found that KTN1 is highly expressed in most cancer tissues and negatively correlated with prognosis, and promoted tumor progression in HNSC.
What is the implication, and what should change now?
• KTN1 has a carcinogenesis effect and is expected to become a cancer biomarker. Further exploration of the oncogenic molecular mechanism of KTN1 is needed.
Introduction
Although the tools for diagnosing and treating cancer are continuously improving, it is still a great challenge to increase the survival of cancer patients. According to the estimation of the International Agency for Research on Cancer, there were 20 million new cancer cases, and nearly 9.7 million related deaths in 2022, and the number of cases may increase to 35 million in 2050 (1). With the continuous development and application of high-throughput sequencing technology in the field of cancer, it is possible to detect cancer mutations in multiple genes on a large scale. The detection of pan-cancer biomarkers, such as sequence mutation changes, gene rearrangements, and copy number changes, can have a positive impact on the treatment and prognosis of cancer patients (2). Thus, to improve the specificity and accuracy of cancer diagnosis and treatment, we need to identify more meaningful genetic markers and the specific roles of these genes across cancers.
Kinectin 1 (KTN1) encodes a membrane protein involved in organelle transport and is located in the endoplasmic reticulum membrane (3,4). The KTN1 protein was first shown to be associated with translation elongation factor EF-δ and to participate in the assembly of the EF-1 complex to regulate the expression of other proteins (5). Recent research on KTN1 has focused mainly its role in some specific cancers. The upregulation of KTN1 in hepatocellular carcinoma tissues may induce tumourigenesis but can also negatively impact tumor development by mediating cell proliferation, invasion, and migration (6). Additionally, in triple-negative breast cancer, the overexpression of KTN1 accelerates the proliferation and invasion of tumor cells, which is related to the poor prognosis of patients (7). Interestingly, it has been proposed that KTN1 can promote the development of skin squamous cell carcinoma through its mediation of MALAT1, which regulates epidermal growth factor receptor (EGFR) in the MALAT1-KTN1-EGFR axis (8). In addition, KTN1 has also been found to be a marker of oral squamous cell carcinoma (9). Therefore, KTN1 is involved in the origin and development of tumors, but its expression and specific molecular mechanism across cancers are still unknown.
In this study, we explored the expression profile of KTN1 across cancers and its correlation with prognosis by making use of data from The Cancer Genome Atlas (TCGA) program. In addition, we explored genetic variation, the immune microenvironment, and the underlying mechanisms involved. Furthermore, we performed experiments to verify the carcinogenic function of KTN1 in head and neck squamous cell carcinoma (HNSC). We provide a theoretical basis for the oncogenic role of KTN1, which highlights the possibility of KTN1 serving as a cancer prognostic biomarker of cancer. We present this article in accordance with the MDAR reporting checklist (available at https://tcr.amegroups.com/article/view/10.21037/tcr-24-752/rc).
Methods
Source of research data
The data were from the TCGA and The Genotype-Tissue Expression (GTEx) website. The KTN1expression and its correlation with cancer-associated fibroblasts (CAFs) infiltration were analyzed in TIMER2.0 (http://timer.comp-genomics.org/) (10). The survival analysis, pathological stage correlation, and co-expression gene correlation analysis of KTN1 were performed on GEPIA2.0 (http://gepia2.cancerpku.cn/) (11). The protein expression level of KTN1 in tumors was analyzed by UALCAN (http://ualcan.path.uab.edu/) (12). The cBioportal (https://www.cbioportal.org/) platform was used to analyze the variation frequency and type of KTN1 (13). STRING database (https://string-db.org/) was used for protein-protein interaction (PPI) network analysis (14). The DAVID online database (https://david.ncifcrf.gov/) was used for Gene Ontology (GO) and Kyoto Encyclopedia of Genes and Genomes (KEGG) signal pathway analysis (15,16). This study was conducted in accordance with the Declaration of Helsinki (as revised in 2013).
Analysis of gene expression
We used the “Gene_DE” module of the TIMER2.0 online database to analyze the expression of KTN1 in 33 types of tumor tissues and corresponding normal tissues. Due to the lack of normal samples for some cancer species in the database, we analyzed and supplemented the cancer species with differential expression of KTN1 through “Expression Analysis Box Plots” in GEPIA2.0 database. To investigate the protein expression level of KTN1 in cancers, we used the CPTAC database of UALCAN online source to generate a box chart for comparison. The “Survival Map” module in the GEPIA2.0 database was used to study the relationship between KTN1 expression and tumor pathological stage.
Analysis of survival
The relationship between KTN1 gene expression and overall survival (OS) and disease-free survival (DFS) of TCGA tumors was inferred by using the “Survival Analysis” module of GEPIA2.0 database. The median expression of KTN1 was set as the cutoff value.
Analysis of genetic variation
We analyzed the genetic variation of KTN1 through the “TCGA Pan-cancer Atlas Studies” dataset in the cBioportal platform, including the mutation type distribution of each domain in the “Mutations” module and the 3-dimensional (3D) model. The correlation between the mutation status of KTN1 and the OS and DFS of TCGA cancer patients was conducted through the “Comparison” module of Uterine Corpus Endometrial Carcinoma (UCEC) dataset.
Analysis of immune microenvironment
The “Immune” module of TIMER2.0 online database was applied to investigate the association between KTN1 and CAFs in various cancer types. We used four algorithms “EPIC”, “MCPCOUNTER”, “XCELL” and “TIDE”. We selected the results with consistency of three or more algorithms to evaluate the infiltration of KTN1 in the tumor.
Analysis of function and mechanism
We investigated the potential binding proteins of KTN1 through STRING database, by setting the following parameters: measuring of network edges (evidence), active interaction sources (experiences), minimum required interaction score (low confidence), and maximum number of actors to show (no more than 50 actors). We analyzed the first 100 genes related to KTN1 expression in the data set of all TCGA tumors and normal tissues through the “Simple Genes Detection” module of GEPIA2.0 database. We used the “Gene_Corr” module of TIMER2.0 database to analyze the correlation between KTN1 and the selected genes. We conducted the pathway enrichment analysis in DAVID online database.
Cell lines and main reagents
The human HNSC cell lines 93UV147 and Cal27 were kindly provided by Dr. Timothy Chan (Memorial Sloan Kettering Cancer Center) (17). All these cells were recently authenticated by Short Tandem Repeat (STR) analysis. Authentication testing and mycoplasma contamination testing results for all cell lines were confirmed. The quantitative real-time polymerase chain reaction (qPCR) kit and the GoScript™ Reverse Transcription System reagent were purchased from Promga. TRIzol reagent was purchased from Invitrogen. qPCR primer sequences are as follows: GAPDH-F 5'-GAAGGTCGGAGTCAACGGATT-3'; GAPDH-R 5'-CCTGGAAGATGGTGATGGGATT-3'; KTN1-F 5'-GGCAGAGATAGCTCACTTGAAG-3'; KTN1-R 5'-ACCTAGCATAATCCTGGCGTAG-3'.
Cell culture and transfection
The human HNSC cell lines 93UV147 and Cal27 were placed in complete Dulbecco’s Modified Eagle’s Medium (DMEM) medium supplemented with 10% fetal bovine serum (FBS), 100 U/mL penicillin and 100 µg/mL streptomycin, cultured in an incubator at 37 ℃ and 5% carbon dioxide (CO2) saturation humidity. 93UV147 and Cal27 cell lines were transfected with riboFECT CP transfection reagent for small interfering RNA (siRNA) and scrambled. According to the instructions, siRNAs sequences are as follows: KTN1siRNA1-GATGGATAATGCTGACTCA, KTN1siRNA2-GAGTGATCTTTCTAGCAAA. After 48 h of transfection, the transfection efficiency was determined by qPCR.
Cell Counting Kit-8 (CCK8) assay
Cell counting kit was used to detect cell proliferation ability. In each well 8×103 cells were seeded in 96-well plates and cultured 48 h in an incubator after transfection. Then 90 µL of DMEM medium and 10 µL of CCK8 reagent were added to each well, and plates were incubated in dark 37 ℃ incubator for 1 hour. Then a microplate reader was used for the measurement.
Colony formation assay
In each well 2×103 cells were seeded in 6-well plates, added the medium containing 10% FBS. After 10 days, the medium was discarded, then per well was fixed it with 4% paraformaldehyde, and stained with 1% crystal violet. The formation of colonies was washed, took photos, and counted.
Transwell assay
Transwell assay was used to detect the migration and invasion of cells. The migration and invasion of cells were evaluated by blank chamber and matrigel invasion chamber respectively. Then 700 µL 10% FBS cell culture medium was added in the lower chamber, 300 µL 0.1% FBS cell culture medium was added in the upper chamber, and 5×104 cells were added in each well. After 36 hours of culture in 5% CO2 incubator at 37 ℃. Giemsa staining was carried out, the non-migrated cells were wiped off and photographed under low power microscope for counting.
Statistical analysis
GraphPad Prism 8.0 software was used for statistical analysis. Comparisons between groups were performed using two independent sample t-tests. The difference was statistically significant at P<0.05. Data were represented as mean ± standard deviation (SD).
Results
KTN1 was increased in tumor tissue of different types of cancer and was associated with tumor progression
In order to explore the mRNA expression level of KTN1 in all TCGA tumors, we used the “Gene_DE” module of TIMER2.0 database for analysis. The results showed that the expression level of KTN1 in cholangiocarcinoma (CHOL), esophageal carcinoma (ESCA), HNSC, liver hepatocellular carcinoma (LIHC), lung squamous cell carcinoma (LUSC) and stomach adenocarcinoma (STAD) tumor tissues was up-regulated relative to the adjacent normal tissues (Figure 1A). For the reason that lacking of expression of some normal tissues in the TIMER2.0 database, we used the GEPIA2 database to cooperate with the analysis. The results showed that the expression level of KTN1 gene in diffuse large B-cell lymphoma (DLBC) and thymic carcinoma (THYM) tumor tissues was significantly higher than the corresponding normal tissues (Figure 1B). In addition, we used the CPTAC database to analyze the protein level of KTN1. The results showed that KTN1 protein was highly expressed in primary tumor tissues of glioblastoma multiforme (GBM), breast invasive carcinoma (BRCA), ovarian cancer (OV), UCEC, lung adenocarcinoma (LUAD), HNSC, pancreatic adenocarcinoma (PAAD) and renal cell carcinoma (RCC) compared with normal tissues (Figure 1C-1J). The above results suggested that KTN1 plays a role as a carcinogen in most tumors.
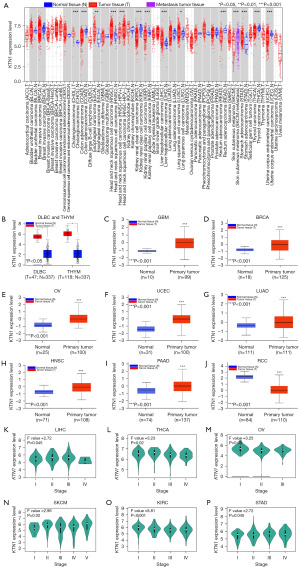
We used GEPIA’s “Expression Analysis” module to further explore the relationship between KTN1 and tumor pathological stages. The results showed that KTN1 is related to the pathological stages of LIHC, thyroid carcinoma (THCA), OV, skin cutaneous melanoma (SKCM), kidney renal clear cell carcinoma (KIRC), and STAD tumors (Figure 1K-1P), indicating that high expression of KTN1 may promote tumor metastasis.
KTN1 impacted the survival of patients with different types of cancer
We investigated the prognostic significance of KTN1 in 33 different types of cancer via the “survival analysis” module of GEPIA2 database. The results showed that the high expression of KTN1 was negatively correlated with the OS prognosis of HNSC patients, while positively correlated with the OS prognosis of KIRC and LIHC patients (Figure 2A). In addition, DFS analysis showed that up-regulation of KTN1 was significantly associated with poor prognosis of adrenocortical carcinoma (ACC), HNSC and LIHC (Figure 2B). The above results indicated that the expression of KTN1 is different from the prognosis of patients with different tumors, but the impact on the total survival and DFS of patients with HNSC cancer was consistent.
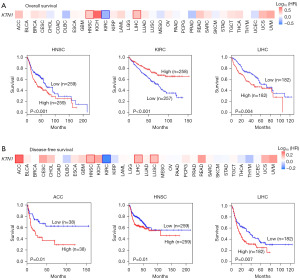
KTN1 alteration impacted the survival of patients with UCEC
We analyzed the variation type of KTN1 in TCGA cancers through cBioportal online database. It can be seen that the variation type of KTN1 was mainly “mutation”, especially in UCEC patients (8.13%). SKCM cases with gene variation were all “mutation” type. In addition, more mutations were “amplification”, even reaching nearly 50% in DLBL cases. Mutation of KTN1 gene was not observed in acute myeloid leukemia (LAML), CHOL, kidney chromophobe (KICH), mesothelioma (MESO), PAAD, testicular germ cell tumors (TGCTs) and THYM patients (Figure 3A). Moreover, it was found that the specific amino acid site with the highest mutation frequency on the domain was K1101Rfs*18/A1102Gfs*15 (Figure 3B), which is highlighted in the corresponding 3D protein domain (Figure 3C). In addition, we investigated the potential relationship between KTN1 gene mutation and survival prognosis of various cancer patients, as shown in the figure, patients with KTN1 mutation in UCEC had better OS prognosis (P=0.050) and progression-free survival prognosis (P=0.04) than those without mutation (Figure 3D). According to the above data, we concluded that KTN1 mutation is the potential cause of tumor formation.
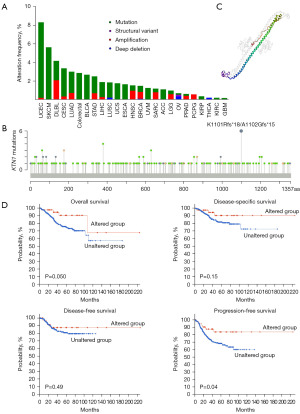
Positive correlation among the number of CAFs and KTN1 expression in different types of cancer
CAFs were highly heterogeneous and highly activated fibroblasts, which had been proven to promote tumorigenesis, migration, inflammation and drug resistance by secreting chemokines and cytokines (18,19). We analyzed the potential association between KTN1 expression level and CAFs through the “Immune” module of TIMER2.0 database. As shown in the figure, we observed a positive correlation among the number of CAFs and KTN1 expression in BRCA, cervical squamous cell carcinoma and endocervical adenocarcinoma (CESC), CHOL, ESCA, HNSC, LIHC, sarcoma (SARC), LUAD, SKCM Metastasis, STAD and THYM, with at least three algorithms showing consistency (Figure 4A). Correlation increases with the depth of color. It was noteworthy that KTN1 expression level was positively associated with CAFs in LIHC (R=0.276), CESC (R=0.354), HNSC-human papilloma virus+ (HNSC-HPV+) (R=0.402), ESCA (R=0.491), CHOL (R=0.492), THYM (R=0.677) (Figure 4B). A publication by Ying Zhang showed that MALAT1 interacts with c-MYC to form a complex which directly bound to the promoter region of KTN1 gene and enhanced its transactivation to positively regulate EGFR protein expression in cutaneous squamous cell carcinoma (8). A novel review discussed high expression and activity of EGFR contributing to collective cell migration in cooperation with CAFs (20). These implied that KTN1 promotes tumor progression by regulating CAFs.
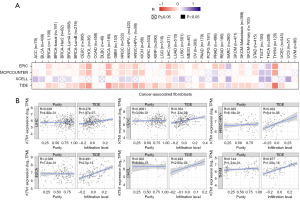
The potential molecular mechanism of KTN1 in tumorigenesis was AMPK signaling pathway
To study the potential molecular mechanism of KTN1 in tumorigenesis, we conducted PPI network analysis via the STRING online tool. We obtained 50 KTN1-binding proteins and their interaction networks (Figure 5A). Furthermore, the expression data of all TCGA tumors were merged in GEPIA2.0 online database, and the first 100 genes most related to KTN1 expression were obtained. We analyzed that KTN1 was positively correlated with their expression levels, namely KTN1-AS1 (R=0.63), MNAT1 (R=0.60), NAA30 (R=0.59), PPP2R5E (R=0.56), and PSMC6 (R=0.55) by substituting the first five genes into TIMER2.0 (Figure 5B,5C). Meanwhile, in combination with the above two data sets, we conducted enrichment analysis of KEGG and GO pathways for the first 100 genes. KEGG data showed that “AMPK signaling pathway” is the related molecular mechanism that has the greatest impact on KTN1’s participation in tumorigenesis. GO pathway analysis showed that these genes participate in metabolic pathways, such as “granulocyte differentiation”, “inclusion body”, “RNA binding”. These results revealed the potential molecular mechanism of KTN1 gene in tumor development (Figure 5D).
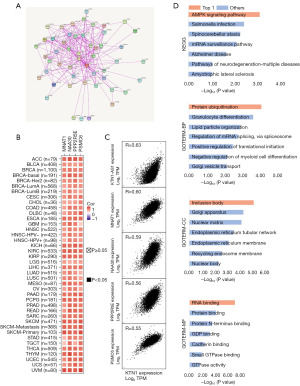
KTN1 promoted the proliferation and metastasis of HNSC cells
To confirm KTN1 is involved in the development of HNSC, we designed the siRNA of KTN1 and performed knockdown efficiency verification in 93UV147 and Cal27 cells (Figure 6A,6B). Subsequently, to study the effect of KTN1 on cell physiology, we silenced KTN1 and found that various physiological behaviors of cells changed significantly, such as cell proliferation (Figure 6C,6D), cell colony formation (Figure 6E-6G), and cell metastasis (Figure 6H-6K). Compared with the control group, 94UV147 and Cal27 cells transfected with the siRNA group (KTN1 siRNA1 or KTN1 siRNA2) significantly inhibited cell proliferation and metastasis. Therefore, we came to the conclusion that KTN1 promotes proliferation and metastasis in HNSC cells.
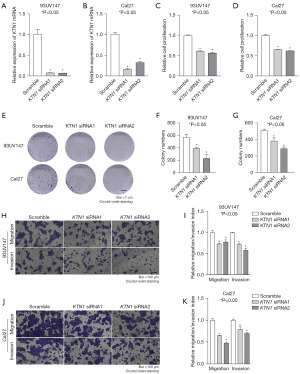
Discussion
Through pan-cancer analysis, this study revealed that KTN1 was differentially expressed in 20 types of tumor tissues compared with the corresponding normal tissues. Specifically, we found that KTN1 was upregulated in 16 types of cancer. These data indicate that KTN1 plays an important role as a carcinogen in these tumors. Interestingly, our results also revealed that KTN1 was upregulated in cancer tissues of metastatic SKCM compared with cancer tissues in situ. Moreover, the data analysis of KTN1 expression during tumor development suggested that it is related to the pathological stages of SKCM, indicating that the high expression of KTN1 may promote the metastasis of SKCM. Therefore, KTN1 plays a certain role in promoting the occurrence and metastasis of tumors. The five-year OS of patients with SKCM is only 19%, indicating that metastasis still poses considerable threat to these individuals (21). At present, the combination of radiotherapy, chemotherapy and biochemical therapy has been widely used in patients with metastatic SKCM, but only minimal clinical benefits can be obtained (22). More effective therapeutic targets are needed. Therefore, the role of KTN1 in metastatic SKCM deserves further exploration.
In this study, the pan-cancer analysis revealed that KTN1 affected the survival of patients with different types of cancer. KTN1 upregulation was negatively correlated with survival of patients with HNSC. Taken together the previous analysis of the carcinogenic effect of KTN1 in HNSC, we conclude that KTN1 may be a target with clinical significance in HNSC. However, the value of KTN1 in HNSC has not yet been explored. Therefore, we further explored the function of KTN1 and found that it promoted the proliferation and metastasis of HNSC cells. We next explored the sensitivity and specificity of KTN1 as a diagnostic marker and therapeutic target for HNSC. We will detect its expression level in patient plasma samples in the next research plan. Collecting these samples is noninvasive and repeatable, which is highly important for monitoring the efficacy of the treatment and recurrence of patients (23,24). KTN1 was significantly upregulated in HNSC-HPV− patients compared with HNSC-HPV+ patients. HPV is an important cause of initiation and development of HNSC, and some studies have shown that, compared with patients with HNSC-HPV−, patients with HNSC-HPV+ are more sensitive to radiotherapy and chemotherapy (25-29). Therefore, we speculated that there is a noteworthy relationship between the high expression of KTN1 expression and patients with HNSC-HPV−.
Normal fibroblasts are exposed to carcinogenic factors and activated to form CAFs. Among a large number of carcinogenic factors, interleukin 6 (IL-6), transforming growth factor beta 1 (TGFβ1), and platelet derived growth factor subunit A S homeolog (PDGF) are widely recognized as fibroblast activating factors that promote the signaling pathways (30,31). Compared with normal fibroblasts, fibroblast activation protein (FAP) alpha, platelet derived growth factor receptor alpha (PDGFRα), and actin alpha 2, smooth muscle, aorta (αSMA) are highly expressed in CAFs, and are therefore used as biomarkers in the isolation of CAFs. CAFs play a role in promoting tumor formation and metastasis by secreting growth factors and remodelling the extracellular matrix. In addition, CAFs are involved in tumor resistance, angiogenesis, and inflammation (32). Our study revealed that the number of CAFs and the expression level of KTN1 in BRCA, CESC, CHOL, ESCA, HNSC-HPV+, LIHC, SARC, SKCM Metastasis, STAD and THYM were consistent (at least three algorithms presented showing the same trend). These findings suggest that KTN1 may be involved in regulating the activation and formation of CAFs.
For the final part of this study, GEPIA2.0 and TIMER2.0 database analyses were performed and revealed that KTN1 was positively correlated with the expression levels of KTN1-AS1, MNAT1, NAA30, PPP2R5E, PSMC6 and other genes. Several studies have shown that KTN1 promotes the cancer progression of various cancers, including HNSC, bladder cancer carcinoma, oral squamous cell carcinoma and triple-negative breast cancer (7,9,33). Enrichment analysis of the KEGG and GO pathways revealed that KTN1 may participate in tumorigenesis and affect clinical prognosis through the AMPK signaling pathway, and the genes that it is co-expressed with may participate in biological processes such as granulocyte differentiation, inclusion body formation, and RNA binding, suggesting that KTN1 may regulate the process of tumorigenesis at the genetic level.
Malignant tumors constitute one of the main diseases that endanger human health. These tumors occur because of genetic and environmental factors (34). The common feature is that the cells in the tumor exhibit uncontrolled growth and abnormal differentiation and leading to local tissue infiltration and distant transplantation (35). To reduce the incidence rate and mortality of malignant tumors, early diagnosis and intervention is the focus. In 2012, TCGA put forwards the “pan-cancer plan” to understand the expression level, mutation status and downstream molecular mechanism of genes through pan-cancer analysis of big data from various platforms; this would provide a basis for studying the clinical significance of genes in the context of clinical trials or the development of new drugs (36). For example, the study found that FGFR2 mutations are relatively common in endometrial cancer, and the common targets are S252W and N549K; patients with these mutations are prone to cancer, but the targeted treatment effects are minimal (37). The expression of SND1 is related to the level of CD8+ T-cell infiltration in colon adenocarcinoma and melanoma, and cancer-related fibroblast infiltration has been observed in other cancer, such as bladder urothelial carcinoma or TGCTs (38). In this study, multiple online database platforms were used to analyze the expression and molecular mechanism of KTN1 in various cancers, providing a basic understanding of the clinical significance of KTN1 in tumorigenesis and development.
Conclusions
KTN1 promotes the proliferation and metastasis of HNSC cells. The pan-cancer analysis of KTN1 revealed its significance in different cancers, which provides a new marker for the diagnosis and prognosis of cancers.
Acknowledgments
We would like to thank the authors of previous studies and the staff members of the cBioPortal and TCGA for providing the available data. We used medical writing service (AJE Editing Service, https://secure.aje.com/).
Funding: This study was supported by funding from
Footnote
Reporting Checklist: The authors have completed the MDAR reporting checklist. Available at https://tcr.amegroups.com/article/view/10.21037/tcr-24-752/rc
Data Sharing Statement: Available at https://tcr.amegroups.com/article/view/10.21037/tcr-24-752/dss
Peer Review File: Available at https://tcr.amegroups.com/article/view/10.21037/tcr-24-752/prf
Conflicts of Interest: All authors have completed the ICMJE uniform disclosure form (available at https://tcr.amegroups.com/article/view/10.21037/tcr-24-752/coif). The authors have no conflicts of interest to declare.
Ethical Statement: The authors are accountable for all aspects of the work in ensuring that questions related to the accuracy or integrity of any part of the work are appropriately investigated and resolved. The study was conducted in accordance with the Declaration of Helsinki (as revised in 2013).
Open Access Statement: This is an Open Access article distributed in accordance with the Creative Commons Attribution-NonCommercial-NoDerivs 4.0 International License (CC BY-NC-ND 4.0), which permits the non-commercial replication and distribution of the article with the strict proviso that no changes or edits are made and the original work is properly cited (including links to both the formal publication through the relevant DOI and the license). See: https://creativecommons.org/licenses/by-nc-nd/4.0/.
References
- Bray F, Laversanne M, Sung H, et al. Global cancer statistics 2022: GLOBOCAN estimates of incidence and mortality worldwide for 36 cancers in 185 countries. CA Cancer J Clin 2024;74:229-63. [Crossref] [PubMed]
- Dong F. Pan-Cancer Molecular Biomarkers: A Paradigm Shift in Diagnostic Pathology. Surg Pathol Clin 2021;14:507-16. [Crossref] [PubMed]
- Ng IC, Pawijit P, Teo LY, et al. Kinectin-dependent ER transport supports the focal complex maturation required for chemotaxis in shallow gradients. J Cell Sci 2016;129:2660-72. [Crossref] [PubMed]
- Vallee RB, Sheetz MP. Targeting of motor proteins. Science 1996;271:1539-44. [Crossref] [PubMed]
- Ong LL, Lin PC, Zhang X, et al. Kinectin-dependent assembly of translation elongation factor-1 complex on endoplasmic reticulum regulates protein synthesis. J Biol Chem 2006;281:33621-34. [Crossref] [PubMed]
- Pan J, Chao NX, Zhang YY, et al. Upregulating KTN1 promotes Hepatocellular Carcinoma progression. J Cancer 2021;12:4791-809. [Crossref] [PubMed]
- Gao L, Chen S, Hong M, et al. Kinectin 1 promotes the growth of triple-negative breast cancer via directly co-activating NF-kappaB/p65 and enhancing its transcriptional activity. Signal Transduct Target Ther 2021;6:250. [Crossref] [PubMed]
- Zhang Y, Gao L, Ma S, et al. MALAT1-KTN1-EGFR regulatory axis promotes the development of cutaneous squamous cell carcinoma. Cell Death Differ 2019;26:2061-73. [Crossref] [PubMed]
- Jakob M, Mattes LM, Unger K, et al. Human microRNA-182-5p and kinectin 1: Potential biomarkers for prognosis in oral squamous cell carcinoma. Head Neck 2021;43:3707-19. [Crossref] [PubMed]
- Li T, Fu J, Zeng Z, et al. TIMER2.0 for analysis of tumor-infiltrating immune cells. Nucleic Acids Res 2020;48:W509-14. [Crossref] [PubMed]
- Tang Z, Kang B, Li C, et al. GEPIA2: an enhanced web server for large-scale expression profiling and interactive analysis. Nucleic Acids Res 2019;47:W556-60. [Crossref] [PubMed]
- Chandrashekar DS, Karthikeyan SK, Korla PK, et al. UALCAN: An update to the integrated cancer data analysis platform. Neoplasia 2022;25:18-27. [Crossref] [PubMed]
- Cerami E, Gao J, Dogrusoz U, et al. The cBio cancer genomics portal: an open platform for exploring multidimensional cancer genomics data. Cancer Discov 2012;2:401-4. [Crossref] [PubMed]
- Szklarczyk D, Gable AL, Nastou KC, et al. The STRING database in 2021: customizable protein-protein networks, and functional characterization of user-uploaded gene/measurement sets. Nucleic Acids Res 2021;49:D605-12. [Crossref] [PubMed]
- Huang da W. Sherman BT, Lempicki RA. Systematic and integrative analysis of large gene lists using DAVID bioinformatics resources. Nat Protoc 2009;4:44-57. [Crossref] [PubMed]
- Huang da W. Sherman BT, Lempicki RA. Bioinformatics enrichment tools: paths toward the comprehensive functional analysis of large gene lists. Nucleic Acids Res 2009;37:1-13. [Crossref] [PubMed]
- Jiang Y, Jiang YY, Xie JJ, et al. Co-activation of super-enhancer-driven CCAT1 by TP63 and SOX2 promotes squamous cancer progression. Nat Commun 2018;9:3619. [Crossref] [PubMed]
- Guo X, Oshima H, Kitmura T, et al. Stromal fibroblasts activated by tumor cells promote angiogenesis in mouse gastric cancer. J Biol Chem 2008;283:19864-71. [Crossref] [PubMed]
- Kojima Y, Acar A, Eaton EN, et al. Autocrine TGF-beta and stromal cell-derived factor-1 (SDF-1) signaling drives the evolution of tumor-promoting mammary stromal myofibroblasts. Proc Natl Acad Sci U S A 2010;107:20009-14. [Crossref] [PubMed]
- Yoshida GJ. Regulation of heterogeneous cancer-associated fibroblasts: the molecular pathology of activated signaling pathways. J Exp Clin Cancer Res 2020;39:112. [Crossref] [PubMed]
- Miller KD, Nogueira L, Mariotto AB, et al. Cancer treatment and survivorship statistics, 2019. CA Cancer J Clin 2019;69:363-85. [Crossref] [PubMed]
- Bhatia S, Tykodi SS, Thompson JA. Treatment of metastatic melanoma: an overview. Oncology (Williston Park) 2009;23:488-96. [PubMed]
- Dumitrescu RG. Early Epigenetic Markers for Precision Medicine. Methods Mol Biol 2018;1856:3-17. [Crossref] [PubMed]
- Arantes LMRB, De Carvalho AC, Melendez ME, et al. Serum, plasma and saliva biomarkers for head and neck cancer. Expert Rev Mol Diagn 2018;18:85-112. [Crossref] [PubMed]
- Marur S, D'Souza G, Westra WH, et al. HPV-associated head and neck cancer: a virus-related cancer epidemic. Lancet Oncol 2010;11:781-9. [Crossref] [PubMed]
- Marur S, Forastiere AA. Head and Neck Squamous Cell Carcinoma: Update on Epidemiology, Diagnosis, and Treatment. Mayo Clin Proc 2016;91:386-96. [Crossref] [PubMed]
- Vidal L, Gillison ML. Human papillomavirus in HNSCC: recognition of a distinct disease type. Hematol Oncol Clin North Am 2008;22:1125-42. vii. [Crossref] [PubMed]
- Smeets SJ, Brakenhoff RH, Ylstra B, et al. Genetic classification of oral and oropharyngeal carcinomas identifies subgroups with a different prognosis. Cell Oncol 2009;31:291-300. [PubMed]
- Lindel K, Beer KT, Laissue J, et al. Human papillomavirus positive squamous cell carcinoma of the oropharynx: a radiosensitive subgroup of head and neck carcinoma. Cancer 2001;92:805-13. [Crossref] [PubMed]
- Chen X, Song E. Turning foes to friends: targeting cancer-associated fibroblasts. Nat Rev Drug Discov 2019;18:99-115. [Crossref] [PubMed]
- Sahai E, Astsaturov I, Cukierman E, et al. A framework for advancing our understanding of cancer-associated fibroblasts. Nat Rev Cancer 2020;20:174-86. [Crossref] [PubMed]
- Nurmik M, Ullmann P, Rodriguez F, et al. In search of definitions: Cancer-associated fibroblasts and their markers. Int J Cancer 2020;146:895-905. [Crossref] [PubMed]
- Hu X, Xiang L, He D, et al. The long noncoding RNA KTN1-AS1 promotes bladder cancer tumorigenesis via KTN1 cis-activation and the consequent initiation of Rho GTPase-mediated signaling. Clin Sci (Lond) 2021;135:555-74. [Crossref] [PubMed]
- Srinivas PR, Kramer BS, Srivastava S. Trends in biomarker research for cancer detection. Lancet Oncol 2001;2:698-704. [Crossref] [PubMed]
- Liu J, Wang X, Deng Y, et al. Research Progress on the Role of Regulatory T Cell in Tumor Microenvironment in the Treatment of Breast Cancer. Front Oncol 2021;11:766248. [Crossref] [PubMed]
- Tomczak K, Czerwińska P, Wiznerowicz M. The Cancer Genome Atlas (TCGA): an immeasurable source of knowledge. Contemp Oncol (Pozn) 2015;19:A68-77. [Crossref] [PubMed]
- Li J, Hu K, Huang J, et al. A Pancancer Analysis of the Expression Landscape and Clinical Relevance of Fibroblast Growth Factor Receptor 2 in Human Cancers. Front Oncol 2021;11:644854. [Crossref] [PubMed]
- Cui X, Zhang X, Liu M, et al. A pan-cancer analysis of the oncogenic role of staphylococcal nuclease domain-containing protein 1 (SND1) in human tumors. Genomics 2020;112:3958-67. [Crossref] [PubMed]