KIF18A and CDK1 as combined therapeutic targets in cervical and endometrial carcinomas: based on bioinformatics analysis and in vitro experiments
Highlight box
Key findings
• We have proposed a novel therapeutic approach for cervical squamous cell carcinoma and endocervical adenocarcinoma (CESC) and uterine corpus endometrial carcinoma (UCEC) by targeting cyclin-dependent kinase 1 (CDK1) in conjunction with kinesin family member 18A (KIF18A).
What is known and what is new?
• CDK1 and KIF18A represent significant potential targets for exerting antitumor effects through the regulation of cell mitosis. However, it remains unclear whether these two targets exhibit synergistic antitumor effects in CESC and UCEC.
• The concurrent inhibition of CDK1 and KIF18A exhibits a synergistic effect in suppressing the proliferation and migration, and promoting apoptosis of CESC and UCEC cells.
What is the implication, and what should change now?
• Our findings introduce a novel combination therapy for the treatment of CESC and UCEC. This therapeutic approach has the potential to establish a new regimen for overcoming resistance to CDK inhibitors.
Introduction
Cervical squamous cell carcinoma and endocervical adenocarcinoma (CESC) and uterine corpus endometrial carcinoma (UCEC) are the most prevalent gynecological tumors (1-3). In western countries, CESC ranks third in incidence, behind UCEC and ovarian cancer (1). According to the latest cancer report in China, CESC and UCEC rank as the first and second most common gynecological tumors, with incidence of 150,700 and 77,700, respectively, in 2022 (1). Although CESC and UCEC typically present with early clinical symptoms and have a favorable prognosis with a 5-year survival rate exceeding 80%, the outlook becomes grim upon recurrence and metastasis, with the 5-year survival rate dropping to less than 20% (4,5). Currently, combination chemotherapy, hormone therapy, targeted therapy, and immunotherapy are the first-line therapies for the treatment of metastatic and recurrent CESC and UCEC. Specifically, pembrolizumab has demonstrated considerable improvements in progression-free survival (PFS) and overall survival (OS) among CESC patients, particularly in those with programmed cell death ligand 1 (PD-L1)-positive tumors. Although trastuzumab and cemiplimab have shown efficacy in other cancers, their role in CESC and UCEC is limited and not well-established (6-9). Nevertheless, the exploration of molecular mechanisms associated with CESC and UCEC and identification of potential therapeutic targets remain crucial aspects in the advancement of targeted drug development.
Kinesin family member 18A (KIF18A) is a mitosis-driving protein that regulates spindle microtubule dynamics and chromosome alignment during cell division, maintaining genome stability and successfully completing mitosis (10). Bioinformatic analysis and immunohistochemical experiments have confirmed the upregulation of KIF18A in 27 kinds of tumors and its correlation with tumor stage (T stage), node stage (N stage), pathological stage, histological grade, and Ki-67 index of various cancers (10-13). KIF18A expression levels are positively correlated with immune cell invasion, immune regulatory genes, immune checkpoints, tumor mutation load, microsatellite instability, mismatch repair, DNA methylation, RNA methylation, and resistance to radiation and chemotherapy (10,14,15). KIF18A knockout causes spindle assembly checkpoint activation to maintain the adhesion stability of centromere microtubules (16). Thus, microtubule-pulled centromere separation is inhibited, mitosis arrest is induced, and the probability of cell death is increased (16,17). Chromosomal instability (CIN) is a hallmark of cancer, caused by persistent errors in chromosome separation during mitosis (18,19). Aggressive cancers such as high-grade serous ovarian cancer (HGSOC) and triple-negative breast cancer (TNBC) have a high frequency of CIN and TP53 mutations (20). TP53-mutated unstable aneuploidy cells characterized by CIN rely on KIF18A motor activity to prevent lethal multipolar cell division (20). KIF18A protein inhibitors activate mitotic checkpoints and selectively kill cancer cells that are chromosomally unstable (20). In TP53-mutated HGSOC and TNBC cell lines characterized by CIN, including cyclin E1 amplification, cyclin-dependent kinase 4 (CDK4) or CDK6 inhibitor resistance, and breast cancer susceptibility gene 1 (BRCA1)-altered cell line models, a very strong sensitivity to KIF18A inhibition has been observed (20). These findings suggest that tumor cells exhibiting CIN may display heightened sensitivity to KIF18A knockdown, indicating a potential therapeutic target for mitotic inhibitors in CIN tumors.
CDK1, a serine/threonine protein kinase, is essential for regulating the cell cycle. In humans, the CDC2 gene encodes CDK1, which forms a complex with cyclin to phosphorylate various substrates, thereby facilitating the advancement of the G1/S phase (21). Moreover, the CDK1 pathway plays a crucial role in safeguarding the attachment of chromosomal microtubules and maintaining chromosome stability, whereas dysregulation of CDK1 expression not only hinders cell proliferation but also contributes to genomic and CIN (22-26). The findings of the aforementioned study suggest that CDK1 and KIF18A are likely to be pivotal in sustaining tumor cell proliferation and CIN. Consequently, the concurrent targeting of CDK1 and KIF18A presents a potential therapeutic target and strategy for combination therapy in the treatment of tumors. Our initial findings indicated a significant up-regulation and positive correlation between CDK1 and KIF18A expression in CESC and UCEC tissues. Bioinformatics analysis suggested that CDK1 and KIF18A could potentially serve as common targets for CESC and UCEC. Subsequently, CESC and UCEC cell lines were employed to validate the synergistic efficacy of CDK1 and KIF18A inhibitors (KIF18Ais) in combating tumors. We present this article in accordance with the MDAR reporting checklist (available at https://tcr.amegroups.com/article/view/10.21037/tcr-24-1831/rc).
Methods
Bioinformatics analysis
Gene expression was analyzed using The Cancer Genome Atlas (TCGA) database (https://portal.gdc.cancer.gov) in the transcripts per million (TPM) reads data format, with R software (version 4.2.1; R Foundation for Statistical Computing, Vienna, Austria) and the ggplot2 package (version 3.3.3), as previous literature (27). KIF18A-associated genes were identified by screening the TCGA database utilizing R software (version 4.2.1) in conjunction with the DESeq2 package (version 1.36.0) and edgeR package (version 3.38.2) (28,29). The Database for Annotation, Visualization and Integrated Discovery (DAVID) online database (https://davidbioinformatics.nih.gov/) was employed to forecast Gene Ontology (GO) terms [including biological process (BP), cellular component (CC), and molecular function (MF)] and Kyoto Encyclopedia of Genes and Genomes (KEGG) pathway enrichment analysis for the genes related to KIF18A. In order to explore potential upstream regulators that influence gene expression patterns, the X2K Appyter (https://maayanlab.cloud/X2K/) database was utilized to examine kinases associated with KIF18A-related genes in CESC and UCEC, as detailed in prior research (30). While not a substitute for experimental validation, the X2K Appyter database offers a degree of insight into the potential indirect correlation between KIF18A and CDK1. The X2K Appyter (Expression2Kinases) predicts upstream regulatory networks associated with user-inputted sets of genes. Discrete query gene sets are compared first to ChEA3 libraries of transcription factor target gene sets assembled from orthogonal ‘omics’ datasets. Afterwards, ChEA3 results are put through a protein-protein interaction database to determine the transcription factor intermediate protein interactors. Finally, protein interactors are compared to the KEA3 background database—which contains measured and predicted kinase-substrate interactions, kinase-protein interactions, and interactions supported by co-expression and co-occurrence data—to determine which kinases may be most closely associated with the transcription factor intermediate protein interactors. Overall, Expression2Kinases can provide a method of identifying upstream regulators likely responsible for observed patterns in genome-wide gene expression. The Human Protein Atlas (HPA; https://www.proteinatlas.org/) databases were used to analyze KIF18A immunohistochemical staining. Input “CDK1” and “KIF18” into the search field, subsequently selecting the subcategories “tissue” and “cancer”. Proceed to examine the immunostaining outcomes for CDK1 and KIF18 in both normal and tumor tissues. The study was conducted in accordance with the Declaration of Helsinki (as revised in 2013).
Cell culture and transfection
HeLa, SiHa, RL95-2, and HEC-151 cell lines were obtained from either the American Type Culture Collection or the Shanghai Cell Bank (Type Culture Collection; Chinese Academy of Sciences, Shanghai, China). These cell lines were cultured in Roswell Park Memorial Institute (RPMI)-1640 medium supplemented with 10% fetal bovine serum, 100 U/mL penicillin, 50 mg/mL streptomycin, and 2 mmol/L glutamine, and were maintained in a humidified CO2 incubator at 37 ℃. The small interfering RNAs (siRNAs), namely si-normal control (si-NC) and si-CDK1, were synthesized by Gene-Pharma (Shanghai, China), whereas Lipofectamine 3000 (Invitrogen, Waltham, MA, USA) was employed for cell transfection in accordance with the manufacturer’s guidelines. The KIF18Ai (AMG-650) and CDK1 inhibitor (CDK1i; RO-3306) were obtained from MedChemExpress (Shanghai, China). The cell experiment involved three biological replicates in each group.
Cell Counting Kit-8 (CCK-8), transwell, and terminal-deoxynucleotidyl transferase-mediated nick end labeling (TUNEL) assays
The CCK-8 kit from the Beyotime Institute of Biotechnology (Haimen, China) was employed for evaluating in vitro cell proliferation, and cell viability was determined using a SpectraMax M5 enzyme-linked immunosorbent assay (ELISA) plate reader from Molecular Devices (LLC, Sunnyvale, CA, USA) at a wavelength of 450 nm. Migration assays were performed using the transwell migration without Matrigel assay obtained from Corning Incorporated (Corning, NY, USA). Subsequent to migration, cells that had crossed the underside of the membrane were fixed in 1% paraformaldehyde, stained with hematoxylin, imaged, and quantified by enumerating the cells in five arbitrarily chosen fields. Cell apoptosis was assessed utilizing a TUNEL assays kit (Roche, Basel, Switzerland). The TUNEL assay outcomes were observed via fluorescence microscopy (BX53; Olympus, Tokyo, Japan), with TUNEL-positive nuclei exhibiting a red hue in the fluorescence images. The rate of apoptosis was quantified by calculating the percentage of TUNEL-positive cells per field utilizing Image-Pro Plus software (Media Cybernetics, Rockville, MD, USA).
Statistical analysis
The data were presented as mean ± standard deviation. Statistical analysis was performed using the software SPSS 23.0 (IBM Corp., Armonk, NY, USA). Intergroup differences were evaluated using one-way analysis of variance (ANOVA) with the post-hoc Tukey honest significant difference (HSD) test. Spearman correlation coefficient was utilized to assess relationships. The log-logistic method was applied for model fitting and determination of the median effect concentration (EC50) value by analyzing cell activity percentages with R software (version 4.2.1), ggplot2 package (version 3.3.6), and drc package (version 3.0-1). A P value less than 0.05 was considered statistically significant.
Results
KIF18A expression in CESC and UCEC
Initially, the TCGA and Genotype-Tissue Expression (GTEx) databases were utilized to assess the expression levels of KIF18A in pan-cancer. Our findings indicated a significant up-regulation of KIF18A in 27 tumor types, including CESC and UCEC, compared to normal tissues (Figure 1A). Subsequently, a comparative analysis of KIF18A expression in paired samples of CESC and UCEC revealed a significant upregulation in UCEC in comparison to paracancerous tissue. Although a similar upward trend was observed in CESC, the difference was not deemed statistically significant (P=0.07), primarily attributed to result bias stemming from the limited sample size (Figure 1B). The results of immunostaining (Figure 1C,1D) and subcellular localization (Figure 1E) analysis from the HPA database revealed elevated expression of the KIF18A protein in cytoplasmic microtubules in both CESC and UCEC.
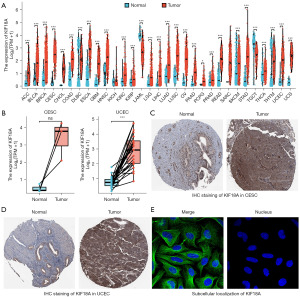
KIF18A-related kinases screening
A thorough examination of the TCGA database revealed the presence of 239 KIF18A-associated genes in CESC and 2,007 genes in UCEC, satisfying the conditions (Spearman correlation coefficient >0.7, P<0.05). Additionally, a subset of 221 KIF18A-related genes was identified to be common between CESC and UCEC, as illustrated in Figure 2A. Our ongoing investigation involved the examination of a subset comprising 221 genes associated with KIF18A through GO and KEGG analyses. Our findings indicated that the genes related to KIF18A primarily participate in chromosome segregation, mitotic cell cycle phase transition, cell cycle G2/M phase transition, cell cycle, microtubule motor activity, and DNA replication (Figure 2B). Given the pivotal role of kinases, particularly cyclins and CDKs, in regulating the cell cycle and DNA synthesis (31,32), our study delved into the potential interactions between a specific subset of 221 KIF18A-related genes and kinases. In order to investigate the correlation between KIF18A-related genes and kinase activity, the X2K Appyter database was employed to identify potential kinases. Analysis of the data indicated that the top 10 kinases, including checkpoint kinase 2 (CHEK2), CDK1, CDK2, CDK7, CDK9, protein kinase, DNA-activated, catalytic subunit (PRKDC), aurora kinase A (AURKA), ataxia telangiectasia and Rad3-related serine/threonine kinase (ATR), ataxia telangiectasia-mutated serine/threonine kinase (ATM), and homeodomain interacting protein kinase 2 (HIPK2), exhibit a strong association with genes associated with KIF18A (Figure 2C). The expression levels of the top 10 kinase genes were confirmed through analysis of TCGA data. Our results demonstrated that CDK1, CDK2, CDK7, CHEK2, PRKDC, and AURKA display significant up-regulation in CESC tissues compared to normal tissues, whereas CDK9, ATM, and HIPK2 exhibited significant down-regulation (Figure 2D). In UCEC tissues (Figure 2E), CDK1, CDK7, CHEK2, PRKDC, and AURKA were significantly up-regulated, whereas CDK9, ATM, and ATR were significantly down-regulated compared to normal tissues.
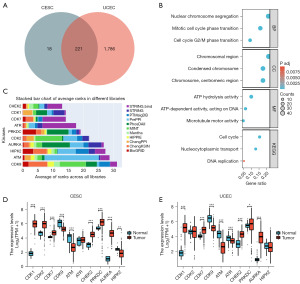
The correlation between KIF18A and kinases
We identified six genes (CDK1, CDK2, CDK7, CHEK2, PRKDC, and AURKA) that exhibited significant upregulation in CESC, and five genes (CDK1, CDK7, CHEK2, PRKDC, and AURKA) that showed significant upregulation in UCEC. The correlation between these genes and KIF18A was subsequently examined. In CESC (Figure 3A), a significant positive correlation was observed between KIF18A and CDK1 (r=0.615; P<0.001), CDK2 (r=0.502; P<0.001), CDK7 (r=0.349; P<0.001), CHEK2 (r=0.372; P<0.001), PRKDC (r=0.479; P<0.001), and AURKA (r=0.531; P<0.001). Similarly, in UCEC (Figure 3B), a significant positive correlation was found between KIF18A and CDK1 (r=0.797; P<0.001), CDK7 (r=0.393; P<0.001), CHEK2 (r=0.711; P<0.001), PRKDC (r=0.679; P<0.001), and AURKA (r=0.788; P<0.001). The aforementioned findings suggest that the association between KIF18A and CDK1 is most pronounced in CESC and UCEC. Consequently, our subsequent cellular experiments primarily focused on exploring the synergistic anti-tumor properties of KIF18A and CDK1.
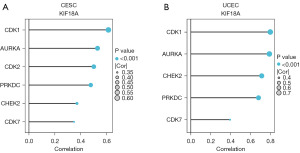
EC50 value of KIF18A and CDK1is in CESC and UCEC cells
To conduct a more in-depth examination of the combined anti-tumor properties of KIF18A and CDK1is, we investigated the EC50 values of the KIF18Ai (AMG-650) and CDK1i (RO-3306) in four cell lines of CESC and UCEC. The EC50 value of KIF18Ai in HeLa, SiHa, RL95-2, and HEC-151 was 0.590, 0.855, 1.391, and 1.800 µM, respectively (Figure 4A). The EC50 value of CDK1i in HeLa, SiHa, RL95-2, and HEC-151 was 2.924, 4.136, 3.419, and 5.485 µM, respectively (Figure 4B).
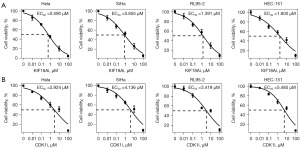
Inhibition of CDK1 enhances the sensitivity of KIF18Ai in CESC and UCEC cells
In this study, we investigated the susceptibility of CESC and UCEC cells to KIF18Ai through the genetic and pharmacological inhibition of CDK1 expression. Our findings demonstrated that transfection with si-CDK1 resulted in a significant decrease in the EC50 value of KIF18Ai compared to transfection with si-NC (Figure 5A and Table 1). Specifically, HeLa (0.654 vs. 0.206 µM), SiHa (0.985 vs. 0.366 µM), RL95-2 (1.433 vs. 0.681 µM), and HEC-151 (1.943 vs. 0.485 µM) exhibited reduced EC50 values. Furthermore, compared to the NC group, CDK1i significantly lowered the EC50 value of KIF18Ai in HeLa (0.681 vs. 0.278 µM), SiHa (0.854 vs. 0.363 µM), RL95-2 (1.330 vs. 0.531 µM), and HEC-151 (1.936 vs. 0.639 µM) (Figure 5B and Table 1).
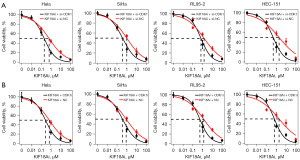
Table 1
Group | EC50 (μM) | Hill slope | P value |
---|---|---|---|
HeLa | <0.001 | ||
KIF18Ai + si-NC | 0.654 (0.564 to 0.744) | −0.514 (−0.481 to −0.547) | |
KIF18Ai + si-CDK1 | 0.206 (0.183 to 0.229) | −0.776 (−0.711 to −0.841) | |
SiHa | <0.001 | ||
KIF18Ai + si-NC | 0.985 (0.805 to 1.164) | −0.495 (−0.455 to −0.536) | |
KIF18Ai + si-CDK1 | 0.366 (0.308 to 0.423) | −0.654 (−0.586 to −0.722) | |
RL95-2 | <0.001 | ||
KIF18Ai + si-NC | 1.433 (1.181 to 1.685) | −0.482 (−0.445 to −0.519) | |
KIF18Ai + si-CDK1 | 0.681 (0.589 to 0.772) | −0.807 (−0.717 to −0.897) | |
HEC-151 | <0.001 | ||
KIF18Ai + si-NC | 1.943 (1.472 to 2.413) | −0.411 (−0.372 to −0.449) | |
KIF18Ai + si-CDK1 | 0.485 (0.402 to 0.569) | −0.774 (−0.668 to −0.879) | |
HeLa | <0.001 | ||
KIF18Ai + NC | 0.681 (0.575 to 0.787) | −0.526 (−0.487 to −0.565) | |
KIF18Ai + CDK1i | 0.278 (0.242 to 0.313) | −0.769 (−0.695 to −0.844) | |
SiHa | <0.001 | ||
KIF18Ai + NC | 0.854 (0.721 to 0.986) | −0.472 (−0.440 to −0.503) | |
KIF18Ai + CDK1i | 0.363 (0.315 to 0.410) | −0.644 (−0.593 to −0.696) | |
RL95-2 | <0.001 | ||
KIF18Ai + NC | 1.330 (1.103 to 1.557) | −0.481 (−0.445 to −0.517) | |
KIF18Ai + CDK1i | 0.531 (0.462 to 0.599) | −0.809 (−0.724 to −0.895) | |
HEC-151 | <0.001 | ||
KIF18Ai + NC | 1.936 (1.484 to 2.388) | −0.411 (−0.374 to −0.449) | |
KIF18Ai + CDK1i | 0.639 (0.535 to 0.742) | −0.830 (−0.712 to −0.945) |
EC50, median effect concentration; CESC, cervical squamous cell carcinoma and endocervical adenocarcinoma; UCEC, uterine corpus endometrial carcinoma; KIF18A, kinesin family member 18A; CDK1, cyclin-dependent kinase 1; KIF18Ai, kinesin family member 18A inhibitor; si-NC, small interfering RNA normal control; si-CDK1, small interfering RNA cyclin-dependent kinase 1; NC, normal control; CDK1i, cyclin-dependent kinase 1 inhibitor.
KIF18Ai and CDK1i act in synergy to inhibit migration and induce apoptosis
Following 48 h of co-incubation of CESC and UCEC cells with KIF18Ai (1 µM) and CDK1i (5 µM), the effects of these inhibitors on migration and apoptosis were assessed by transwell (Figure 6) and TUNEL (Figure 7) assays, respectively. The results indicated that individual administration of KIF18Ai or CDK1i significantly suppressed migration and induced apoptosis. Furthermore, the combined treatment of KIF18Ai and CDK1i demonstrated enhanced inhibition of migration and promotion of apoptosis compared to the CDK1i or KIF18Ai treatment alone.
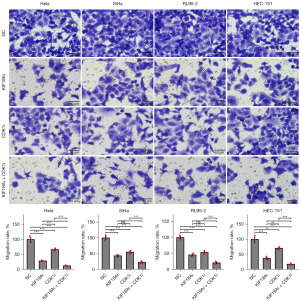
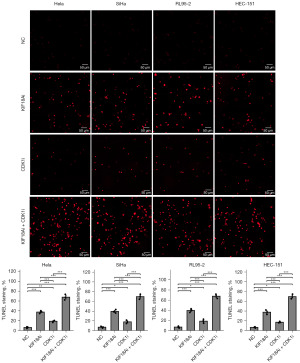
Discussion
Our findings indicated that the combination of CDK1is may potentially enhance the efficacy of KIF18Ais, given its close involvement in cell cycle and chromosome segregation. Bioinformatics analysis revealed a significant up-regulation of CDK1 expression in CESC and UCEC, which showed a strong correlation with KIF18A expression. CDK1 could be a viable combination therapeutic target for KIF18A, which was substantiated by cell experiments. CDK1 inhibition markedly increased the sensitivity of CESC and UCEC cells to KIF18Ais. The combined use of CDK1is and KIF18Ais exhibited a synergistic effect in inhibiting cell migration and inducing apoptosis in CESC and UCEC cells.
CDK1, a known substrate of E2F transcription factor 1, plays a role in the regulation of the G1 checkpoint. Knockdown of CDK1 impeded progression from G1 to S phase, whereas overexpression of CDK1 mitigated the effects of regulator of chromatin condensation 1 knockdown on G1 cell cycle progression in cervical cancer cells (33). Using bioinformatics prediction, both Vaghasia et al. (34) and Liu et al. (35) identified CDK1 as a hub gene and therapeutic target in CESC and UCEC. The increased expression of CDK1 is implicated in the development of resistance in endometrial cancer-associated fibroblasts to medroxyprogesterone acetate, indicating a potential impact of CDK1 on estrogen therapy outcomes in patients with UCEC in a clinical setting (36). Lin et al. (37) discovered that CDK1 exhibits elevated expression level in both UCEC cell lines and tissues, contributing to various cellular processes such as cell survival, proliferation, invasion, and migration, while also suppressing apoptosis and inducing DNA damage through phosphorylation of KIFC1. Furthermore, the CDK1-KIFC1 axis was shown to further stimulate the PI3K/AKT pathway to provoke tumor growth in vivo (37). These findings suggest that CDK1 may modulate the progression of UCEC through interactions with members of the KIF family. Our research indicated that KIF18A, another member of the KIF family, exhibits a synergistic anti-tumor effect with CDK1 via inhibiting cell proliferation and migration, and promoting cell apoptosis. Inhibition of CDK1 expression, via transfection of si-CDK1 or treatment with a small molecule inhibitor, increased the susceptibility of CESC and UCEC cells to KIF18Ais. Targeting both CDK1, a critical regulator of the G1/S cell cycle transition, and KIF18A, a gene involved in cell division and chromosome segregation, may represent a promising combined therapeutic approach for CESC and UCEC.
The small molecule inhibitor AMG-650, which targets KIF18, is currently undergoing clinical trials for the treatment of solid tumors. Previous preclinical studies have identified several candidate compounds targeting KIF18 with EC50 values below 0.05 µM (20,38). However, these compounds did not demonstrate a significant anti-proliferative effect in KIF18-insensitive cells (20,38). It has been proposed that CIN may be a prerequisite for sensitivity to KIF18 inhibitors (16,17,20,38). Therefore, inducing CIN could potentially enhance tumor sensitivity to KIF18 inhibitors. Drugs that induce CIN, combined with KIF18 inhibitors, could represent a novel therapeutic strategy for anti-tumor treatment. However, there is a lack of literature concerning the impact of KIF18Ais on CESC and UCEC cells. Our preliminary investigation revealed an EC50 value of 0.590–1.800 µM for the KIF18Ai on CESC and UCEC cells in vitro, which is notably higher than the value reported by Payton et al. (20). This discrepancy indicates that CESC and UCEC cells may require additional targets to augment the efficacy of the KIF18 inhibitor.
The majority of contemporary CDK1is are classified as pan-kinase inhibitors. For example, in the study conducted by Massard et al. (39), cohorts consisting of three to six patients received varying doses of PHA-793887 (11, 22, 44, and 66 mg/m2). PHA-793887 functions as an inhibitor of multiple cyclin-dependent kinases (CDKs), exhibiting activity against CDK2, CDK1, and CDK4. It was administered as a 1-h intravenous infusion on days 1, 8, and 15 within a 4-week cycle. However, PHA-793887 was found to induce severe, dose-dependent hepatic toxicity, a side effect not anticipated by preclinical models, which currently hinders its further clinical development. AZD5438 is an orally bioavailable inhibitor targeting cyclin E-CDK2, cyclin A-CDK2, and cyclin B-CDK1 complexes. While it is generally well tolerated when administered on weekly dosing schedules, it exhibits reduced tolerability on continuous dosing regimens. Consequently, based on the tolerability and exposure data obtained from this study, the clinical development program for AZD5438 was discontinued (40). Dinaciclib, a CDK1/CDK2is, in combination with epirubicin, is associated with significant toxicities and does not demonstrate efficacy as a treatment option for TNBC (41). The results presented above suggest that the administration of CDK1 multi-kinase inhibitors, whether individually or in combination, demonstrates a relatively high tissue toxicity. This may be attributed to the specific targeting mechanisms of CDK1is. Consequently, future research should focus on enhancing the specificity of CDK1is targeting to minimize tissue toxicity.
Our study suggests that KIF18A and CDK1 may be promising combined therapeutic targets for CESC and UCEC. However, our research has certain limitations. Firstly, we did not investigate the precise molecular mechanisms through which KIF18Ais and CDK1is impede the growth of CESC and UCEC cells. Secondly, we did not validate the efficacy of these inhibitors in suppressing tumor growth in vivo with animal models. We intend to pursue animal trials in future research to further corroborate our findings and aspire to collaborate with clinical research teams to undertake pertinent clinical validation. Thirdly, both KIF18A and CDK1 play critical roles in cell division, a process regulated by numerous genes, including various members of the CDK family. Fourthly, our current study is unable to determine whether the inhibition of CDK1 triggers feedback regulation. Finally, we validated the expression levels of KIF18A and CDK1 using data from a public database rather than our own cohort. In future studies, we plan to utilize clinical samples collected by our team to further verify the expression levels of KIF18A and CDK1.
Conclusions
The results of our study, which utilized bioinformatics analysis and in vitro experiments, suggest a novel therapeutic approach for CESC and UCEC by targeting CDK1 in conjunction with KIF18A. The combined inhibition of CDK1 and KIF18A demonstrates a synergistic effect in suppressing the growth and migration of CESC and UCEC cells, while also inducing apoptosis in tumor cells. Our finding provides a valuable contribution to the field of oncology by exploring the potential of targeting KIF18A and CDK1 in CESC and UCEC. The findings are robust, supported by both bioinformatics and in vitro experiments, and open up new possibilities for combination cancer therapies. However, further research is needed to confirm these results in vivo, explore underlying molecular mechanisms, and address clinical translation challenges. The study provides a promising foundation for future investigations into improving therapeutic strategies for CESC and UCEC but requires additional validation and refinement to ensure its applicability in clinical settings.
Acknowledgments
Funding: None.
Footnote
Reporting Checklist: The authors have completed the MDAR reporting checklist. Available at https://tcr.amegroups.com/article/view/10.21037/tcr-24-1831/rc
Data Sharing Statement: Available at https://tcr.amegroups.com/article/view/10.21037/tcr-24-1831/dss
Peer Review File: Available at https://tcr.amegroups.com/article/view/10.21037/tcr-24-1831/prf
Conflicts of Interest: All authors have completed the ICMJE uniform disclosure form (available at https://tcr.amegroups.com/article/view/10.21037/tcr-24-1831/coif). The authors have no conflicts of interest to declare.
Ethical Statement: The authors are accountable for all aspects of the work in ensuring that questions related to the accuracy or integrity of any part of the work are appropriately investigated and resolved. The study was conducted in accordance with the Declaration of Helsinki (as revised in 2013).
Open Access Statement: This is an Open Access article distributed in accordance with the Creative Commons Attribution-NonCommercial-NoDerivs 4.0 International License (CC BY-NC-ND 4.0), which permits the non-commercial replication and distribution of the article with the strict proviso that no changes or edits are made and the original work is properly cited (including links to both the formal publication through the relevant DOI and the license). See: https://creativecommons.org/licenses/by-nc-nd/4.0/.
References
- Zheng RS, Chen R, Han BF, et al. Cancer incidence and mortality in China, 2022. Zhonghua Zhong Liu Za Zhi 2024;46:221-31. [PubMed]
- Giannini A, D'Oria O, Corrado G, et al. The role of L1CAM as predictor of poor prognosis in stage I endometrial cancer: a systematic review and meta-analysis. Arch Gynecol Obstet 2024;309:789-99. [Crossref] [PubMed]
- Vizza E, Bruno V, Cutillo G, et al. Prognostic Role of the Removed Vaginal Cuff and Its Correlation with L1CAM in Low-Risk Endometrial Adenocarcinoma. Cancers (Basel) 2021;14:34. [Crossref] [PubMed]
- Cohen PA, Jhingran A, Oaknin A, et al. Cervical cancer. Lancet 2019;393:169-82. [Crossref] [PubMed]
- Crosbie EJ, Kitson SJ, McAlpine JN, et al. Endometrial cancer. Lancet 2022;399:1412-28. [Crossref] [PubMed]
- Eskander RN, Sill MW, Beffa L, et al. Pembrolizumab plus Chemotherapy in Advanced Endometrial Cancer. N Engl J Med 2023;388:2159-70. [Crossref] [PubMed]
- Meric-Bernstam F, Makker V, Oaknin A, et al. Efficacy and Safety of Trastuzumab Deruxtecan in Patients With HER2-Expressing Solid Tumors: Primary Results From the DESTINY-PanTumor02 Phase II Trial. J Clin Oncol 2024;42:47-58. [Crossref] [PubMed]
- Tewari KS, Monk BJ, Vergote I, et al. Survival with Cemiplimab in Recurrent Cervical Cancer. N Engl J Med 2022;386:544-55. [Crossref] [PubMed]
- Barra F, Lorusso D, Leone Roberti Maggiore U, et al. Investigational drugs for the treatment of cervical cancer. Expert Opin Investig Drugs 2017;26:389-402. [Crossref] [PubMed]
- Liu T, Yang K, Chen J, et al. Comprehensive Pan-Cancer Analysis of KIF18A as a Marker for Prognosis and Immunity. Biomolecules 2023;13:326. [Crossref] [PubMed]
- Ren J, Yao X, Yang M, et al. Kinesin Family Member-18A (KIF18A) Promotes Cell Proliferation and Metastasis in Hepatocellular Carcinoma. Dig Dis Sci 2024;69:1274-86. [Crossref] [PubMed]
- Hemida AS, Shabaan MI, Taha MA, et al. Impact of immunohistochemical expression of kinesin family member 18A (Kif18A) and β-catenin in infiltrating breast carcinoma of no special type. World J Surg Oncol 2024;22:15. [Crossref] [PubMed]
- Tao BY, Liu YY, Liu HY, et al. Prognostic Biomarker KIF18A and Its Correlations With Immune Infiltrates and Mitosis in Glioma. Front Genet 2022;13:852049. [Crossref] [PubMed]
- Cai F, Li J, Zhang J, et al. Knockdown of Circ_CCNB2 Sensitizes Prostate Cancer to Radiation Through Repressing Autophagy by the miR-30b-5p/KIF18A Axis. Cancer Biother Radiopharm 2022;37:480-93. [Crossref] [PubMed]
- Cho SY, Kim S, Kim G, et al. Integrative analysis of KIF4A, 9, 18A, and 23 and their clinical significance in low-grade glioma and glioblastoma. Sci Rep 2019;9:4599. [Crossref] [PubMed]
- Janssen LME, Averink TV, Blomen VA, et al. Loss of Kif18A Results in Spindle Assembly Checkpoint Activation at Microtubule-Attached Kinetochores. Curr Biol 2018;28:2685-2696.e4. [Crossref] [PubMed]
- Marquis C, Fonseca CL, Queen KA, et al. Chromosomally unstable tumor cells specifically require KIF18A for proliferation. Nat Commun 2021;12:1213. [Crossref] [PubMed]
- Drews RM, Hernando B, Tarabichi M, et al. A pan-cancer compendium of chromosomal instability. Nature 2022;606:976-83. [Crossref] [PubMed]
- Castellanos G, Valbuena DS, Pérez E, et al. Chromosomal Instability as Enabling Feature and Central Hallmark of Breast Cancer. Breast Cancer (Dove Med Press) 2023;15:189-211. [Crossref] [PubMed]
- Payton M, Belmontes B, Hanestad K, et al. Small-molecule inhibition of kinesin KIF18A reveals a mitotic vulnerability enriched in chromosomally unstable cancers. Nat Cancer 2024;5:66-84. [Crossref] [PubMed]
- Wang Q, Bode AM, Zhang T. Targeting CDK1 in cancer: mechanisms and implications. NPJ Precis Oncol 2023;7:58. [Crossref] [PubMed]
- Schmidt AK, Pudelko K, Boekenkamp JE, et al. The p53/p73 - p21(CIP1) tumor suppressor axis guards against chromosomal instability by restraining CDK1 in human cancer cells. Oncogene 2021;40:436-51. [Crossref] [PubMed]
- Gao YF, Li T, Chang Y, et al. Cdk1-phosphorylated CUEDC2 promotes spindle checkpoint inactivation and chromosomal instability. Nat Cell Biol 2011;13:924-33. [Crossref] [PubMed]
- Malumbres M, Barbacid M. Cell cycle, CDKs and cancer: a changing paradigm. Nat Rev Cancer 2009;9:153-66. [Crossref] [PubMed]
- Joo YK, Black EM, Trier I, et al. ATR promotes clearance of damaged DNA and damaged cells by rupturing micronuclei. Mol Cell 2023;83:3642-3658.e4. [Crossref] [PubMed]
- Song X, Conti D, Shrestha RL, et al. Counteraction between Astrin-PP1 and Cyclin-B-CDK1 pathways protects chromosome-microtubule attachments independent of biorientation. Nat Commun 2021;12:7010. [Crossref] [PubMed]
- Ghandi M, Huang FW, Jané-Valbuena J, et al. Next-generation characterization of the Cancer Cell Line Encyclopedia. Nature 2019;569:503-8. [Crossref] [PubMed]
- Love MI, Huber W, Anders S. Moderated estimation of fold change and dispersion for RNA-seq data with DESeq2. Genome Biol 2014;15:550. [Crossref] [PubMed]
- Robinson MD, McCarthy DJ, Smyth GK. edgeR: a Bioconductor package for differential expression analysis of digital gene expression data. Bioinformatics 2010;26:139-40. [Crossref] [PubMed]
- Clarke DJB, Kuleshov MV, Schilder BM, et al. eXpression2Kinases (X2K) Web: linking expression signatures to upstream cell signaling networks. Nucleic Acids Res 2018;46:W171-9. [Crossref] [PubMed]
- Dang F, Nie L, Wei W. Ubiquitin signaling in cell cycle control and tumorigenesis. Cell Death Differ 2021;28:427-38. [Crossref] [PubMed]
- Fischer M, Schade AE, Branigan TB, et al. Coordinating gene expression during the cell cycle. Trends Biochem Sci 2022;47:1009-22. [Crossref] [PubMed]
- Qiao L, Zheng J, Tian Y, et al. Regulator of chromatin condensation 1 abrogates the G1 cell cycle checkpoint via Cdk1 in human papillomavirus E7-expressing epithelium and cervical cancer cells. Cell Death Dis 2018;9:583. [Crossref] [PubMed]
- Vaghasia H, Sakaria S, Prajapati J, et al. Interactive bioinformatics analysis for the screening of hub genes and molecular docking of phytochemicals present in kitchen spices to inhibit CDK1 in cervical cancer. Comput Biol Med 2022;149:105994. [Crossref] [PubMed]
- Liu Y, Yi Y, Wu W, et al. Bioinformatics prediction and analysis of hub genes and pathways of three types of gynecological cancer. Oncol Lett 2019;18:617-28. [Crossref] [PubMed]
- Omar IS, Mat Adenan NA, Godoy A, et al. Aberrant upregulation of CDK1 contributes to medroxyprogesterone acetate (MPA) resistance in cancer-associated fibroblasts of the endometrium. Biochem Biophys Res Commun 2022;628:133-40. [Crossref] [PubMed]
- Lin X, He Y, Liu Y, et al. CDK1 promotes the phosphorylation of KIFC1 to regulate the tumorgenicity of endometrial carcinoma. J Gynecol Oncol 2024;35:e68. [Crossref] [PubMed]
- Tamayo NA, Bourbeau MP, Allen JR, et al. Targeting the Mitotic Kinesin KIF18A in Chromosomally Unstable Cancers: Hit Optimization Toward an In Vivo Chemical Probe. J Med Chem 2022;65:4972-90. [Crossref] [PubMed]
- Massard C, Soria JC, Anthoney DA, et al. A first in man, phase I dose-escalation study of PHA-793887, an inhibitor of multiple cyclin-dependent kinases (CDK2, 1 and 4) reveals unexpected hepatotoxicity in patients with solid tumors. Cell Cycle 2011;10:963-70. [Crossref] [PubMed]
- Boss DS, Schwartz GK, Middleton MR, et al. Safety, tolerability, pharmacokinetics and pharmacodynamics of the oral cyclin-dependent kinase inhibitor AZD5438 when administered at intermittent and continuous dosing schedules in patients with advanced solid tumours. Ann Oncol 2010;21:884-94. [Crossref] [PubMed]
- Mitri Z, Karakas C, Wei C, et al. A phase 1 study with dose expansion of the CDK inhibitor dinaciclib (SCH 727965) in combination with epirubicin in patients with metastatic triple negative breast cancer. Invest New Drugs 2015;33:890-4. [Crossref] [PubMed]
(English Language Editor: J. Jones)