PGC1α curtails prostate cancer metastasis via metabolic rewiring
Prostate cancer is the second most commonly diagnosed malignancy in men; and in spite of recent therapeutic advances, it remains the fifth leading cause of male cancer deaths worldwide (1,2). Aside from prostatectomy and radiation, used with curative intent for the treatment of localized prostate cancer, approved life-prolonging treatment options for metastasized prostate cancer are limited to androgen receptor signaling inhibitors, microtubule targeting taxane chemotherapeutics, the bone targeted radioisotope Ra223, and active immunotherapy with sipuleucel-T (2). Hence, to improve prostate cancer care it is essential to identify novel treatment targets (3). Comprehensive genetic analyses have revealed a number of actionable molecular aberrations, including alterations of the PI3K-AKT and WNT signaling pathways, and DNA repair defects (4-6).
Although alterations of metabolic pathways have been recognized as essential aspects of cancer progression and metastasis, there is a need to better understand the molecular mechanisms behind how the metabolic landscape of cancer cells changes in coordinated manner to support tumor growth (7). In a detailed and elegant study Torrano et al. examined the peroxisome proliferator-activated receptor gamma co-activator 1 alpha (PGC1α, also PPARGC1A) transcriptional network and its role in suppressing prostate cancer metastasis (8). Initially, a bioinformatics analysis was completed to identify regulators of prostate cancer metabolism responsible for disease progression. Amongst three metabolic co-regulators identified in most or all data sets studied, covering different stages of prostate cancer (i.e., PGC1α, PGC1β, HDAC1), the expression of PGC1α was the only one found to be associated with the Gleason scoring system and disease-free survival. The expression pattern of PGC1α was characteristic of a tumor suppressor.
Next, PGC1α was chosen for further analyses. While PGC1α deletion did not promote prostate cancer initiation, it was shown to be responsible for impairing prostate cancer growth and metastasis. PGC1α has been identified as a promoter of inflammation, angiogenesis and the production of reactive oxygen species; neither of these processes, however, contributed to the PGC1α-mediated anti-prostate cancer effects described by Torrano et al. In gene expression profiling and metabolomics analyses, PGC1α was found to significantly alter gene expression and metabolite levels with respect to mitochondrial catabolic programs and oxidative processes such as fatty-acid β-oxidation. Altogether, the findings suggest that in prostate cancer PGC1α may serve as a metabolic regulator balancing a catabolic, tumor suppressive state (high PGC1α expression) versus an anabolic, tumor promoting state (low PGC1α expression) (Figure 1).
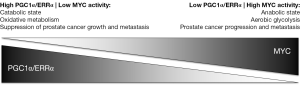
Finally, Torrano et al. used promoter and gene set enrichment analyses to identify estrogen-related receptor alpha (ERRα or ESRRA) as a major transcription factor mediating the tumor suppressive activities of PGC1α. Furthermore, using two independent patient gene expression data sets they demonstrated that the PGC1α/ERRα transcriptional program is positively associated with time to prostate cancer recurrence.
ERRα and two additional ERR isoforms (i.e., ERRβ and ERRγ) belong to a subfamily of constitutively active, orphan nuclear receptors that share high homology with estrogen receptors, have been implicated in metabolic regulation, are involved in prostate and breast cancer biology, and often exert opposing biological effects (9). A number of natural phytoestrogens have ERR-activating properties and could serve as lead compounds for the development of potent and specific ERRα agonists with potential anti-prostate cancer properties (9). Yet, are the findings by Torrano et al. compelling enough to consider PGC1α/ERRα activation as a novel treatment strategy for prostate cancer? Fittingly, the authors are cautious with the interpretation of their results.
The importance of metabolic rewiring for malignant growth in general, and the role of the PGC1 co-activator and ERR transcription factor families for oncogenic metabolic reprogramming in particular, are widely recognized (7,10). However, the consequences of PGC1/ERR signaling are highly context-dependent. Torrano et al. describe prostate cancer suppressive PGC1α/ERRα activities. Conversely, the expression of PGC1α/ERRα has been associated with tumor promoting properties in prostate and other cancers by others. As an example, Fradet et al. showed that ERRα promotes prostate cancer progression in bone (11). Although the opposing conclusions of the Torrano and Fradet studies are not easily reconciled, one notes that the anti-metastatic consequences of inducing PGC1α expression in PC-3 prostate cancer cells in the study by Torrano et al. are more pronounced regarding visceral (i.e., lung) than bone metastases (8). Could it be that the role of PGC1α/ERRα signaling is not only tumor type dependent, but also dependent on the organ site of metastasis?
Although a PGC1α/ERRα activation strategy may be particularly promising in tumors with low PGC1α and/or ERRα activity, it is currently not known how frequently such a constellation would exist in prostate cancer. Copy number alterations or mutations of PGC1α and ERRα are rarely found in prostate adenocarcinomas, and therefore are unlikely genetic driver aberrations (Table 1) (4,5). Indeed, PGC1α deletion alone was not found to initiate prostate carcinogenesis in the study by Torrano et al. (8). On the other hand, the PGC1α transcriptional network is under the control of MYC, a master regulator of cancer cell metabolism (12). MYC and PGC1α expression are inversely related to each other (Figure 1) (13,14). Intriguingly, MYC is amplified in more than 50% of neuroendocrine prostate cancers (Table 1), which are characterized by aggressive clinical behavior, are rarely diagnosed de novo, but are increasingly recognized as a prostate cancer phenotype in patients with inherent or acquired resistance to androgen receptor signaling inhibitors (6). Furthermore, the PC-3 prostate cancer cell line, prominently used in the study by Torrano et al., harbors neuroendocrine features. Thus, MYC amplification may contribute to a PGC1α low state, potentially amenable to a PGC1α/ERRα activation strategy, and may serve as a predictive marker for such a treatment approach.
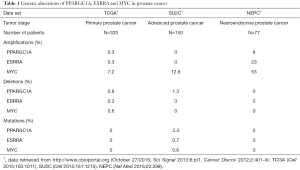
Full table
In summary, the findings by Torrano et al. identify the PGC1α/ERRα transcriptional network as one of only few well-defined molecular mechanisms of prostate cancer metastasis. Accounting for the opposing biological functions attributed to PGC1α and ERRα in different tumor models, the study by Torrano et al. serves as an invaluable starting point to obtain a more detailed picture of the complex interplay between tumor cell metabolism and prostate cancer metastasis. Only time will tell if PGC1α/ERRα modulation will become a strategy to treat prostate cancer. The latter may apply especially to neuroendocrine prostate cancers, an area of increasing therapeutic need, due to the widespread use of second-generation androgen receptor signaling inhibitors.
Acknowledgments
Funding: This work was supported by funds of the Joseph and Silvana Cancer Research Fund to U Emmenegger.
Footnote
Provenance and Peer Review: This article was commissioned and reviewed by the Section Editor Peng Zhang (Department of Urology, Union Hospital, Tongji Medical College, Huazhong University of Science and Technology, Wuhan, China).
Conflicts of Interest: All authors have completed the ICMJE uniform disclosure form (available at http://dx.doi.org/10.21037/tcr.2016.12.23). The authors have no conflicts of interest to declare.
Ethical Statement: The authors are accountable for all aspects of the work in ensuring that questions related to the accuracy or integrity of any part of the work are appropriately investigated and resolved.
Open Access Statement: This is an Open Access article distributed in accordance with the Creative Commons Attribution-NonCommercial-NoDerivs 4.0 International License (CC BY-NC-ND 4.0), which permits the non-commercial replication and distribution of the article with the strict proviso that no changes or edits are made and the original work is properly cited (including links to both the formal publication through the relevant DOI and the license). See: https://creativecommons.org/licenses/by-nc-nd/4.0/.
References
- Torre LA, Bray F, Siegel RL, et al. Global cancer statistics, 2012. CA Cancer J Clin 2015;65:87-108. [Crossref] [PubMed]
- Gillessen S, Omlin A, Attard G, et al. Management of patients with advanced prostate cancer: recommendations of the St Gallen Advanced Prostate Cancer Consensus Conference (APCCC) 2015. Ann Oncol 2015;26:1589-604. [Crossref] [PubMed]
- Yap TA, Smith AD, Ferraldeschi R, et al. Drug discovery in advanced prostate cancer: translating biology into therapy. Nat Rev Drug Discov 2016;15:699-718. [Crossref] [PubMed]
- Robinson D, Van Allen EM, Wu YM, et al. Integrative clinical genomics of advanced prostate cancer. Cell 2015;161:1215-28. [Crossref] [PubMed]
- Cancer Genome Atlas Research Network. The Molecular Taxonomy of Primary Prostate Cancer. Cell 2015;163:1011-25. [Crossref] [PubMed]
- Beltran H, Prandi D, Mosquera JM, et al. Divergent clonal evolution of castration-resistant neuroendocrine prostate cancer. Nat Med 2016;22:298-305. [Crossref] [PubMed]
- Sullivan LB, Gui DY, Vander Heiden MG. Altered metabolite levels in cancer: implications for tumour biology and cancer therapy. Nat Rev Cancer 2016;16:680-93. [Crossref] [PubMed]
- Torrano V, Valcarcel-Jimenez L, Cortazar AR, et al. The metabolic co-regulator PGC1α suppresses prostate cancer metastasis. Nat Cell Biol 2016;18:645-56. [Crossref] [PubMed]
- Misawa A, Inoue S. Estrogen-Related Receptors in Breast Cancer and Prostate Cancer. Front Endocrinol (Lausanne) 2015;6:83. [Crossref] [PubMed]
- Deblois G, St-Pierre J, Giguère V. The PGC-1/ERR signaling axis in cancer. Oncogene 2013;32:3483-90. [Crossref] [PubMed]
- Fradet A, Bouchet M, Delliaux C, et al. Estrogen related receptor alpha in castration-resistant prostate cancer cells promotes tumor progression in bone. Oncotarget 2016; [Epub ahead of print]. [Crossref] [PubMed]
- Hsieh AL, Walton ZE, Altman BJ, et al. MYC and metabolism on the path to cancer. Semin Cell Dev Biol 2015;43:11-21. [Crossref] [PubMed]
- Ahuja P, Zhao P, Angelis E, et al. Myc controls transcriptional regulation of cardiac metabolism and mitochondrial biogenesis in response to pathological stress in mice. J Clin Invest 2010;120:1494-505. [Crossref] [PubMed]
- Sancho P, Burgos-Ramos E, Tavera A, et al. MYC/PGC-1α Balance Determines the Metabolic Phenotype and Plasticity of Pancreatic Cancer Stem Cells. Cell Metab 2015;22:590-605. [Crossref] [PubMed]