Inactivation of Wnt-LRP5 signaling suppresses the proliferation and migration of ovarian cancer cells
Introduction
Ovarian cancer (OCa) is one of the most common female malignant tumors with high mortality. It is the second most common female malignant tumor in terms of mortality (1). In the past 30 years, the 5-year survival rate of OCa patients is less than 30%. A major contributor to the high mortality rate is the fact that 70% of women with OCa initially manifests with little or no specific symptoms at the early stage. Therefore, patients present with metastases and invasion throughout the peritoneal cavity (2). Due to lack of effective early detection methods, OCa is often diagnosed as advanced disease clinically. While the mortality of OCa remains highest, the etiology of OCa is still elusive.
Conserved Wnt signaling is generally active during human development and growth activities, and plays an important role in physiological activities including embryonic development, organ growth and maintenance of various functions (3-6). Mutations in Wnt genes or components of the Wnt signaling pathway can cause various human diseases, such as developmental defects, and their abnormal functions can also cause cancer (7). In addition, there is growing evidence to show the cross-talk between Wnt signaling pathway and other signaling pathways including Notch (8) and Sonic Hedgehog (9) signaling pathways, which has implications for Wnt signaling pathway in therapeutic interventions of multiple cancers. As currently understood, Wnt proteins bind to receptors of the Frizzled proteins and lipoprotein receptor-related proteins 5/6 (LRP5/6) on the cell surface to further exert its physiological function (10). LRP5 is one member of low density lipoprotein receptor (LDLP) family and is found to be involved in fat metabolism mostly (11). As a co-receptor of the Wnt signaling pathway, LRP5 participates in the development of embryo (12), and especially the development of bone (13) and eye (14). Several line of evidence show the important physiological function of LRP5, for instance, LRP5 triggers osteoporosis-pseudo-glioma syndrome (OPPG), LRP5 is positively correlated with the activity of osteosarcoma cells (15), and mutations in LRP5 directly affect bone acquisition and eye development (16), cause insulin-dependent diabetes (17) and non-syndromic hearing loss (18).
Recently, LRP5 was found to be involved in multiple tumors progression though the exact mechanism is unknown. Rabbani et al. showed LRP5 regulated the process of epithelial-mesenchymal transition (EMT) and mediated the proliferation and migration of prostate cancer cells (19). Furthermore, LRP5 has proven to affect or directly interact with new tumor-associated factors such as STK40 (20), JAM3 (21), TG2 (22), Hsp90ab1 (23), in different cancer cells to regulate tumorigenesis and migration. In addition, a variety of natural products and synthetic compounds also exert their anticancer activity by acting on Wnt/LRP5 signaling (24,25), all of which suggests LRP5 could be a new anti-cancer target.
In our research, we have immediate study the role of Wnt-LRP5 signaling pathway in OCa progression by transfection of the dominant negative plasmid of LRP5 (DN-LRP5) into highly metastatic human OCa cells HO8910PM. The effect of transfection of DN-LRP5 plasmid and abrogation of Wnt-LRP5 pathway was examined on OCa cells characteristics in vitro and on mouse tumor growth in vivo. We present the following article in accordance with the ARRIVE reporting checklist (available at http://dx.doi.org/10.21037/tcr-20-3462).
Methods
Cell culture
Human liver cell line (L02, Chang Liver), human liver cancer cell lines (HepG2, HepG2.2.1.5, Hep3B, 7721, PLC, Huh7, 97-H, LM3, FOCUS, SNU398, sk-hep-1), human colorectal cancer cell lines (LOVO, SW480, HCT116, SW620), human OCa cells (A2780, HO8910, SK-OV-3, HO8910PM), human lung cancer cell lines (H460, H446), human embryonic kidney cell line (HEK293T) were from the American Type Culture Collection and maintained in Dulbecco’s Modified Eagle’s Medium (DMEM) with 10% fetal bovine serum (FBS). HO8910PM was infected with DN-LRP5 lentiviral and selected with puromycin to obtain the stable clone of HO8910PM/DN-LRP5, then maintained in DMEM with 10% FBS and puromycin for future experiments.
MTT assay
The logarithmic growth phase HO8910PM and HO8910PM/DN-LRP5 cells were plated to 96-well culture plates with a density of 104 cells/mL in medium containing 10% FBS. MTT was added to the cell culture at specific time points with a final concentration of 1 mg/mL and incubated at 37 °C for another 3 h. The optical absorbance at 570 nm was measured to determine the change of cell density (26).
Western blotting analysis
Protein lysates (20–80 µg) were obtained by lying HO8910PM and HO8910PM/DN-LRP5 cells, and then boiled at 100 °C for 5–10 minutes and resolved by 10% SDS-PAGE. Proteins were transferred to PVDF membranes, and sealed with 5% skim milk, the membranes were treated with specific primary antibodies. Then horseradish peroxidase-conjugated secondary antibodies were used to treat the blots for 1h. The blots were visualized through enhanced chemiluminescence.
Wound-healing analysis
HO8910PM and HO8910PM/DN-LRP5 cells were plated to a 6-well culture plates with a density of 106 cells/mL. After adherence, draw a vertical parallel wound on the cell layer using a p10 tip, then the medium was removed and cells were rinsed 3 times with sterile PBS to remove shed cells and cell debris. Proliferation of the cells in DMEM medium containing 2% FBS were measured to assay the rate of wound healing (27).
Transwell migration assay
2×104 cells were mixed and added to the inside of the transwell, and the transwell was placed in a 24-well plate containing 500 µL of complete medium, and cells were cultured for another 24 hours. Then the transwell was fixed with methanol for 25 min, stained with crystal violet for 45 min and washed with PBS three times, and the cell layer was gently scraped off with a medical cotton swab. Add 200 µL of sterile water or PBS to the inside of the transwell after cleaning. Photograph the transwell with an inverted microscope and count the number of cells (28).
Actin staining
HO8910PM and HO8910PM/DN-LRP5 cells were grown on coverslips for 24 hours, then fixed with Ready-made 4% paraformaldehyde at room temperature for 10 minutes, diluted Triton X-100 with PBS to a final concentration of 0.1%, and then penetrated the cells 20 minutes, then fix with 5% bovine serum albumin (BSA) for 1 hour, and stain with FITC-Phalloidin for 2 hours at room temperature and dark conditions. After washing twice with PBS, it was counter-stained with DAPI for 10 min. Finally, the distribution of F-actin (29) was observed by laser confocal microscope.
Animal protocols
Male BALB/C nude mice were grown to 6 weeks of age for tumor formation experiments. The experiment was divided into two groups. The experiment was performed under a project license [No. SYXK(Chuan)2014-189] granted by institutional ethics board of Southwest Jiaotong University, in compliance with Sichuan guidelines for the care and use of animals. The prepared cell suspensions HO8910PM and HO8910PM/DN-LRP5 (5×107 cells/mL) were aspirated under sterile conditions, and 100 µL of cell suspension was injected into the right neck of each nude mouse (5×106 cells/mouse). Each nude mouse was labeled and kept under sterile conditions. The lengths of the long and short axes of tumors were measured every two days, and the tumor volume was calculated according to the formula V = 0.5236 × L1 × L2 × L2 (where L1 is the long axis of the tumor, and L2 is the short axis of the tumor).
Statistical analysis
Data were presented as the mean ± SD. At least three independent experiments were performed. Comparisons of the experimental data were assessed using an independent two-sample t-test at P<0.05 level of significance.
Results
DN-LRP5 suppresses the proliferation of HO8910PM cells
In the previous study, we found LRP5 had significantly increased expression in highly metastatic colorectal cancer cells SW620 and OCa cells HO8910PM, while little expression in non-metastatic colorectal cancer cell line SW480 and OCa cell line HO8910 through western blot experiments (Appendix 1, Figure S1), which suggests that LRP5 may regulate cell migration and invasion. Then we constructed dominant negative LRP5 in HO8910PM cell line to explore the functions of LRP5 (Appendix 1, Figure S2), as expected, we observed that HO8910PM/DN-LRP5 cells grew much slower than that of HO8910PM. Further growth rate measurements by MTT assay indicated DN-LRP5 significantly inhibited the proliferation of HO8910PM cells (Figure 1).
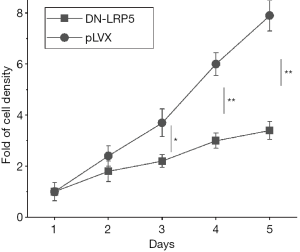
DN-LRP5 inhibits the migration of HO8910PM
To explore effects of DN-LRP5 on cell migration, HO8910PM and HO8910PM/DN-LRP5 cell lines were employed to perform the wound healing and the transwell experiments in a time course manner. The results showed the wound healing rate of HO8910PM cells was 93% at 48 h, which is much higher than a 32% of wound healing rate at 48 h for HO8910PM/DN-LRP5 cells (Figure 2A,B), indicating DN-LRP5 can significantly inhibit the migration of HO8910PM cells. Similarly, the transwell assay showed that HO8910PM/DN-LRP5 migrated much lower than HO8910PM, indicating that DN-LRP5 inhibited the migration of HO8910PM cells significantly with an inhibition rate of around 45% (Figure 2C,D).
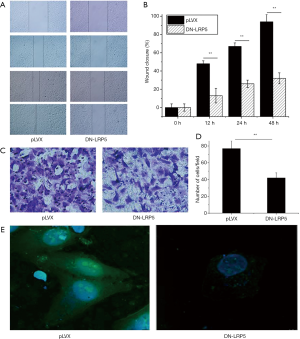
DN-LRP5 rearranges the cytoskeleton of HO8910PM
The arrangement of the cytoskeleton is closely related to the morphology and motility of the cells (30). Usually, the rearrangement of the cytoskeleton triggers changes in the structure of the microfilaments, resulting in an increase in the production of protrusive structures and an increase in the ability of tumor cells to move and migrate (31). As expected, HO8910PM cells had obvious protrusive structures (Figure 2E, left panel), showing high metastatic characteristics, while HO8910PM/DN-LRP5 showed no obvious protrusive structures, suggesting DN-LRP5 rearranged the cytoskeleton and inhibited the mobility of HO8910PM cells (Figure 2E, right panel).
DN-LRP5 regulates EMT process
EMT plays key roles in tumor metastasis. EMT molecular markers (for example, E-cadherin, Keratin 8/18, vimentin and fibronectin) are the hallmark events during EMT (32), accordingly, we detected the key molecules of EMT in both HO8910PM and HO8910PM/DN-LRP5 cells by western blotting, seeing the Appendix 1. As shown in Figure 3A,B and Figure S3A,B, HO8910PM/DN-LRP5 cells showed decreased expression of mesenchymal markers vimentin and fibronectin and increased expression of epithelial markers E-cadherin and Keratin 8/18, suggesting DN-LRP5 somehow reversed EMT process of HO8910PM cells and participated the regulation of cell migration.
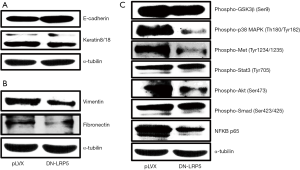
DN-LRP5 regulates multiple cancer-associated pathways
Wnt signaling pathway is often implicated in regulation of other cancer signaling pathways, for example, the HGF/c-Met (33), the PI3 Kinase/Akt (34), the MAPK (35) and NF-κB (36) pathways to play a pivot role in tumor formation and metastasis. With this context, we explored if DN-LRP5 has any effects on classical cancer signaling pathways including the Akt, p38, HGF/c-Met and NF-κB, seeing the Appendix 1. As shown in Figure 3C and Figure S3C, DN-LRP5 significantly down-regulated the activity of the Akt, p38, HGF/c-Met and NF-κB, while had little effects on the GSK3β, the Stat3 and the Smad3 signaling pathways, suggesting Wnt-LRP5 cross-talked with those signaling pathways by a synergy manner possibly though the detailed mechanisms are elusive.
DN-LRP5 inhibits tumor proliferation of nude mice
Next, we were concerned about the effectiveness of DN-LRP5 on tumor inhibition in nude mice. With this purpose, nude mice were injected with HO8910PM and HO8910PM/DN-LRP5 cells at the same amount of cells respectively. From the fourth day after injection, the tumor volume was recorded every 2 days. The results showed that DN-LRP5 significantly inhibited tumor growth in nude mice in a time-dependent manner, with an inhibition rate high to around 90% eventually (Figure 4).
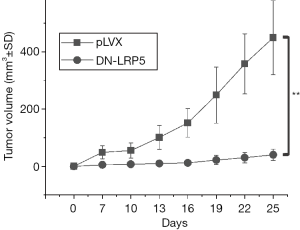
Discussion
The Wnt signaling pathway is super complex, containing 19 different Wnt ligands, 10 Frizzled receptors, several helper receptors, and numerous downstream target proteins, which have been evaluated by researchers for many years (37). Even though, the Wnt signaling pathway can be broadly classified into two categories, the canonical Wnt/β-catenin pathway and non-canonical pathways, such as the Wnt/Ca2+ pathway, the Wnt/PCP pathway, and the specificity of each pathway depends on different cell types, WNTs, SFRPs, WIF1, DKKs, Frizzled, co-receptors (LRP5/6), and regulation of Wnt signaling regulators in the cytoplasm (38). Except the developmental function of Wnt signaling pathway, recent studies have shown that inhibition of Wnt signaling activity can significantly inhibit the growth of tumor cells such as bladder cancer, rectal cancer, and liver cancer (39) though the detailed mechanisms are elusive. Here, we found Wnt-LRP5 is involved in progression of OCa cells, which adds a new possibility for OCa detection and treatment by targeting this classical signaling pathway.
As a co-receptor of Wnt signaling pathway, LRP5 was thought to be involved in the regulation of development (for example, bone and eye development) mostly in previous research. The role of LRP5 in tumors regulation was rarely reported. In terms of the specific role of LRP5 in tumor cells, studies have shown that the dominant negative mutant of LRP5 overexpressing in prostate cancer PC-3 cells can inhibit the infiltration and migration of tumor cells and formation of subcutaneous xenograft tumors in nude mice, and the main mechanism is by up-regulating E-cadherin expression and inhibiting MMPs activity (40). After that, overexpression of DN-LRP5 also significantly inhibited the infiltration and migration of osteosarcoma cells HT1080 and SW872, as well as tumorigenicity in nude mice, via down-regulation of hepatocyte growth factor receptor c-Met expression and inhibition of c-Met-mediated activity (41). In our current study, we found DN-LRP5 significantly inhibited the proliferation and migration of OCa cells in vitro (Figure 1) and tumor formation of mouse in vivo (Figure 4), our data plus the results from other group suggests LRP5 could be a new anti-tumor target.
In addition to the migration assay in vitro, the invasive phenotype of OCa may need to change the molecular structure from epithelial cells to mesenchymal cells. Down regulation of E-cadherin and up regulation of N-cadherin are associated with OCa progression, which is a common phenomenon in EMT sites during normal development and cancer (42). In our study, suppressing LRP5 expression in HO8910PM cells weakened cellular mobility, accompanying an obviously upregulation of epithelial markers E-cadherin and Keratin8/18 (Figure 3A) as well as downregulation of mesenchymal markers fibronectin and vimentin (Figure 3B), similar to the reversal of EMT (43). In summary, the reversal of EMT and the migration and actin staining assay in vitro suggested that LRP5 affected migration of OCa cells.
Activated phospho-Akt could promote cell proliferation via exerting anti-apoptotic effects through multiple downstream pathways including mTOR, Bcl-2, Caspase-9, NF-κB (34). Similarly, phosphorylation activation of p38 MAPK (35) and c-Met (33) and combination of NF-κB and p65 (36) could activate the corresponding downstream pathway to promote cell proliferation and migration. The western blot results suggested that DN-LRP5 prevented phosphorylation activation of Akt, p38 MAPK and c-Met and combination of NF-κB and p65 to inhibit cell proliferation and migration. This indicates that Wnt/LRP5 can cross talk with HGF/c-Met, Akt, p38 MAPK and NF-κB signaling pathways to regulate cell proliferation and migration.
Our study successfully established a stable expression cell line of DN-LRP5 in OCa cells and proved that DN-LRP5 has a certain inhibitory effect on the growth and migration of OCa cells. This lays a foundation for further exploration of the anti-OCa and molecular mechanisms of DN-LRP5, thus building a framework for the development of anti-tumor drugs against Wnt signaling pathway.
Acknowledgments
Funding: This work was supported by
Footnote
Reporting Checklist: The authors have completed the ARRIVE reporting checklist. Available at http://dx.doi.org/10.21037/tcr-20-3462
Data Sharing Statement: Available at http://dx.doi.org/10.21037/tcr-20-3462
Conflicts of Interest: All authors have completed the ICMJE uniform disclosure form (available at http://dx.doi.org/10.21037/tcr-20-3462). The authors have no conflicts of interest to declare.
Ethical Statement: The authors are accountable for all aspects of the work in ensuring that questions related to the accuracy or integrity of any part of the work are appropriately investigated and resolved. Experiments were performed under a project license [No. SYXK(Chuan)2014-189] granted by institutional ethics board of Southwest Jiaotong University, in compliance with Sichuan guidelines for the care and use of animals.
Open Access Statement: This is an Open Access article distributed in accordance with the Creative Commons Attribution-NonCommercial-NoDerivs 4.0 International License (CC BY-NC-ND 4.0), which permits the non-commercial replication and distribution of the article with the strict proviso that no changes or edits are made and the original work is properly cited (including links to both the formal publication through the relevant DOI and the license). See: https://creativecommons.org/licenses/by-nc-nd/4.0/.
References
- Siegel RL, Miller KD, Jemal A. Cancer Statistics, 2017. CA Cancer J Clin 2017;67:7-30. [Crossref] [PubMed]
- Vaughan S, Coward JI, Bast RC Jr, et al. Rethinking ovarian cancer: recommendations for improving outcomes. Nat Rev Cancer 2011;11:719-25. [Crossref] [PubMed]
- Brafman D, Willert K. Wnt/beta-catenin signaling during early vertebrate neural development. Dev Neurobiol 2017;77:1239-59. [Crossref] [PubMed]
- Hussain M, Xu C, Lu M, et al. Wnt/beta-catenin signaling links embryonic lung development and asthmatic airway remodeling. Biochim Biophys Acta Mol Basis Dis 2017;1863:3226-42. [Crossref] [PubMed]
- Veltri A, Lang C, Lien WH. Concise Review: Wnt Signaling Pathways in Skin Development and Epidermal Stem Cells. Stem Cells 2018;36:22-35. [Crossref] [PubMed]
- Girardi F, Le Grand F. Wnt Signaling in Skeletal Muscle Development and Regeneration. Prog Mol Biol Transl Sci 2018;153:157-79. [Crossref] [PubMed]
- Nusse R, Clevers H. Wnt/beta-Catenin Signaling, Disease, and Emerging Therapeutic Modalities. Cell 2017;169:985-99. [Crossref] [PubMed]
- Collu GM, Hidalgo-Sastre A, Brennan K. Wnt-Notch signalling crosstalk in development and disease. Cell Mol Life Sci 2014;71:3553-67. [Crossref] [PubMed]
- Noubissi FK, Yedjou CG, Spiegelman VS, et al. Cross-Talk between Wnt and Hh Signaling Pathways in the Pathology of Basal Cell Carcinoma. Int J Environ Res Public Health 2018;15:1442. [Crossref] [PubMed]
- MacDonald BT, He X. Frizzled and LRP5/6 receptors for Wnt/beta-catenin signaling. Cold Spring Harb Perspect Biol 2012;4:a007880. [Crossref] [PubMed]
- Go GW, Mani A. Low-density lipoprotein receptor (LDLR) family orchestrates cholesterol homeostasis. Yale J Biol Med 2012;85:19-28. [PubMed]
- Chen Q, Zhang Y, Lu J, et al. Embryo-uterine cross-talk during implantation: the role of Wnt signaling. Mol Hum Reprod 2009;15:215-21. [Crossref] [PubMed]
- Kato M, Patel MS, Levasseur R, et al. Cbfa1-independent decrease in osteoblast proliferation, osteopenia, and persistent embryonic eye vascularization in mice deficient in Lrp5, a Wnt coreceptor. J Cell Biol 2002;157:303-14. [Crossref] [PubMed]
- Fujimura N. WNT/beta-Catenin Signaling in Vertebrate Eye Development. Front Cell Dev Biol 2016;4:138. [Crossref] [PubMed]
- Hoang BH, Kubo T, Healey JH, et al. Dickkopf 3 inhibits invasion and motility of Saos-2 osteosarcoma cells by modulating the Wnt-beta-catenin pathway. Cancer Res 2004;64:2734-9. [Crossref] [PubMed]
- Gong Y, Slee RB, Fukai N, et al. LDL receptor-related protein 5 (LRP5) affects bone accrual and eye development. Cell 2001;107:513-23. [Crossref] [PubMed]
- Ferrari SL, Deutsch S, Choudhury U, et al. Polymorphisms in the low-density lipoprotein receptor-related protein 5 (LRP5) gene are associated with variation in vertebral bone mass, vertebral bone size, and stature in whites. Am J Hum Genet 2004;74:866-75. [Crossref] [PubMed]
- Xia W, Hu J, Liu F, et al. New role of LRP5, associated with nonsyndromic autosomal-recessive hereditary hearing loss. Hum Mutat 2017;38:1421-31. [Crossref] [PubMed]
- Rabbani SA, Arakelian A, Farookhi R. LRP5 knockdown: effect on prostate cancer invasion growth and skeletal metastasis in vitro and in vivo. Cancer Med 2013;2:625-35. [Crossref] [PubMed]
- Maubant S, Tahtouh T, Brisson A, et al. LRP5 regulates the expression of STK40, a new potential target in triple-negative breast cancers. Oncotarget 2018;9:22586-604. [Crossref] [PubMed]
- Zhang Y, Xia F, Liu X, et al. JAM3 maintains leukemia-initiating cell self-renewal through LRP5/AKT/beta-catenin/CCND1 signaling. J Clin Invest 2018;128:1737-51. [Crossref] [PubMed]
- Ayinde O, Wang Z, Griffin M. Tissue transglutaminase induces Epithelial-Mesenchymal-Transition and the acquisition of stem cell like characteristics in colorectal cancer cells. Oncotarget 2017;8:20025-41. [Crossref] [PubMed]
- Wang H, Deng G, Ai M, et al. Hsp90ab1 stabilizes LRP5 to promote epithelial-mesenchymal transition via activating of AKT and Wnt/β-catenin signaling pathways in gastric cancer progression. Oncogene 2019;38:1489-507. [Crossref] [PubMed]
- Shi X, Zhu M, Kang Y, et al. Wnt/beta-catenin signaling pathway is involved in regulating the migration by an effective natural compound brucine in LoVo cells. Phytomedicine 2018;46:85-92. [Crossref] [PubMed]
- Dai B, Ma Y, Yang T, et al. Synergistic effect of berberine and HMQ1611 impairs cell proliferation and migration by regulating Wnt signaling pathway in hepatocellular carcinoma. Phytother Res 2019;33:745-55. [Crossref] [PubMed]
- van Meerloo J, Kaspers GJ, Cloos J. Cell sensitivity assays: the MTT assay. Methods Mol Biol 2011;731:237-45. [Crossref] [PubMed]
- Bedoya C, Cardona A, Galeano J, et al. Accurate Region-of-Interest Recovery Improves the Measurement of the Cell Migration Rate in the In Vitro Wound Healing Assay. SLAS Technol 2017;22:626-35. [Crossref] [PubMed]
- Marshall J. Transwell((R)) invasion assays. Methods Mol Biol 2011;769:97-110. [Crossref] [PubMed]
- Chazotte B. Labeling cytoskeletal F-actin with rhodamine phalloidin or fluorescein phalloidin for imaging. Cold Spring Harb Protoc 2010;2010:pdb.prot4947.
- Olson MF, Sahai E. The actin cytoskeleton in cancer cell motility. Clin Exp Metastasis 2009;26:273-87. [Crossref] [PubMed]
- Alblazi KM, Siar CH. Cellular protrusions--lamellipodia, filopodia, invadopodia and podosomes--and their roles in progression of orofacial tumours: current understanding. Asian Pac J Cancer Prev 2015;16:2187-91. [Crossref] [PubMed]
- Nieto MA, Huang RY, Jackson RA, et al. EMT: 2016. Cell 2016;166:21-45. [Crossref] [PubMed]
- Wozney JL, Antonarakis ES. Growth factor and signaling pathways and their relevance to prostate cancer therapeutics. Cancer Metastasis Rev 2014;33:581-94. [Crossref] [PubMed]
- Mabuchi S, Kuroda H, Takahashi R, et al. The PI3K/AKT/mTOR pathway as a therapeutic target in ovarian cancer. Gynecol Oncol 2015;137:173-9. [Crossref] [PubMed]
- Burotto M, Chiou VL, Lee JM, et al. The MAPK pathway across different malignancies: a new perspective. Cancer 2014;120:3446-56. [Crossref] [PubMed]
- Christian F, Smith EL, Carmody RJ. The Regulation of NF-kappaB Subunits by Phosphorylation. Cells 2016;5:12. [Crossref] [PubMed]
- Wodarz A, Nusse R. Mechanisms of Wnt signaling in development. Annu Rev Cell Dev Biol 1998;14:59-88. [Crossref] [PubMed]
- Clevers H, Nusse R. Wnt/beta-catenin signaling and disease. Cell 2012;149:1192-205. [Crossref] [PubMed]
- Hernandez-Maqueda JG, Luna-Ulloa LB, Santoyo-Ramos P, et al. Protein kinase C delta negatively modulates canonical Wnt pathway and cell proliferation in colon tumor cell lines. PLoS One 2013;8:e58540. [Crossref] [PubMed]
- Zi X, Guo Y, Simoneau AR, et al. Expression of Frzb/secreted Frizzled-related protein 3, a secreted Wnt antagonist, in human androgen-independent prostate cancer PC-3 cells suppresses tumor growth and cellular invasiveness. Cancer Res 2005;65:9762-70. [Crossref] [PubMed]
- Guo Y, Xie J, Rubin E, et al. Frzb, a secreted Wnt antagonist, decreases growth and invasiveness of fibrosarcoma cells associated with inhibition of Met signaling. Cancer Res 2008;68:3350-60. [Crossref] [PubMed]
- Hazan RB, Qiao R, Keren R, et al. Cadherin switch in tumor progression. Ann N Y Acad Sci 2004;1014:155-63. [Crossref] [PubMed]
- Kang Y, Massague J. Epithelial-mesenchymal transitions: twist in development and metastasis. Cell 2004;118:277-9. [Crossref] [PubMed]