Lycorine affects tamoxifen resistance of breast cancer via m6A-based HAGLR
Highlight box
Key findings
• Lycorine inhibits tamoxifen-resistant breast cancer by repressing IGF2BP2-mediated N6-methyladenosine (m6A) methylation of HAGLR, thereby reducing tamoxifen resistance.
What is known and what is new?
• Tamoxifen resistance is a major challenge in breast cancer treatment. Long non-coding RNAs (lncRNAs) and m6A modification play roles in cancer drug resistance. IGF2BP2 functions as an m6A reader, affecting lncRNA stability.
• Total m6A level of HAGLR is elevated in tamoxifen-resistant breast cancer cells. Only IGF2BP2 accounts for the upregulation and stability of HAGLR through an m6A manner. Lycorine weakens the IGF2BP2/HAGLR interaction and expression to act against tamoxifen resistance of breast cancer.
What is the implication, and what should change now?
• The IGF2BP2/HAGLR/m6A axis represents a potential therapeutic target for tamoxifen-resistant breast cancer.
• Lycorine should be further investigated as a promising agent to combat tamoxifen resistance.
• Future research should focus on in vivo validation and clinical trials to assess the efficacy of targeting this pathway in tamoxifen-resistant breast cancer patients.
Introduction
The incidence of breast cancer (BC), a prevalent malignancy among women, has increased notably worldwide in recent decades (1,2). Ranking sixth among cancer-related causes of mortality, BC represents a major public health burden in China today (3,4). Endocrine treatment has become a standard therapeutic option for BC patients since around 70% BC cases are hormone-responsive (5,6). As a selective estrogen receptor (ER) regulator, tamoxifen can block the binding of estrogen and its cognate receptor, thus being frequently used for the treatment of ER-positive BC patients, especially for those in pre-menopause (7). A 5-year adjuvant treatment of tamoxifen markedly decreases the fifteen-year risk of recurrence and death in BC patients (7). While the initial tamoxifen response in BC patients is satisfactory, the majority BC patients eventually develop resistance (8). Unfortunately, tamoxifen-resistant BC cells are more prone to metastasis and invasion, making BC patients’ 5-year survival rate drop from 80% to 27% with the occurrence of metastasis (9). Elucidating the molecular pathways driving tamoxifen resistance in BC is of great importance to address this clinical challenge.
The implication of long non-coding RNAs (lncRNAs) in BC has been well documented by numerous of research over the past decades (10). Evidence has shown that lncRNAs could be used not only as biomarkers for BC, but also as promising therapeutic targets for BC (11). Not surprisingly, they have also been found to take essential actions on affecting tamoxifen resistance of BC through complex regulatory networks. For instance, lncRNA DILA1 is elevated in tamoxifen-resistant BC and contributes to tamoxifen resistance by inhibiting the degradation of cyclin D1 (12). LncRNA HOTAIRM1 was revealed to enhance tamoxifen resistance of ER positive BC by inducing the expression of HOXA1 (13). A recent study of our laboratory validated that lycorine treatment significantly reversed tamoxifen resistance of BC cell via relieving HOXD antisense growth-associated lncRNA (HAGLR)-mediated epigenetic suppression of VGLL4 (14). Although we have already identified a novel link between lycorine and HAGLR involving in tamoxifen resistance of BC, the precise modulation among them remains elusive. Therefore, better understanding the regulatory mechanism of lycorine on HAGLR will certainly contribute to developing a way to enhance the role of lycorine in alleviating tamoxifen resistance of BC.
N6-methyladenosine (m6A), a prevalent internal modification of mammalian RNAs, is pivotal in orchestrating diverse physiological and pathological processes through modulating gene expression (15). In addition, the m6A-mediated epitranscriptomic pathway has been shown to contribute to chemoresistance and radioresistance. Within the past decade, m6A modification has been widely demonstrated to be involved in the regulation of the expression of lncRNAs, and thus affecting the pathogenesis of human cancers (16). IGF2BP2 functions as a m6A recognition factor, binding to target transcripts in an m6A-dependent manner and mediating downstream regulatory effects (17). It is suggested that downstream lncRNAs could be identified by IGF2BP2, thereby altering the stability of their targeted lncRNAs in an m6A-dependent way (18). Interestingly, further investigation revealed that IGF2BP2 modulates the expression of HAGLR in thyroid cancer by recognizing m6A modifications in a sequence-specific fashion (19). However, whether IGF2BP2 would be a key intermediate factor for linking lycorine and HAGLR to participant in tamoxifen resistance of BC remains undetermined.
Herein, in this text, we mainly focus on uncovering the exact regulation of lycorine on HAGLR expression in tamoxifen resistance of BC. Our findings found that lycorine treatment could decrease IGF2BP2 level in tamoxifen-resistant BC, thereby reducing its downstream target, HAGLR, which ultimately alleviated tamoxifen resistance of BC. We present this article in accordance with the MDAR reporting checklist (available at https://tcr.amegroups.com/article/view/10.21037/tcr-24-1077/rc).
Methods
Cell lines
MCF7, T47D and MCF10A cell lines were sourced from the American Type Cell Collection (Manassas, VA, USA). Cells were all kept under 37 ℃ in RPMI culture medium (Sigma-Aldrich, St Louis, MO, USA) containing 10% fetal bovine serum. Tamoxifen-resistant sublines (MCF7/TAMR and T47D/TAMR) were generated by exposure to stepwise increases in the concentration of tamoxifen for six months, with fresh medium replacement every 3 days. As referring to the administration of lycorine (Selleck, Houston, TX, USA), the concentration with 5 µM was applied for incubating 24 h.
m6A distribution prediction
Prediction of m6A distribution across HAGLR sequence was determined by an online software, SRAMP (20).
m6A methylated RNA immunoprecipitation (MeRIP) assay
RNA of indicated cells was isolated by use of a RNeasy-Mini-Kit (Qiagen, Germantown, MD, USA). Isolated RNAs were then fragmented by zinc buffer (10 mM ZnCl2 and Tris-HCl, pH 7.0). Incubated the mixed buffer under 95 ℃ for 5 min and the reaction was inactivated by ethylenediaminetetraacetic acid (EDTA; 50 mM). The fragment RNAs were precipitated by ethanol, and 50 µg of which was added into 500 µL binding buffer containing RNase inhibitor (300 U) and Dynabeads (Thermo Fisher Scientific, Waltham, MA, USA) crosslinked with anti-m6A antibody (ab99080, Abcam, Cambridge, UK) or immunoglobulin G (IgG). After 1 h of incubation, MeRIPs were rinsed by binding buffer, and the RNA was eluted using elution buffer and then detected by quantitative real-time polymerase chain reaction (qRT-PCR). Four pairs of primer were designed for each m6A site.
qRT-PCR analysis
RNA of indicated cells was isolated as depicted above. Reverse transcription of isolated RNA into cDNA was undertaken using the PrimeScript RT kit (Takara, Kusatsu, Shiga, Japan). The resulting cDNA was amplified by qRT-PCR on the ABI 7500 platform (Applied Biosystems, Foster City, CA, USA), employing the YBR Green fluorescent detection system (Roche, Indianapolis, IN, USA). Oligonucleotide primers utilized for targeted gene amplification are enumerated below.
HAGLR: (F: 5'-TGA TCC TGG CCT CCT GGT AT-3', R: 5'-AGG CAG GAA CCA CCA TGT CT-3');
IGF2BP2: (F: 5'-AGG CCA GAC AGA TTG ATT TCC-3', R: 5'-CGG GAC TGG GTC TGC TTA G-3');
GAPDH: (F: 5'-GAGTCCTTCCACGATACCAA-3', Reverse: 5'-ACG TCG CAC TTC ATG ATC GAG-3').
Plasmid construction and transfection
sh-IGF2BP2 was achieved by inserting IGF2BP2 segment into pGLVH1 vector (GenePharma, Shanghai, China). HEK293T cells were transfected with the re-constructed lentiviral vector, and target cells were picked up using puromycin (2 µg/mL). Specific siRNAs of HAGLR were synthesized and provided by GenePharma. For IGF2BP2 overexpression, IGF2BP2 cDNA sequence was sub-cloned into a pcDNA3.1 vector (GenePharma). Transfection process was completed via Lipofectamine 3000 (Invitrogen, Carlsbad, CA, USA) as per the provided protocol.
RNA immunoprecipitation (RIP) assay
Interplay within IGF2BP2 and HAGLR was tested by RIP assay using Magna-RIP Kit (Millipore, Billerica, MA, USA) as per the provided protocol. Treated TAMR BC cells were lysed in the RIP buffer provided in the Kit (Millipore). Then, the lysis was subjected to incubation with anti-IGF2BP2 (1:1000, Cell Signaling Tech, Danvers, MA, USA) or IgG (1:1,000, Cell Signaling Tech)-coated buffer containing beads for 8 h. Next, the RNA-protein complex was then eluted and detected by qRT-PCR analysis.
Immunofluorescence staining and RNA-fluorescence in-situ hybridization (FISH)
To examine the cellular co-localization of lncRNA HAGLR and IGF2BP2 protein in BC cells, FISH and immunofluorescence assays were simultaneously performed. BC cells adherent to glass coverslips underwent chemical fixation in 4% paraformaldehyde followed by membrane permeabilization via incubation with 0.5% Triton X-100 detergent. Cells were then incubated simultaneously with a fluorescein-labeled HAGLR antisense probe (GenePharma) and a rabbit anti-IGF2BP2 antibody (1:200, ab124930, Abcam) at 4 ℃ overnight. After washing off unbound probes and antibodies, cells were incubated with an Alexa Fluor 594/488-conjugated goat anti-rabbit secondary antibody (1:500, ab150080/ab150077, Abcam) for 2 h at room temperature in the dark. Subsequently, cells were counterstained with 4',6-diamidino-2-phenylindole (DAPI) and imaged under a fluorescence microscope. The cellular distribution and co-localization of green fluorescent lncRNA HAGLR and red fluorescent IGF2BP2 protein were analyzed using ImageJ software (version 1.54f).
Actinomycin D (Act D) assay
Act D assay was performed to evaluate the regulation of IGF2BP2 on HAGLR stability. Treated BC cells were incubated with 2 µg/mL Act D (Sigma-Aldrich) for another different indicated time points. Subsequently, cells were harvested for the detection of qRT-PCR analysis.
Western blot analysis
Proteins were isolated after lysis using RIPA buffer (Thermo Fisher Scientific), and 50 µg of which was loaded into sodium dodecyl sulfate-polyacrylamide gel electrophoresis (SDS-PAGE) for isolation. The isolated protein bands were then transferred to polyvinylidene fluoride membranes (Millipore) and blocked with low-fat milk (5%, 2 h). Immunoblotting was performed by overnight incubation with primary antibodies recognizing IGF2BP2 (1:2,000, ab124930, Abcam). After incubation with secondary antibody, the signals were determined using Odyssey infrared imaging system (LI-COR Inc., Lincoln, NE, USA) and band was analyzed by ImageJ.
Cell viability and proliferation assessment
A Cell Counting Kit-8 (CCK-8) assay kit (Dojindo, Kumamoto, Japan) was utilized in cell viability assessment. In brief, treated BC cells were plated in 96-well plates at a density of 1×105 cells/mL in 100 µL of DMEM per well. A 10 µL aliquot of CCK8 reagent was added into each well and allowed for 2 h incubation. Absorbance was measured spectrophotometrically at 450 nm by a BioRad (USA), microplate reader. Colony formation assay was adopted for cell proliferation assessment. In brief, treated BC cells were harvested and diluted to 1,000 cells per well in 6-well plates containing 1.5 mL of culture medium. Following 14 days of incubation, resultant colonies fixed with 4% paraformaldehyde (Sigma, St. Louis, MO, USA), stained by crystal violet (Beyotime, Shanghai, China) and manually enumerated under a microscope.
Cell apoptosis assessment
Treated BC cells were collected and fixed overnight in 70% pre-cold ethanol. Following three times of washes with phosphate-buffered saline (PBS), cells were incubated with propidium/Annexin V-FITC (Sigma-Aldrich) for 20 min. A flow cytometer (Gallios, Beckman, CA, USA) was then employed to assess apoptosis rate.
2,5-diphenyl-2H-tetrazolium bromide (MTT) assay
Treated BC cells were collected and plated in 96-well plates with a concentration of 1×105 cells/mL. After 24 h of culture, MTT reagent (10 µL, Vybrant® MTT Cell Proliferation Assay Kit, Thermo Fisher Scientific, Carlsbad, CA, USA) was added to each well and allowed for another 4 h incubation. Next, the supernatant was removed, and the reaction was ended by dimethyl sulfoxide (DMSO; 200 µL, 20 min). Absorbency was detected at 490 nm.
Statistical analysis
Data were presented as mean ± standard deviation from at least three independent experiments. Statistical analysis was performed using GraphPad Prism 7.0 (GraphPad Software, La Jolla, CA, USA). Differences between two groups were analyzed by Student’s t-test, while one-way analysis of variance (ANOVA) was used for comparisons of multiple groups, followed by appropriate post-hoc tests. P values less than 0.05 were considered statistically significant.
Results
Increasing m6A modification status of HAGLR is observed in TAMR BC cells
On the basis of our previous study mentioned above, we next sought to further explore the underlying mechanism for HAGLR upregulation in BC. Firstly, the full sequence of HAGLR was downloaded from National Center for Biotechnology Information (NCBI) and subjected for sequence-based RNA adenosine methylation site predictor (SRAMP) analysis. The SRAMP database presented four specific m6A modification loci in HAGLR sequence with relative high confidence (Figure 1A,1B), consistent with the MeRIP-qPCR assay (Figure 1C). Afterwards, MeRIP-qPCR assay was also performed to determine the m6A modification level of total RNAs. As illustrated in Figure 1D, the results indicated that the m6A modification level was higher in MCF7 and T47D cells than that in MCF10A cells. Moreover, the m6A modification level was further increased in MCF7/TAMR, and T47D/TAMR cells compared to MCF7 and T47D, respectively (Figure 1D), suggesting that m6A modification might be involved in the TAM resistance of BC cells.
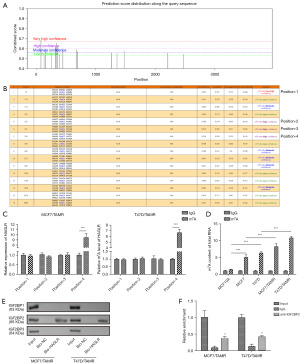
As well documented m6A readers, IGF2BPs, have been proved to control the stability of target mRNA, it is largely unknown whether and how IGF2BPs affect HAGLR stability in the development of BC. Subsequently, interaction between HAGLR and IGF2BP1, IGF2BP2, or IGF2BP3 was tested using RNA pull-down assay through a biotin-labeled HAGLR. As results indicated that only IGF2BP2 was enriched in biotin-labeled HAGLR group (Figure 1E). Results from RIP assay also indicated that HAGLR was enriched in anti-IGF2BP2 group compared to IgG group (Figure 1F).
IGF2BP2-mediated m6A RNA methylation drives the upregulation and stability of HAGLR in TAMR BC cells
Since it remains largely unknown whether and how IGF2BP2 participates in lycorine-mediated suppression on tamoxifen resistance of BC, to answer this question, we firstly examined IGF2BP2 expression levels in MCF7/TAMR, and T47D/TAMR cells with or without lycorine treatment. As presented in Figure 2A, lycorine treatment significantly decreased IGF2BP2 level. What’s more, the data of Figure 2B also validated that in comparison of MCF10A cells, IGF2BP2 was elevated in MCF7 and T47D cells, and it was further increased in MCF7/TAMR, and T47D/TAMR cells. We next utilized specific shRNA targeting IGF2BP2 (sh-IGF2BP2) to knockdown its expression, and qRT-PCR analysis determined a significant downregulation of IGF2BP2 in MCF7/TAMR and T47D/TAMR cells treated with sh-IGF2BP2 (Figure 2C). Similarly, IGF2BP2 depletion also induced a decline of HAGLR in MCF7/TAMR and T47D/TAMR cells (Figure 2D). Likewise, the transfection efficiency of IGF2BP2 overexpression plasmid was further validated by using qRT-PCR analysis, as illustrated in Figure 2E. It was also found that an obvious upregulation of HAGLR level in MCF7/TAMR and T47D/TAMR cells treated with IGF2BP2 overexpression plasmid (Figure 2F). These results suggested that IGF2BP2 could positively regulate the expression of HAGLR in TAMR BC cells. Moreover, the impacts of IGF2BP2 knockdown on the m6A modification level of HAGLR were assessed by MeRIP. Knockdown of IGF2BP2 significantly reduced the m6A modification of HAGLR in MCF7/TAMR and T47D/TAMR cells (Figure 2G). In addition, the degradation rate of HAGLR was found to be facilitated by IGF2BP2 knockdown (Figure 2H). On the contrary, overexpression of IGF2BP2 in MCF7/TAMR and T47D/TAMR cells not only increased the m6A modification of HAGLR (Figure 2I), but also slowed down the degradation rate of HAGLR (Figure 2J). These findings suggested that IGF2BP2 could positively regulate HAGLR expression via an m6A manner in TAMR BC cells.
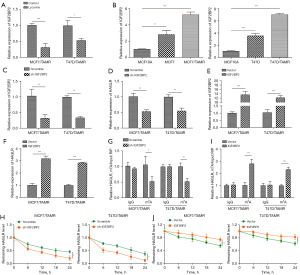
Lycorine disrupts the interaction between HAGLR and IGF2BP2
Considering the inhibitory effects of lycorine on HARGLR and the interaction between HARGLR and IGF2BP2, we then tested whether lycorine could affect the interplay of HARGLR and IGF2BP2 in TAMR BC cells. MCF7/TAMR cells were treated with lycorine for 24 h followed by the detection of HARGLR and IGF2BP2 using RNA FISH (for HARGLR) and immunofluorescence (for IGF2BP2). As results indicated that the fluorescence intensities of HARGLR and IGF2BP2 were dramatically attenuated after lycorine treatment compared to control group (Figure 3). These results indicated that lycorine treatment weakened the interaction between HARGLR and IGF2BP2 in TAMR BC cells.
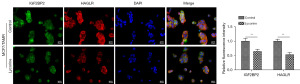
IGF2BP2 overexpression blocks the inhibitory effects of lycorine on TAMR BC cells
To investigate whether IGF2BP2 plays a role in the tamoxifen resistance of BC cells, MCF7/TAMR and T47D/TAMR cells were treated with IGF2BP2 overexpression plasmid in the presence of lycorine. Lycorine treatment significantly decreased the mRNA and protein expression of IGF2BP2, while IGF2BP2 overexpression reversed the downregulation of IGF2BP2 (Figure 4A,4B). In CCK-8 and colony formation assays, we found that lycorine treatment reduced the cell viability and proliferation of MCF7/TAMR and T47D/TAMR cells, however, the lycorine-induced inhibition on cell viability and proliferation was reversed by IGF2BP2 overexpression (Figure 4C,4D). Analysis of cell apoptosis suggested that lycorine treatment significantly increased the apoptosis rate of MCF7/TAMR, and T47D/TAMR cells, while IGF2BP2 overexpression could block this phenomenon (Figure 4E). These results indicated that IGF2BP2 overexpression could reverse the inhibitory effects of lycorine on tamoxifen resistance of BC cells.
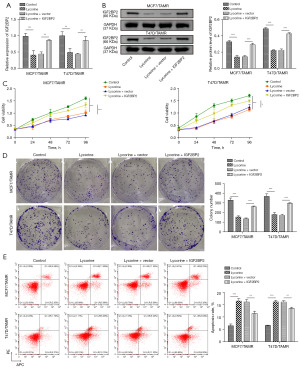
Effects of IGF2BP2 or HAGLR knockdown in TAMR BC cells
As demonstrated before, lycorine treatment significantly decreased the expression level of HAGLR, while IGF2BP2 overexpression reversed the downregulation of HAGLR caused by lycorine treatment (Figure 5A). In cell proliferation activity assessment, we found that the protective effects of IGF2BP2 overexpression on cell proliferation activity in the presence of lycorine were blocked by HAGLR knockdown (Figure 5B,5C). Next, the IC50 value of MCF7, T47D, MCF7/TAMR, and T47D/TAMR cells to tamoxifen was determined by MTT assay after treatment with sh-IGF2BP2 or si-HAGLR. As results indicated the IC50 values of MCF7/TAMR and T47D/TAMR cells to tamoxifen were higher than their parental cells (Figure 5D,5E). Moreover, we found that the IC50 values of MCF7/TAMR and T47D/TAMR cells were reduced by the transfection with sh-IGF2BP2 or si-HAGLR (Figure 5D,5E). These findings suggested that IGF2BP2 or HAGLR knockdown could reduce the IC50 value of MCF7/TAMR and T47D/TAMR cells to tamoxifen.
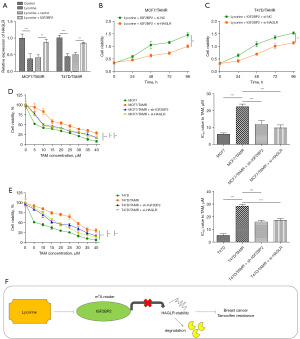
Discussion
In this study, we found that the total m6A was increased in tamoxifen-resistant BC cells, we also found the presence of m6A modification on HAGLR sequence. IGF2BP2 was found to be significantly increased in tamoxifen-resistant BC cells, of which m6A modification was crucial for maintaining the upregulation and stability of HAGLR. Moreover, reinforced IGF2BP2 abrogated the inhibitory effects of lycorine on tamoxifen-resistant BC cells. Knockdown of IGF2BP2 reduced the IC50 value of tamoxifen-resistant BC cells to tamoxifen. In conclusion, our results indicated that lycorine treatment could decrease the expression of IGF2BP2 in tamoxifen-resistant BC cells, thereby reducing the m6A level on HAGLR and ultimately alleviating tamoxifen resistance of BC (Figure 5F).
Increasing studies suggest that m6A modification is closely linked with tumor proliferation, invasion, metastasis, and differentiation and serves as either an inhibitor or oncogene in BC (21,22). As a key “reader” of m6A modification, IGF2BP2 has been found to be overexpressed in BC tissues and cells by numerous of research (23,24). Silencing of IGF2BP2 was demonstrated to decrease metastatic endothelial density and therefore repressed lung metastasis of BC cells (25). Overexpression of IGF2BP2 enhances cell migration and decreases cell adhesion through controlling connective tissue growth factor (CTGF) mRNA and induces BC cell proliferation and differentiation (26). Consistently, we found that IGF2BP2 was elevated in BC, and it was further increased in tamoxifen-resistant BC cells. Our previous study suggested that lycorine treatment could increase the sensitivity of BC cells to tamoxifen (14), herein, we found that lycorine decreased the expression of IGF2BP2 in BC cells, and IGF2BP2 overexpression could block the effects of lycorine on tamoxifen-resistant BC cells. These results suggested that IGF2BP2 is involved in the tamoxifen resistance of BC cells.
As we all know that the m6A modification was mainly found on mRNA in eukaryotes, while the most of the m6A regulators, such as METTL3, IGF2BPs, and ALKBH5, also have the ability to write m6A modification on non-coding RNAs, including microRNAs, lncRNAs, and circular RNAs (27,28). Meanwhile, ncRNAs can regulate the expression of m6A regulators by targeting to them (27). The interaction within ncRNAs and m6A modification affects multiple biological events of tumor cells. LINC00460 was reported to increase the expression of HMGA1 by regulating IGF2BP2 in colorectal cancer (29). Moreover, IGF2BP2 knockdown was found to reduce the m6A level on HAGLR in thyroid cancer, thus attenuating the stability of HAGLR and suppressing the progression of thyroid cancer (19). Herein, for the first time, we reported that IGF2BP2 could stabilize the expression of HAGLR in BC by increasing its m6A level in BC.
Limitation
Although our study revealed the crucial role of IGF2BP2-mediated m6A methylation of HAGLR in tamoxifen resistance in BC, there are some limitations. Firstly, the sample size of cell lines was relatively small. More tamoxifen-resistant and parental cell lines need to be tested to support the conclusion. Secondly, the in vivo role of IGF2BP2 and HAGLR was not determined using animal models, which should be examined before clinical translation. Thirdly, the detailed mechanisms of how m6A methylation of HAGLR regulates tamoxifen resistance remains unclear. Fourthly, whether targeting this pathway can benefit tamoxifen-resistant BC patients needs clinical investigation. In the future, we will expand the sample size, establish tamoxifen-resistant xenograft models to verify the role of IGF2BP2/HAGLR/m6A axis in vivo, elucidate the downstream signaling pathways, and conduct clinical trials to assess the therapeutic potential of targeting this newly identified driving pathway for tamoxifen-resistant BC patients. We believe further mechanistic and translational studies will promote it to be a promising therapy for the clinical management of tamoxifen resistance.
Conclusions
Our findings suggested that lycorine inhibited the expression of IGF2BP2 in tamoxifen-resistant BC cells, thereby reducing the m6A level on HAGLR and ultimately alleviating tamoxifen resistance of BC. Our study revealed the molecular mechanism of lycorine in regulating BC tamoxifen resistance and provided a new target for alleviating BC tamoxifen resistance.
Acknowledgments
Funding: None.
Footnote
Reporting Checklist: The authors have completed the MDAR reporting checklist. Available at https://tcr.amegroups.com/article/view/10.21037/tcr-24-1077/rc
Data Sharing Statement: Available at https://tcr.amegroups.com/article/view/10.21037/tcr-24-1077/dss
Peer Review File: Available at https://tcr.amegroups.com/article/view/10.21037/tcr-24-1077/prf
Conflicts of Interest: All authors have completed the ICMJE uniform disclosure form (available at https://tcr.amegroups.com/article/view/10.21037/tcr-24-1077/coif). The authors have no conflicts of interest to declare.
Ethical Statement: The authors are accountable for all aspects of the work in ensuring that questions related to the accuracy or integrity of any part of the work are appropriately investigated and resolved.
Open Access Statement: This is an Open Access article distributed in accordance with the Creative Commons Attribution-NonCommercial-NoDerivs 4.0 International License (CC BY-NC-ND 4.0), which permits the non-commercial replication and distribution of the article with the strict proviso that no changes or edits are made and the original work is properly cited (including links to both the formal publication through the relevant DOI and the license). See: https://creativecommons.org/licenses/by-nc-nd/4.0/.
References
- Michaels E, Worthington RO, Rusiecki J. Breast Cancer: Risk Assessment, Screening, and Primary Prevention. Med Clin North Am 2023;107:271-84. [Crossref] [PubMed]
- Swaminathan H, Saravanamurali K, Yadav SA. Extensive review on breast cancer its etiology, progression, prognostic markers, and treatment. Med Oncol 2023;40:238. [Crossref] [PubMed]
- Tao X, Li T, Gandomkar Z, et al. Incidence, mortality, survival, and disease burden of breast cancer in China compared to other developed countries. Asia Pac J Clin Oncol 2023;19:645-54. [Crossref] [PubMed]
- Wang X, O'Regan RM. Breast cancer therapy in China: Introducing the Special Collection. Cancer 2024;130:1368-70. [Crossref] [PubMed]
- Reinbolt RE, Mangini N, Hill JL, et al. Endocrine therapy in breast cancer: the neoadjuvant, adjuvant, and metastatic approach. Semin Oncol Nurs 2015;31:146-55. [Crossref] [PubMed]
- Ziyeh S, Wong L, Basho RK. Advances in Endocrine Therapy for Hormone Receptor-Positive Advanced Breast Cancer. Curr Oncol Rep 2023;25:689-98. [Crossref] [PubMed]
- Chan CWH, Law BMH, So WKW, et al. Pharmacogenomics of breast cancer: highlighting CYP2D6 and tamoxifen. J Cancer Res Clin Oncol 2020;146:1395-404. [Crossref] [PubMed]
- Irarrázaval O ME, Gaete G L. Antidepressants agents in breast cancer patients using tamoxifen: review of basic and clinical evidence. Rev Med Chil 2016;144:1326-35. [PubMed]
- Kahn KL, Schneider EC, Malin JL, et al. Patient centered experiences in breast cancer: predicting long-term adherence to tamoxifen use. Med Care 2007;45:431-9. [Crossref] [PubMed]
- Xu J, Wu KJ, Jia QJ, et al. Roles of miRNA and lncRNA in triple-negative breast cancer. J Zhejiang Univ Sci B 2020;21:673-89. [Crossref] [PubMed]
- Li X, Li Y, Yu X, et al. Identification and validation of stemness-related lncRNA prognostic signature for breast cancer. J Transl Med 2020;18:331. [Crossref] [PubMed]
- Shi Q, Li Y, Li S, et al. LncRNA DILA1 inhibits Cyclin D1 degradation and contributes to tamoxifen resistance in breast cancer. Nat Commun 2020;11:5513. [Crossref] [PubMed]
- Kim CY, Oh JH, Lee JY, et al. The LncRNA HOTAIRM1 Promotes Tamoxifen Resistance by Mediating HOXA1 Expression in ER+ Breast Cancer Cells. J Cancer 2020;11:3416-23. [Crossref] [PubMed]
- Zhai J, Jiang JF, Shi L. Lycorine weakens tamoxifen resistance of breast cancer via abrogating HAGLR-mediated epigenetic suppression on VGLL4 by DNMT1. Kaohsiung J Med Sci 2023;39:278-89. [Crossref] [PubMed]
- Niu Y, Zhao X, Wu YS, et al. N6-methyl-adenosine (m6A) in RNA: an old modification with a novel epigenetic function. Genomics Proteomics Bioinformatics 2013;11:8-17. [Crossref] [PubMed]
- Liu Y, Shi M, He X, et al. LncRNA-PACERR induces pro-tumour macrophages via interacting with miR-671-3p and m6A-reader IGF2BP2 in pancreatic ductal adenocarcinoma. J Hematol Oncol 2022;15:52. [Crossref] [PubMed]
- Wang J, Chen L, Qiang P. The role of IGF2BP2, an m6A reader gene, in human metabolic diseases and cancers. Cancer Cell Int 2021;21:99. [Crossref] [PubMed]
- Hu X, Peng WX, Zhou H, et al. IGF2BP2 regulates DANCR by serving as an N6-methyladenosine reader. Cell Death Differ 2020;27:1782-94. [Crossref] [PubMed]
- Dong L, Geng Z, Liu Z, et al. IGF2BP2 knockdown suppresses thyroid cancer progression by reducing the expression of long non-coding RNA HAGLR. Pathol Res Pract 2021;225:153550. [Crossref] [PubMed]
- Zhou Y, Zeng P, Li YH, et al. SRAMP: prediction of mammalian N6-methyladenosine (m6A) sites based on sequence-derived features. Nucleic Acids Res 2016;44:e91. [Crossref] [PubMed]
- Zhou M, Dong M, Yang X, et al. The emerging roles and mechanism of m6a in breast cancer progression. Front Genet 2022;13:983564. [Crossref] [PubMed]
- Wan W, Ao X, Chen Q, et al. METTL3/IGF2BP3 axis inhibits tumor immune surveillance by upregulating N(6)-methyladenosine modification of PD-L1 mRNA in breast cancer. Mol Cancer 2022;21:60. [Crossref] [PubMed]
- McMullen ER, Gonzalez ME, Skala SL, et al. CCN6 regulates IGF2BP2 and HMGA2 signaling in metaplastic carcinomas of the breast. Breast Cancer Res Treat 2018;172:577-86. [Crossref] [PubMed]
- Liu W, Li Y, Wang B, et al. Autoimmune Response to IGF2 mRNA-Binding Protein 2 (IMP2/p62) in Breast Cancer. Scand J Immunol 2015;81:502-7. [Crossref] [PubMed]
- Zheng F, Du F, Zhao J, et al. The emerging role of RNA N6-methyladenosine methylation in breast cancer. Biomark Res 2021;9:39. [Crossref] [PubMed]
- Li Y, Francia G, Zhang JY. p62/IMP2 stimulates cell migration and reduces cell adhesion in breast cancer. Oncotarget 2015;6:32656-68. [Crossref] [PubMed]
- Chen DH, Zhang JG, Wu CX, et al. Non-Coding RNA m6A Modification in Cancer: Mechanisms and Therapeutic Targets. Front Cell Dev Biol 2021;9:778582. [Crossref] [PubMed]
- Chen C, Guo Y, Guo Y, et al. m6A Modification in Non-Coding RNA: The Role in Cancer Drug Resistance. Front Oncol 2021;11:746789. [Crossref] [PubMed]
- Hou P, Meng S, Li M, et al. LINC00460/DHX9/IGF2BP2 complex promotes colorectal cancer proliferation and metastasis by mediating HMGA1 mRNA stability depending on m6A modification. J Exp Clin Cancer Res 2021;40:52. [Crossref] [PubMed]