PD-L1 combined with HDAC9 is a useful prognostic predictor in hepatocellular carcinoma
Introduction
Hepatocellular carcinoma (HCC) is the sixth most common malignancy and the third leading cause of cancer-related death worldwide (1,2). Currently, surgical resection and liver transplantation are considered as the only available approaches to treat HCC. However, large percentage of patients are diagnosed with HCC at an advanced stage, thereby missing the local treatment opportunity (3,4). Several multi-targeted tyrosine kinase inhibitors (TKIs), including sorafenib, lenvatinib, regorafenib and cabozantinib, are considered to be the most efficient targeted drugs for the systemic treatment of patients with advanced HCC (5-7). However, a study demonstrated that sorafenib could extend the median overall survival (OS) by only 3 months (8). Therefore, novel therapeutic modalities are urgently needed to treat patients with advanced HCC.
Programmed cell death protein 1 (PD-1), an inhibitory receptor expressed on activated T cells, negatively regulates T cell responses. When PD-1 binds to the co-inhibitory receptors on tumor cells, such as programmed death ligand 1 or 2 (PD-L1 or PD-L2), s T cell the proliferation and cytokine secretion of T cells were attenuated, ultimately leading to T cell dysfunction or apoptosis (9-11). Immunotherapy, especially the immune checkpoint blockade with anti-cytotoxic T lymphocyte associated antigen 4 (CTLA-4) and anti-PD-1/PD-L1 antibodies, has been successfully utilized in treating several types of advanced tumors, including non-small cell lung cancer (NSCLC) (12), melanoma, bladder carcinoma (13,14), Hodgkin’s lymphoma (15,16), and Merkel cell carcinoma (17). Clinical studies on antibodies against PD-1 in advanced HCC, showed safety and durable antitumor activity, suggesting that immunotherapy with immune checkpoint inhibitors could bring new hope for advanced HCC treatment. Nivolumab, a PD-1 monoclonal antibody, has been approved by the Food and Drug Administration (FDA) for the systemic treatment of HCC, in patients who have previously received sorafenib treatment (18). However, the response rate of patients underwent anti-PD-1 monotherapy was <20% (19). Importantly, reliable predictive biomarkers to assess the response of patients with HCC to immunotherapy are still lacking. Accumulating evidence has suggested that the expression profile of PD-L1 on tumor cells is significantly associated with the therapeutic effect of PD-1 blocking antibodies (20,21). In addition, a study revealed that anti-PD-1 therapy exerted an increased objective response rate in patients with PD-L1 positive disease (22). Since the expression of PD-L1 is very dynamic, revealing the regulatory mechanism underlying PD-L1 expression remains a main challenge for tumor immunotherapy.
It has been shown that histone deacetylases (HDACs) are overexpressed and associated with tumor progression in several cancer types such as lung, gastric, colorectal, breast, prostate and pancreatic carcinomas (23-28). Clinical trials are currently exploring the potential of HDAC inhibitors as anticancer agents. Several studies have shown that treatment with epigenetic modulators, including HDAC inhibitors, upregulates the expression of checkpoint inhibitors in tumor cells and enhances the responses to blockade therapy (29,30). These findings prompted any investigation into whether there was any association between HDACs and PD-Ls. Therefore, the present study evaluated the protein expression levels and clinical significance of PD-L1 in HCC and evaluated the correlation between HDACs and PD-L1.
We present the following article in accordance with the ARRIVE reporting checklist (available at http://dx.doi.org/10.21037/tcr-20-3415).
Methods
Patients and sample collection
One hundred nine patients were randomly collected from HCC patients who underwent liver resection in Eastern Hepatobiliary Surgery Hospital (Shanghai, China) from September 2012 to October 2014. The patients did not receive any preoperative anticancer treatment. All patients were observed until November 2019. OS referred to the interval between the dates of surgery and death. Disease-free survival (DFS) referred to the interval between the surgery and recurrence. If recurrence was not diagnosed, patients were censored on the date of death or the last follow-up. The study was conducted in accordance with the Declaration of Helsinki (as revised in 2013). The study was approved by the Ethic Committee of Eastern Hepatobiliary Surgery Hospital (No.: SHDC12017122) and informed consent was taken from all the patients.
Real time RT-PCR
Total RNA of HCC tumor tissues was extracted using Trizol reagent (Gibco, Carlsbad, CA, USA). Real-time PCR was performed using an ABI 7300 Fast Real-time PCR System (Applied Biosystems) and SYBR Green PCR kit (Applied TaKaRa, Japan). The primer sequences are shown as following (to detect all isoforms of HDAC9, the primers used for HDAC9 qPCR were designed to amplify a fragment from exon 21 to exon 23 of the HDAC9 mRNA):
Gene | Forward primer | Reverse primer |
---|---|---|
PD-L1 | 5’-GTTGAAGGACCAGCTCTCCC-3’ | 5’-CTTGTAGTCGGCACCACCAT-3’ |
HDAC2 | 5’-TGAAGTCTTGTTTCAGTGGCT-3’ | 5’-AAGCTATAGAGGGCAAGGT-3’ |
HDAC9 | 5’-TTCAGGACCATCGTGAAGCC-3’ | 5’-TAGAGCCAACACCACACGTC-3’ |
β-actin | 5’-CGCGAGAAGATGCCCAGATC-3’ | 5’-TCACCGGAGTCCATCACGA-3’ |
Immunohistochemical staining and quantification
Paraffin embedded tissue samples were cut into 5 µm sections. Immunohistochemical staining were performed following the routine protocol. The primary antibodies were the following: anti-PD-L1 (ab205921, Abcam, Cambridge, UK), anti-HDAC9 (ab109446, Abcam, Cambridge, UK) and anti-HDAC2 (ab32117, Abcam, Cambridge, UK). The density of IHC staining was quantified by Image-Pro Plus v6.2 software (Media Cybernetics Inc., Bethesda, MD).
Cell line and lentivirus
HCC cell line MHCC-97L were purchased from Cell Bank of Type Culture Collection of Chinese Academy of Sciences, Shanghai Institute of Cell Biology, Chinese Academy of Sciences. Full length human HDAC9 or green fluorescent protein (GFP) CDS sequence was inserted into pLenti-CMV-3FLAG lentiviral vector (OBiO Technology, Shanghai). The lentivirus, LV-HDAC9 or LV-GFP were infected into MHCC-97L cells with a multiplicity of infection (MOI) 20 for 4 hours. After 12 hours, the original medium was replaced with fresh medium.
Western blot analysis
Whole cell extracts were prepared in lysis buffer (20 mM Tris, pH 7.4, 137 mM NaCl, 10% glycerol, 1% Triton X-100, 2 mM EDTA, 1 mM PMSF, 10 mM NaF, 5 mg/mL aprotinin, 20 mM leupeptin, and 1 mM sodium orthovanadate) and centrifuged at 12,000 rpm for 15 min. Protein extracts were subjected to SDS-PAGE. The primary antibodies used were anti-HDAC9 (ab109446, Abcam, Cambridge, UK) and anti-GAPDH (60004, Proteintech, Wuhan, China).
Nude mice xenograft model
Experiments were performed under a project license (No. 2018ZX10723204) granted by institutional ethics committee of Second Military Medical University (Shanghai, China), in compliance with the institutional guidelines for the care and use of animals. Five-week-old male athymic BALB/c nude mice (weight ~19–22 g) were obtained from Shanghai Model Organisms Center (Shanghai, China) and allowed to acclimate to their new surroundings for one week. All mice were kept in home cages in a specific pathogen-free (SPF) environment with free access to sterilized food and water, and animal experiments were conducted at the Second Military Medical University Animal Experiment Center (Shanghai, China). The home cages were cleaned, and sterilized food and water were exchanged weekly during the experiments. In the animal xenograft assays, 5×106 HDAC9-transfected MHCC-97L cells or control cells were injected subcutaneously in the right flank of mice (n=6). Tumor size was measured every three days, and tumor volume was calculated by the formula: (width)2 × length/2. Mice were sacrificed 3 weeks after injection and tumors were excised, fixed in 10% buffered formalin solution and used for further experiments.
Statistical analysis
The SPSS version 21 and R version 3.2.3 softwares were used in data analysis. The experiment results were shown as mean ± SEM. Data analysis was carried out by Student’s t test, χ2 test, Fisher’s exact test. In survival analysis, the median value for PD-L1 expression was used to divide the patients into high-PD-L1 (scored as 1) and low-PD-L1 (scored as 0) groups. In the same way, the patients were divided into high-HDAC9 (scored as 1) and low-HDAC9 (scored as 0) groups. We define the patient cases that have two “0” scores as “combined low PD-L1 and HDAC9”, and those have two “1” scores as “combined high PD-L1 and HDAC9”. Kaplan-Meier was used to calculate survival curves. Multivariate Cox proportional hazards regression model was used to determine prognostic factors. The significant difference was determined by P<0.05.
Results
PD-L1 is upregulated in HCC samples
To determine the expression status of PD-L1 in HCC, 109 patients with HCC, who underwent liver resection (Table S1), from the tissue microarrays (TMA) were used to detect the protein levels of PD-L1 by immunohistochemical (IHC) staining. The results showed that PD-L1 was increased in 83/109 (76.1%) HCC tumor samples compared with the peritumoral normal tissues (Figure 1A). Representative PD-L1 immunostaining results showing the different levels of PD-L1 in HCC and peritumoral tissues are illustrated in Figure 1B. In addition, the average PD-L1 protein exhibited remarkably higher levels in HCC tissues in comparison with normal peritumoral tissues (Figure 1C). Based on the IHC results of PD-L1 expression in tumor tissues, we divided 109 HCC patients into two groups, namely the high-expression (n=55) and low-expression (n=54) groups. Although PD-L1 expression was positively associated with recurrence (Table S2), no obvious difference in DFS or OS was observed between the high- and low-expression groups (Figure 1D). These findings suggested that the upregulated PD-L1 expression could not serve as a prognostic predictor for HCC.
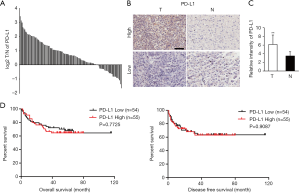
mRNA expression of PD-L1 in HCC is associated with HDACs
To investigate whether PD-L1 expression was associated with that of HDACs in HCC, a gene correlation analysis was carried out using Gene Expression Profiling Interactive Analysis (GEPIA) using RNA expression data from The Cancer Genome Atlas (TCGA) database. The results indicated that the mRNA expression levels of seven HDACs in 369 HCC tissue samples, including HDAC1, HDAC2, HDAC3, HDAC4, HDAC7, HDAC8 and HDAC9, showed a positive correlation with the mRNA levels of PD-L1 (CD274; P<0.01, Figure 2A). Notably, among the seven HDACs tested, HDAC9 (R=0.42) and HDAC2 (R=0.37) were the most significantly correlated with PD-L1 expression. The mRNA expression levels of PD-1, HDAC2 and HDAC9 were also evaluated in 84 clinically and pathologically characterized HCC tissues from our hospital. RT-qPCR analysis showed that the expression of HDAC2 (R2=0.0766) and HDAC9 (R2=0.442) was positively correlated with PD-L1 expression in HCC samples (Figure 2B).
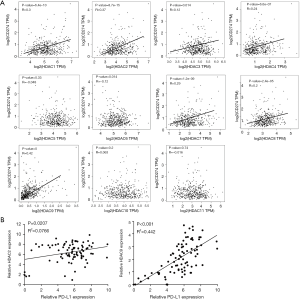
Protein expression levels of HDAC9 and HDAC2 are associated with PD-L1 in HCC TMA
To further verify the correlation between PD-1 and HDAC expression, the protein expression levels of HDAC9 and HDAC2 in HCC TMAs were determined by IHC staining. As illustrated in Figure 3A, the HDAC9 and HDAC2 protein levels were elevated in 67 (67/109; 61.5%) and 69 (69/109; 63.3%) HCC samples, respectively, compared with those in the matched peritumoral tissues (Figure 3B). Representative IHC results in HCC tissues with high or low HDAC9 and HDAC2 protein levels are shown in Figure 3C and D. Furthermore, the average protein expression levels of HDAC9 and HDAC2 were dramatically higher in HCC tissues in comparison with normal peritumoral tissues (Figure 3E,F).
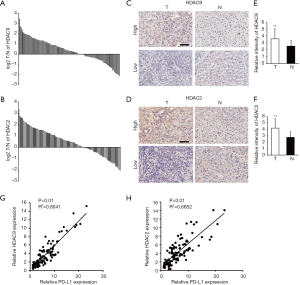
Subsequently, the association of HDAC9 and HDAC2 with PD-L1 protein expression was analyzed. The expression of both HDAC9 and HDAC2 was positively correlated with PD-L1 protein expression levels, while the association was stronger for HDAC9 than HDAC2. This finding was consistent with the data obtained on the mRNA level from HCC TCGA (Figure 3G,H).
Combined high expression of HDAC9 and PD-L1 in HCC tissues predicts poor prognosis
To determine the clinical significance of HDAC9 expression in human HCC, HCC patients were divided into high- (> median) and low-expression (< median) groups according to the median values for HDAC9 and PD-L1 expression. The survival rates were compared between the low (n=54) and high (n=55) HDAC9 expression groups. No significant differences were observed in OS and DFS between the two groups (Figure 4A,B). However, patients with high levels of both HDAC9 and PD-L1 (n=44) exhibited increased distant metastasis (P=0.036) and recurrence (P=0.006) in comparison with those with low levels of HDAC9 and PD-L1 (n=46; Table 1). Kaplan-Meier survival analyses showed that patients with high HDAC9 and PD-L1 expression had markedly reduced DFS and OS (Figure 4C,D). In addition, the clinical significance of HDAC2 expression in human HCC samples was also investigated; however, no differences were observed in then survival rate when the HDAC2 and PD-L1 expression levels were compared (Table S3; Figure S1). Moreover, univariate and multivariate analyses also suggested that the combined high HDAC9 and PD-L1 expression was an independent factor for worse OS (HR =2.164; 95% CI =1.1–4.257; P=0.025; Table 2). These results indicated that the combination of PD-L1 and HDAC9 expression could be a powerful predictor for poor prognosis in patients with HCC.
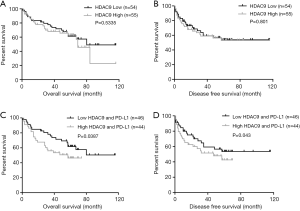
Table 1
Variable | All cases | HDAC9 + PD-L1 density | P value | |
---|---|---|---|---|
Low (n=46) | High (n=44) | |||
Age (years) | 0.506 | |||
<50 | 42 | 21 | 21 | |
>50 | 48 | 25 | 23 | |
Gender | 0.618 | |||
Female | 8 | 4 | 4 | |
Male | 82 | 42 | 40 | |
HBsAg | 0.459 | |||
Positive | 73 | 38 | 35 | |
Negative | 17 | 8 | 9 | |
AFP (ng⁄ mL) | 0.143 | |||
<20 | 24 | 15 | 9 | |
>20 | 66 | 31 | 35 | |
Cirrhosis | 0.148 | |||
Yes | 47 | 27 | 20 | |
No | 43 | 19 | 24 | |
Tumor size (cm) | ||||
<5 | 34 | 18 | 16 | 0.479 |
>5 | 56 | 28 | 28 | |
TNM stage | 0.165 | |||
I–II | 73 | 35 | 38 | |
III–IV | 17 | 11 | 6 | |
Distant metastasis | 0.036 | |||
Yes | 11 | 2 | 9 | |
No | 79 | 44 | 35 | |
Microvascular invasion | 0.498 | |||
Yes | 46 | 23 | 23 | |
No | 44 | 23 | 21 | |
Involucrum | 0.275 | |||
Complete | 31 | 14 | 17 | |
Incomplete or absent | 59 | 32 | 27 | |
Recurrence | 0.006 | |||
Yes | 38 | 12 | 26 | |
No | 52 | 34 | 18 |
Patients with HCC were divided into Low HDAC9 + PD-L1 expression group (whose final density was lower than the median) and High expression group (whose final density was higher than the median). The patient and disease profiles in each group were compared. Italic P values indicate statistical difference.
Table 2
Variable | Univariable analysis | Multivariable analysis | |||||
---|---|---|---|---|---|---|---|
HR | 95% CI | P | HR | 95% CI | P | ||
PDL1 expression | 1.1 | 0.576–2.101 | 0.774 | – | – | – | |
HDAC9 expression | 1.389 | 0.724–2.664 | 0.322 | – | – | – | |
HDAC9 + PDL1 expression | 2.115 | 1.101–4.062 | 0.024 | 2.164 | 1.1–4.257 | 0.025 | |
HDAC2 expression | 1.011 | 0.53–1.927 | 0.973 | – | – | – | |
HDAC2 + PDL1 expression | 0.993 | 0.498–1.977 | 0.983 | – | – | – | |
Age, year | 0.814 | 0.427–1.552 | 0.532 | – | – | – | |
Gender, male vs. female | 2.112 | 0.508–8.785 | 0.304 | – | – | – | |
Tumor size, cm | 5.784 | 2.247–14.892 | 0 | 4.108 | 1.536–10.984 | 0.005 | |
AFP, μg/L | 1.569 | 0.689–3.573 | 0.284 | – | – | – | |
Distant metastasis, yes vs. no | 5.912 | 2.836–12.323 | 0 | 2.409 | 1.101–5.273 | 0.028 | |
Microvascular invasion, yes vs. no | 3.648 | 1.764–7.547 | 0 | 2.701 | 1.265–5.769 | 0.01 | |
Involucrum, complete vs. incomplete | 0.495 | 0.239–1.023 | 0.058 | – | – | – | |
HBV infection, yes vs. no | 0.621 | 0.3–1.283 | 0.198 | – | – | – | |
Cirrhosis, yes vs. no | 0.857 | 0.449–1.634 | 0.639 | – | – | – | |
Differentiation, III/IV vs. I/II | 0.427 | 0.151–1.205 | 0.108 | – | – | – |
HR, hazard ratio; CI, confidence interval; OS, overall survival.
HDAC9 overexpression promotes HCC cell growth in vivo
Whether HDAC9 had any direct effect on HCC cells? Next, lentiviral vectors encoding HDAC9 and GFP (control) were constructed and infected into MHCC-97L cells. The expression levels of HDAC9 in the infected cells were detected by western blot analysis (Figure 5A). Subsequently, the HDAC9-overexpressed and control cells were used to establish xenografts model on nude mice to assess tumor growth in vivo. As shown in Figure 5B and C, mice in the HDAC9-overexpressed group exhibited accelerated tumor growth and increased tumor volume compared with the control group. Furthermore, IHC staining revealed upregulated PD-L1 expression in the HDAC9-overexpressed tumors (Figure 5D). Taken together, the aforementioned findings suggested that HDAC9 overexpression in HCC cells could promote tumor growth and PD-L1 expression in vivo.
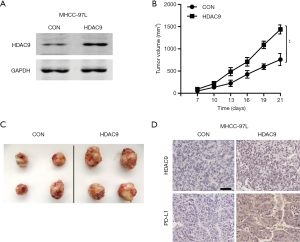
Discussion
The expression of PD-L1 varies among different cancer types. It has been demonstrated that PD-L1 is overexpressed in several types of tumors, including melanoma, ovarian cancer, breast cancer, lung cancer and hematological malignancies (30). Currently, the expression profile of PD-L1 in patients with HCC has been poorly investigated. Herein, a cohort of 109 HCC specimens were analyzed. The results showed that PD-L1 was overexpressed in the majority of HCC (76.1%) tumor tissues compared with matched normal peritumoral tissues. However, the survival analysis failed to reveal any significant prognostic association between PD-L1 expression with OS, which was consistent with a previous analysis by Xu et al. (31). These findings indicated that PD-L1 expression alone could not serve as a prognostic marker for HCC.
Emerging evidence has demonstrated that the expression levels of PD-L1 related to the response of PD-1/PD-L1 blockade immunotherapy in a variety types of cancer (32). The FDA has approved PD-L1 expression as a companion diagnostic indicator for anti-PD-1 therapy in NSCLC (33,34). By contrast, several studies revealed no association between PD-L1 expression and response to anti-PD-1 therapy (35,36). Therefore, PD-L1 is not considered as an appropriate predictive biomarker for selecting patients predisposed to respond to immunotherapy. Additional predictive biomarkers or combined indicators are urgently needed for selecting patients most likely to benefit from PD-1-based therapy.
Recently, several studies have shown that treatment with HDAC inhibitors induce the expression of checkpoint inhibitors in tumor cells. For example, Woods et al. (29) reported that HDAC inhibitors administration in melanoma-bearing mice lead to enhanced expression of PD-L1 and PD-L2mediated by increased histone acetylation. In the present study, RNA expression data from TCGA database revealed positive correlation between PD-L1 and HDAC expression. More specifically, the HDAC9 and HDAC2 expressions were most significantly correlated with PD-L1 expression. Additionally, the protein expression levels of HDAC9 and HDAC2 were notably upregulated in HCC samples, which was consistent with the PD-L1 mRNA expression profile. Correlation analysis revealed positive correlation between PD-L1 expression and both HDAC9 and HDAC2. This correlation was stronger with HDAC9 expression. Furthermore, survival analysis showed that the combined high PD-L1/HDAC9 levels, but not those of HDAC2, were significantly correlated with worse prognosis. These data strongly indicated that PD-L1/HDAC9 expression could serve as a novel prognostic marker for HCC. The current study suggested a potential role of HDAC9 in modulating PD-L1 expression. However, this mechanism needs to be further investigated.
To detect whether HDAC9 had any effect on PD-L1 expression in human HCC cells, we injected HDAC9-transfected MHCC-97L cells and control cells into immunodeficient nude mice. The results further confirmed that HDAC9 in human HCC cells promoted PD-L1 expression in vivo. As a crucial immune-checkpoint receptor, PD-1 could be expressed by several immune cells, for example CD8+ T cells, NK cells and macrophages. By upregulating PD-L1 expression, HDAC9-transfected HCC cells possibly block antitumor immune induced by multiple immunocytes, and thus yield a bigger tumor size, even in T-cell-deficient nude mice. As a member of the class IIa histone deacetylases, HDAC9 catalyzes the deacetylation of histones and transcription factors to suppress or activate gene transcription. Many studies show that HDAC9 regulates the expression of cancer related genes by altering chromatin structures of promotor region or the transcriptional activities, such as STAT5, Myc and β-catenin, some of which control PD-L1 gene transcription. Therefore, HDAC9 may promote PD-L1 expression through activating its transcription factors, and more mechanistic experiments are needed to validate the hypothesis in future.
Despite PD-1/PD-L1 blockade is emerging as a promising approach in advanced cancer therapy, the response rate of patients to PD-1/PD-L1 targeted monotherapy remains still unsatisfactory in HCC. Therefore, the development of combination strategies to improve the effectiveness of HCC treatment is urgently needed. Previous findings have shown that HDAC inhibitors can enhance the response to anti-PD-1 therapy in mouse models. Herein, the results in human HCC specimens supported that the treatment with combined inhibition of HDAC9 and PD-1/PD-L1 could be considered as a potential approach to benefit patients with HCC.
Conclusions
The present study demonstrated that PD-L1 was upregulated and highly associated with HDAC9 and HDAC2 expression in HCC. Survival analysis showed that PD-L1/HDAC9 expression levels could be used as a powerful prognostic marker for patients with HCC. These results highlighted the strong association between HDACs and immunotherapy. However, further studies are necessary to investigate the potential clinical application of the combined therapy with specific HDAC9 inhibitors and PD-1 blockade in treating HCC.
Acknowledgments
Funding: This study was supported by grants from
Footnote
Reporting Checklist: The authors have completed the ARRIVE reporting checklist. Available at http://dx.doi.org/10.21037/tcr-20-3415
Data Sharing Statement: Available at http://dx.doi.org/10.21037/tcr-20-3415
Conflicts of Interest: All authors have completed the ICMJE uniform disclosure form (available at http://dx.doi.org/10.21037/tcr-20-3415). The authors have no conflicts of interest to declare.
Ethical Statement: The authors are accountable for all aspects of the work in ensuring that questions related to the accuracy or integrity of any part of the work are appropriately investigated and resolved. The study was conducted in accordance with the Declaration of Helsinki (as revised in 2013). The study was approved by the Ethic Committee of Eastern Hepatobiliary Surgery Hospital (No.: SHDC12017122) and informed consent was taken from all the patients. Experiments were performed under a project license (No.: 2018ZX10723204) granted by institutional ethics committee of Second Military Medical University (Shanghai, China), in compliance with the institutional guidelines for the care and use of animals.
Open Access Statement: This is an Open Access article distributed in accordance with the Creative Commons Attribution-NonCommercial-NoDerivs 4.0 International License (CC BY-NC-ND 4.0), which permits the non-commercial replication and distribution of the article with the strict proviso that no changes or edits are made and the original work is properly cited (including links to both the formal publication through the relevant DOI and the license). See: https://creativecommons.org/licenses/by-nc-nd/4.0/.
References
- Torre LA, Bray F, Siegel RL, et al. Global cancer statistics, 2012. CA Cancer J Clin 2015;65:87-108. [Crossref] [PubMed]
- Llovet JM, Zucman-Rossi J, Pikarsky E, et al. Hepatocellular carcinoma. Nat Rev Dis Primers 2016;2:16018. [Crossref] [PubMed]
- Abou-Alfa GK, Huitzil-Melendez FD, O’Reilly EM, et al. Current management of advanced hepatocellular carcinoma. Gastrointest Cancer Res 2008;2:64-70. [PubMed]
- Ikeda M, Morizane C, Ueno M, et al. Chemotherapy for hepatocellular carcinoma: current status and future perspectives. Jpn J Clin Oncol 2018;48:103-14. [Crossref] [PubMed]
- Forner A, Llovet JM, Bruix J. Hepatocellular carcinoma. Lancet 2012;379:1245-55. [Crossref] [PubMed]
- Cheng AL, Kang YK, Chen Z, et al. Efficacy and safety of sorafenib in patients in the Asia-Pacific region with advanced hepatocellular carcinoma: a phase III randomised, double-blind, placebo-controlled trial. Lancet Oncol 2009;10:25-34. [Crossref] [PubMed]
- Yau T, Chan P, Epstein R, et al. Management of advanced hepatocellular carcinoma in the era of targeted therapy. Liver Int 2009;29:10-7. [Crossref] [PubMed]
- Llovet JM, Ricci S, Mazzaferro V, et al. Sorafenib in advanced hepatocellular carcinoma. N Engl J Med 2008;359:378-90. [Crossref] [PubMed]
- Dong H, Strome SE, Salomao DR, et al. Tumor-associated B7-H1 promotes T-cell apoptosis: a potential mechanism of immune evasion. Nat Med 2002;8:793-800. [Crossref] [PubMed]
- Sheppard KA, Fitz LJ, Lee JM, et al. PD-1 inhibits T-cell receptor induced phosphorylation of the ZAP70/CD3zeta signalosome and downstream signaling to PKCtheta. FEBS Lett 2004;574:37-41. [Crossref] [PubMed]
- Parry RV, Chemnitz JM, Frauwirth KA, et al. CTLA-4 and PD-1 receptors inhibit T-cell activation by distinct mechanisms. Mol Cell Biol 2005;25:9543-53. [Crossref] [PubMed]
- Wang S, Hao J, Wang H, et al. Efficacy and safety of immune checkpoint inhibitors in non-small cell lung cancer. Oncoimmunology 2018;7:e1457600. [Crossref] [PubMed]
- Bellmunt J, Powles T, Vogelzang NJ. A review on the evolution of PD-1/PD-L1 immunotherapy for bladder cancer: The future is now. Cancer Treat Rev 2017;54:58-67. [Crossref] [PubMed]
- Suzman DL, Agrawal S, Ning YM, et al. FDA Approval Summary: Atezolizumab or Pembrolizumab for the Treatment of Patients with Advanced Urothelial Carcinoma Ineligible for Cisplatin-Containing Chemotherapy. Oncologist 2019;24:563-9. [Crossref] [PubMed]
- Ansell SM, Lesokhin AM, Borrello I, et al. PD-1 blockade with nivolumab in relapsed or refractory Hodgkin’s lymphoma. N Engl J Med 2015;372:311-9. [Crossref] [PubMed]
- Kasamon YL, de Claro RA, Wang Y, et al. FDA Approval Summary: Nivolumab for the Treatment of Relapsed or Progressive Classical Hodgkin Lymphoma. Oncologist 2017;22:585-91. [Crossref] [PubMed]
- Nghiem PT, Bhatia S, Lipson EJ, et al. PD-1 Blockade with Pembrolizumab in Advanced Merkel-Cell Carcinoma. N Engl J Med 2016;374:2542-52. [Crossref] [PubMed]
- El-Khoueiry AB, Sangro B, Yau T, et al. Nivolumab in patients with advanced hepatocellular carcinoma (CheckMate 040): an open-label, non-comparative, phase 1/2 dose escalation and expansion trial. Lancet 2017;389:2492-502. [Crossref] [PubMed]
- Cheng H, Sun G, Chen H, et al. Trends in the treatment of advanced hepatocellular carcinoma: immune checkpoint blockade immunotherapy and related combination therapies. Am J Cancer Res 2019;9:1536-45. [PubMed]
- Taube JM, Klein A, Brahmer JR, et al. Association of PD-1, PD-1 ligands, and other features of the tumor immune microenvironment with response to anti-PD-1 therapy. Clin Cancer Res 2014;20:5064-74. [Crossref] [PubMed]
- Rizk EM, Gartrell RD, Barker LW, et al. Prognostic and Predictive Immunohistochemistry-Based Biomarkers in Cancer and Immunotherapy. Hematol Oncol Clin North Am 2019;33:291-9. [Crossref] [PubMed]
- Bocanegra A, Fernandez-Hinojal G, Zuazo-Ibarra M, et al. PD-L1 Expression in Systemic Immune Cell Populations as a Potential Predictive Biomarker of Responses to PD-L1/PD-1 Blockade Therapy in Lung Cancer. Int J Mol Sci 2019;20:1631. [Crossref] [PubMed]
- Sasaki H, Moriyama S, Nakashima Y, et al. Histone deacetylase 1 mRNA expression in lung cancer. Lung Cancer 2004;46:171-8. [Crossref] [PubMed]
- Weichert W, Roske A, Gekeler V, et al. Association of patterns of class I histone deacetylase expression with patient prognosis in gastric cancer: a retrospective analysis. Lancet Oncol 2008;9:139-48. [Crossref] [PubMed]
- Weichert W, Roske A, Niesporek S, et al. Class I histone deacetylase expression has independent prognostic impact in human colorectal cancer: specific role of class I histone deacetylases in vitro and in vivo. Clin Cancer Res 2008;14:1669-77. [Crossref] [PubMed]
- Krusche CA, Wulfing P, Kersting C, et al. Histone deacetylase-1 and -3 protein expression in human breast cancer: a tissue microarray analysis. Breast Cancer Res Treat 2005;90:15-23. [Crossref] [PubMed]
- Halkidou K, Gaughan L, Cook S, et al. Upregulation and nuclear recruitment of HDAC1 in hormone refractory prostate cancer. Prostate 2004;59:177-89. [Crossref] [PubMed]
- Wang W, Gao J, Man XH, et al. Significance of DNA methyltransferase-1 and histone deacetylase-1 in pancreatic cancer. Oncol Rep 2009;21:1439-47. [PubMed]
- Woods DM, Sodre AL, Villagra A, et al. HDAC Inhibition Upregulates PD-1 Ligands in Melanoma and Augments Immunotherapy with PD-1 Blockade. Cancer Immunol Res 2015;3:1375-85. [Crossref] [PubMed]
- Dunn J, Rao S. Epigenetics and immunotherapy: The current state of play. Mol Immunol 2017;87:227-39. [Crossref] [PubMed]
- Xu D, Liu X, Wang Y, et al. Identification of immune subtypes and prognosis of hepatocellular carcinoma based on immune checkpoint gene expression profile. Biomed Pharmacother 2020;126:109903. [Crossref] [PubMed]
- Rosenberg JE, Hoffman-Censits J, Powles T, et al. Atezolizumab in patients with locally advanced and metastatic urothelial carcinoma who have progressed following treatment with platinum-based chemotherapy: a single-arm, multicentre, phase 2 trial. Lancet 2016;387:1909-20. [Crossref] [PubMed]
- Havel JJ, Chowell D, Chan TA. The evolving landscape of biomarkers for checkpoint inhibitor immunotherapy. Nat Rev Cancer 2019;19:133-50. [Crossref] [PubMed]
- Rossi A, Noia VD, Gkountakos A, et al. PD-L1 for selecting non-small-cell lung cancer patients for first-line immuno-chemotherapy combination: a systematic review and meta-analysis. Immunotherapy 2019;11:921-30. [Crossref] [PubMed]
- Brahmer J, Reckamp KL, Baas P, et al. Nivolumab versus Docetaxel in Advanced Squamous-Cell Non-Small-Cell Lung Cancer. N Engl J Med 2015;373:123-35. [Crossref] [PubMed]
- Mahoney KM, Atkins MB. Prognostic and predictive markers for the new immunotherapies. Oncology (Williston Park) 2014;28:39-48. [PubMed]