Angiogenic effect of lysophosphatidic acid receptors on cervical cancer cells
Introduction
Cervical cancer is a common solid tumor malignancy (1-7), with racial/ethnic, socioeconomic, and geographical disparities in incidence and mortality (8-20). A 2015 study reported that in 2012 there were an estimated 527,600 new cases and 265,700 deaths worldwide from cervical cancer, which has the fourth highest incidence rate and is the fourth leading cause of cancer death in women (21). In a study using data from the Surveillance, Epidemiology, and End Results Program, after adjusting for confounding factors such as race, marital status, stage, age, treatment, grade, and histology, for cervical cancer there was a significant difference in specific mortality from 1985 to 1989 and from 1990 to 1994 (but not after 1995) (18). Cervical cancer’s high incidence rate and its persistently high mortality highlight its importance as a woman’s health issue.
The standard treatment for cervical cancer’s early stages is radical hysterectomy, with more conservative therapies being used for younger patients (22-26). For locally advanced stages, concomitant chemotherapy and radiotherapy are used (27-32). Recurrent and metastatic disease are treated usually by palliative platinum-based chemotherapy, which possesses a limited utility and can cause significant adverse side-effects (33-38). For advanced cases, research dedicated to molecular targeted therapies has become a promising means of seeking out novel agents that improve patient prognosis and reduce side effects (39-41).
Clinical evidence of angiogenesis in cervical cancer
Formation of new blood vessels is crucial for tumor growth, progression and metastasis. Within the growing tumor, angiogenesis is required for formation of new blood vessels and for recruiting and sustaining its blood supply (42,43). The progression from cervical cancer precursor (CIN) lesion to invasive carcinoma can be a process of converting dormant tumors. Clinical observations have shown that angiogenesis occurs in both pre-invasive and invasive cervical cancers and are linked to clinical symptoms such as spontaneous bleeding and easy bleeding upon contact (44-47).
Angiogenesis is connected with diagnosis. Preinvasive and invasive cervical cancers present distinct features—i.e., microvessel growth along with persistent cell production—which clinically can be directly visualized by colposcopy after magnification (48,49). In correspondence with these colposcopic findings, histological examination of tumor sections has revealed that malignant cervical cancer cells are surrounded by highly tortuous vessels with no uniform direction or branching and by irregularly formed vascular spaces (50). Moreover, during the progression from noninvasive to microinvasive cervical carcinoma, microvessel density (MVD)—a measure of tumor angiogenesis—has been reported as increasing significantly (51). In fact, the different vascular patterns which present themselves between pre-invasive and invasive cancer can be used for pre-treatment differential diagnosis (52). Such angiogenic patterns make cervical cancer one of the most effectively diagnosed of all cancers.
Using 3-dimensional power Doppler angiography, tumor vascularity assessed for cases of cervical cancer all showed intratumoral blood flow (53). Ultrasound also showed a chaotic network of tortuous vessels traversing the cervical cancer tumor mass (54). A significant positive correlation between tumor vascularization and cervical volume has also been found (55).
Angiogenesis is also linked with prognosis. It may affect survival in both early- and advanced-stage cervical cancer patients. Up-regulation of angiogenic factors correlated with severity of CIN lesions and invasive disease (56). In cervical cancer, the angiogenic factors angiogenin, endoglin and endostatin show a definite relationship with disease stage (57). Among 215 healthy subjects and 199 early cervical cancer patients who had been treated with surgical resection, Kim et al. found that polymorphisms of vascular endothelial growth factor (VEGF) genes may affect cancer susceptibility and survival in cases of early cervical cancer by modulating tumor angiogenesis (58). MVD is an independent prognostic parameter for recurrence-free survival in patients with early stage cervical cancer who undergo radical hysterectomy with pelvic lymph node dissection; MVD at or above the cut-off point of nine vessels per high power field had significantly poorer recurrence-free survival (59). In eighty-seven patients with cervical cancer who underwent definitive radiotherapy with a combination of external beam radiotherapy (45-50.4 Gy) and high-dose-rate brachytherapy (5×7 Gy), Dunst et al. found that poorly oxygenated tumors had a significantly increased MVD, a fact which had an overwhelming impact on local failure rate and survival (60). Higher tumor vascularity was associated with lower overall survival (OS) and locoregional control in carcinoma of the cervix treated with radiotherapy (61). Randal et al. used semi-quantitative immunohistochemical staining to examine cervical cancer tissue for VEGF, thrombospondin-1 (TSP-1), CD31 and CD105. They found that high levels of CD31 MVD, but not TSP-1, VEGF or CD105 MVD, was an independent prognostic factor for progression free survival. Tumor angiogenesis measured by CD31 MVD was an independent prognostic factor for cervical cancer (62). In 166 patients with stage IB cervical cancer treated primarily by radical hysterectomy and bilateral lymphadenectomy, high MVD was found to be an independent prognostic factor which adversely influenced patients’ survival (63).
Histological evidence of angiogenesis in cervical cancer
Angiogenesis in cervical cancer can be directly evaluated by microvessel immunohistological staining and counted under a microscope (64). Table 1 shows studies that investigated both angiogenesis and MVD in cervical cancer.
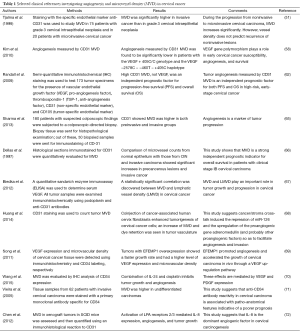
Full table
In cervical cancer, histological sections immunostained for CD31 were quantitatively evaluated for MVD (51,58,62,65-68). Besides CD31, CD34 was the other frequently used angiogenesis marker (69-71). In a nude mice model study, it was shown that a combination of interleukin (IL)-24 and cisplatin inhibited tumor growth and angiogenesis and that these effects were mediated by downregulation of both VEGF and platelet-derived growth factor (PDGF) expression (70).
For MVD, a statistically significant difference has been found between poorly differentiated and well-differentiated carcinomas (73). A comparison of microvessel counts from normal epithelium with those from CIN and invasive carcinoma showed a significant increase in MVD expression in precancerous lesions and invasive cancer.
Angiogenesis is an early event in premalignant changes of the cervix. A comparison of MVD levels between low- and high-grade pre-invasive cervical lesions revealed a statistically significant increase in the more advanced lesions (73,74). Large vascular structures were noted in the upper layers of the epithelium with neovascularization of stromal vascular papillae protruding toward the epithelial surface; clinically this is connected with the colposcopic finding of an abnormal vascular image of dysplastic lesions. The amount of angiogenesis appeared to be independent of the human papillomavirus (HPV) type (75).
Lysophosphatidic acid (LPA) concentrations
LPA is a naturally occurring, potent lysolipid present in human blood. LPA’s sources include plasma lipoproteins (76), cancer cells (77-79), fibroblasts (80), adipocytes (81-83), peritoneal mesothelial cells (84), and activated platelets (85,86). The measurement of total LPA levels can be performed by radioenzymatic, fluorometric, colorimetric, or immunoenzymatic assay. However, determination of LPA molecular species requires the use of techniques that include capillary electrophoresis, gas chromatography, thin layer chromatography, liquid chromatography, matrix-assisted laser desorption/ionization, and electrospray ionization mass spectrometry (ESI-MS) (87-98). The best method is ESI-MS, which can measure LPA species without interference from other compounds (88-90,93,94,96,98).
Meleh et al. used ESI-MS to determine LPA levels and reported that LPA’s mean level in the sera of 50 healthy controls was 2.9 µM (99). In a study of 10 controls (8 healthy women and 2 women with benign gynecologic disease), Xiao et al. used ESI-MS to show that the plasma LPA levels were below 2.0 µM (96). Using an enzymatic cycling assay, Hosogaya et al. reported that in healthy subjects LPA’s mean physiological level in women was higher than that in men (76). Using gas chromatography analysis, Xu et al. found that for 48 healthy controls the mean LPA plasma level was 0.6 µM (range, <0.1-6.3 µM) (95).
LPA concentrations were found to be significantly elevated in the sera from ovarian, cervical, and some endometrial and peritoneal cancer patients and in the ascites from ovarian cancer patients (94-104). It has been suggested that LPA is a potential marker for the screening, diagnosing, and monitoring of ovarian cancer (95,99,100). Xu et al., using gas chromatography analysis, found that 48 ovarian cancer patients had significantly higher plasma LPA levels (mean 8.6 µM) when compared with 48 healthy controls (95). Using ESI-MS, Xiao et al. later confirmed that LPA was elevated in blood specimens from 8 patients with ovarian cancer and one with endometrial cancer (96). Gas chromatography analysis was used to discover that different LPA species were associated with late-stage or recurrent ovarian cancer (97). A meta-analysis done by Li et al. of a total of 980 ovarian cancer patients, 872 benign controls and 668 healthy controls revealed that LPA plasma levels in ovarian cancer patients were significantly higher than in benign controls and healthy controls (101). Using ESI-MS analysis, Meleh et al. reported that the mean LPA level for 50 ovarian cancer patients and for 65 women with benign ovarian tumor was 8.4 and 8.0 µM, respectively, and that the cut off value for the presence of ovarian tumors was 3.9 µM (99).
When electrogenerated chemiluminescence was used to analyze LPA concentrations in plasma, LPA levels from 135 cervical cancer patients (5.11±1.92 µM) were found to be significantly higher than those from 40 healthy controls (2.31±0.45 µM) (103). After using gas chromatography analysis, Xu et al., reported that in 6 cervical cancer patients the mean level for total plasma LPA was 21.9 µM (95). However, ESI-MS is rarely reported as being used to discern LPA species in cervical cancer, and thus further studies are needed.
In instances where liquid chromatography/mass spectroscopy were used to study peritoneal effusion, LPA concentrations were found to be higher in the peritoneal effusions from 10 ovarian cancer patients than in those from 22 nonmalignant patients (102). In peritoneal fluid analyzed using a neurite retraction bioassay, LPA-equivalent levels were 50.2 µM (range, 5.4-200 µM) for all 62 patients and 94.5 µM (range, 15-200 µM) for 13 ovarian cancer patients (104). Using ESI-MS, Xiao et al. also found that LPA in the ascitic fluid from 15 patients with ovarian cancer (mean 18.9 µM) was higher than that found in the fluid from 15 patients with benign liver disease (mean 2.9 µM) (98).
Overall, LPA concentrations were found to be higher in blood specimens from cervical or ovarian cancer patients than in those from healthy controls, and higher in ascitic fluid from malignancies than from non-malignancies.
Autotaxin (ATX) as a LPA-producing enzyme
LPA is present in both cells and biological fluids. In serum or plasma, LPA is produced predominantly by a plasma enzyme called ATX (105-107). ATX (nucleotide pyrophosphatase-phosphodiesterase 2), a secreted lysophospholipase D (lysoPLD) present in serum, is an enzyme that catalyzes the hydrolysis of lysophosphatidylcholine (LPC) into LPA (108). There are two LPA production pathways which convert phospholipds to LPA: (I) the PLA1/PLA2-lysoPLD pathway, where lysophospholipids (LPLs) that are generated by a phospholipase A1 (PLA1) or PLA2 reaction are subsequently converted to LPA by a lysoPLD reaction; and (II) the PLD-PLA1/PLA2 pathway, where phosphatidic acid generated by a phospholipase D or diacylglycerol kinase reaction is subsequently converted to LPA by a PLA1 or PLA2 reaction (106). In blood plasma, LPC is the most abundant phospholipid; ATX is considered the major LPA-producing enzyme in human blood, one which produces the most extracellular LPA (109-111).
In healthy subjects, Hosogaya et al. found that plasma LPA concentrations strongly correlated with lysoPLD activity. Lipid-related parameters other than LPC correlated only slightly or did not correlate with the LPA concentration, which suggests that conversion to LPC by lysoPLD might be the major route for LPA production in plasma (76). Although there are other LPA-producing pathways independent of ATX, a strong association between cancer cells and ATX production has been observed. Accumulating evidence for the physiological and pathological functions of ATX strongly support the claim that ATX is an important and promising therapeutic target (112,113).
During mice development, van Meeteren et al. noted that ATX was essential for blood vessel formation (114). In 50 cultured human tumor cell lines derived from various tumors (CNS, lung, breast, stomach, colon, kidney, ovary, prostate, cervix, fibroblast, and melanoma), Kishi et al. found that some cells expressed a significant amount of ATX at both the mRNA and protein levels. Both ATX protein and lysoPLD activity were detected in the culture supernatants. The highest ATX expression was in SNB-78 cells. Most ATX protein was detected in culture cell supernatant, whereas only a small amount was detected in cells. These results confirm that ATX is secreted by cancer cells (115).
ATX may promote cell migration, metastasis, and angiogenesis, and has been intimately linked with cancer development. According to Umezu-Goto et al., a variety of cancer cell lines (A-2058, CHO-K1, MDA-MB-231, parental RH7777 and RH7777-EDG2 cells) release significant amounts of LPC, a substrate for ATX, into the culture medium (108). Research on the use of ATX inhibitors, both as a primary and as an adjuvant therapy, is accelerating (110). After oral administration to mice of 3 mg/kg of FTY720, a competitive inhibitor of ATX, significantly reduced plasma LPA levels were noted (116). Murph et al. found that in melanoma, vinyl sulfone analogs of LPC irreversibly inhibited ATX and prevented angiogenesis in melanoma (117). Benesch et al. discovered that inhibition of ATX with a new ATX inhibitor, ONO-8430506, delayed breast tumor growth and lung metastasis in BALB/c mice (111).
As was the case for LPA, high expression of ATX in cancers is also associated with increased tumor progression, angiogenesis and metastasis (109). The ATX–LPA signaling axis has emerged as an important factor in many types of cancer. These results thus suggest that ATX-targeting strategies may provide a novel therapeutic approach to the inhibition of cervical cancer angiogenesis.
Functions mediated by LPA
LPA mediates a variety of cellular responses. Its physiologic and pathologic functions include the promotion of cell proliferation (118-131), cell survival (124,132-139), cell apoptosis (140-150), cell motility (91,115,151-158), cell migration (119,122,128,150,158-178), cell shape (179,180), cell differentiation (83,125,134,140,169,181-187), gene expression (188-202), cell transformation (203,204), tumorigenesis (139,205-209), cell invasion and metastasis (124,166,170,210-220) and other cell processes. LPA also enhances angiogenesis (72,118,221-229).
LPA receptors in cancer cells
The cellular responses elicited by LPA are produced by signaling through at least six G-protein coupled receptors (GPCRs) (230-243). LPA receptors were originally defined as an endothelial differentiation gene (Edg) family of GPCRs. However, at present LPA’s GPCRs are thought of as being divisible into two families: the Edg family (106,205,233,241,244-262) and the P2Y family (78,106,205,236,238,240,243,262, 263).
The Edg-family LPA receptors consist of LPA1/Edg2 (247,251,252,255,256,259-262,264), LPA2/Edg4 (230,258,262,265), and LPA3/Edg7 (231,253,262). The p2y-family LPA receptors are the newly discovered GPCRs for LPA, which include LPA4/GPR23/p2y9 (240,262), LPA5/GPR92/93 (235,262), LPA6/p2y5 (236,242,262), GPR87/95 (243,262) and p2y10 (238,262). Among the p2y-family, LPA4, LPA5 and LPA 6 are purinergic receptors. The six protein receptors that interact with LPA were named LPA1-LPA6, with italicized gene names LPAR1-LPAR6 representing human genes and Lpar1-Lpar6 representing non-human genes (266).
LPA receptor expression (LPA1, LPA2, LPA3, and LPA4) has been examined in 50 tumor cell lines from various origins by using quantitative RT-PCR; results revealed that LPA1 was dominant in brain tumor cells, LPA2 was predominantly expressed in cells from colon, stomach, and breast cancers, and LPA3 was expressed in certain ovarian and prostate cancer cell lines. Overall, expression of LPA4 was fairly low (115).
Each LPA receptor in cancer cells has also been studied in the context of their respective cell lines. Up-regulation of LPA1 that promoted pathological functions in cancer cells was found in human pancreatic cancer cell lines (BxPC-3, CFPAC-1, PANC-1, PK-1, YAPC-PD and YAPC cells) (154,156,267), a breast cancer cell line (MDA-MB-231 cells) (268,269), gastric cancer cell lines (AGS, MKN1, MKN28 and MKN74 cells) (269-271), a colon cancer cell line (DLD1 cells) (270), ovarian cancer cell lines (A2780CP, Caov-3, Dov-13, HEY, OVCA432, OVCAR-3 and SKOV-3) (272-275), a melanoma cell line (B16F10 cells) (220), and prostate cancer cell lines (LNCaP, LNCaP-LPA1 and PC3 cells) (130,276-278).
LPA2 expression was found in colon cancer cell lines (CaCo-2, DLD1, HCT116, LS174T, LoVo, SW48 and SW480 cells) (196,270,279-283), ovarian cancer cell lines (3AO, A2780, A2780CP, CaOV-3, DOV-13, HEY, HOC-1, OCC-1,OVCA 420, OVCA 429, OVCA 432, OVCA 433, OVCAR-3 and SKOV3 cells) (152,272-274,284-286), pancreatic cancer cell lines (BxPC-3, CFPAC-1, HPD-K2, PANC-1, PK-1 and YAPC-PD cells) (154,172,267), a gastric cancer cell line (AGS cells) (270), a prostate cancer cell line (PC-3 cells) (277), and thyroid cancer cells (287).
LPA3 expression was found in colon cancer cell lines (DLD1, HCT116, LS174T and SW480 cells) (270,282,283), pancreatic cancer cell lines (BxPC-3, CFPAC-1, PANC-1, PK-1 and YAPC-PD cells) (154,267), ovarian cancer cell lines (3AO, A2780CP, Caov-3, OVCAR-3, Dov-13, HEY, OVCA432 and SKOV-3 cells) (272-275,285), a gastric cancer cell line (AGS cells) (270), and a prostate cancer cell line (PC-3 cells) (277,278,288).
LPA4, LPA5 and LPA6 play diverse roles in the activation of tumor progression in pancreatic cancer cell lines (PANC-sh4, PANC-sh5 and PANC-sh6 cells) (289). LPA4 was expressed in both human colon cancer cell lines (CaCo-2, DLD1, HCT116, LoVo, SW48, and SW480 cells) (196,285) and a human ovarian cancer cell line (SKOV-3) (290). LPA5 was expressed in human colon cancer cell lines (CaCo-2, DLD1, HCT116, LoVo, SW48 and SW480 cells) (196). LPA5 was also expressed in a human melanoma cell line (B16F10 cells) (220). Like other known P2Y receptors, GPR87 was found to be located mainly on the cell surface, with the overexpression of GPR87 found preferentially in squamous carcinoma cells (291). The presence of other non-Edg and non-purinergic family LPA receptors in cancer cell lines has rarely been reported.
In contrast with studies of other cell lines, studies of LPA receptors in cell lines from cervical cancers are relatively scarce. A report from Chen et al. found that the LPA receptors expressed in cervical cancer cells are the Edg-family receptors LPA1, LPA2 and LPA3, which were noted as being up-regulated in cervical cancer cell lines (CaSki, HeLa and SiHa cells) (72). Glatt et al. found that up-regulation of both GPR87 transcript and protein was detectable in cervical cancer samples, most often in squamous cancer. They demonstrated that GPR87 contributes to the proliferation and survival of tumor cells (291).
Angiogenic factors in cancer
Angiogenesis is a complex process that plays an essential role in tumor growth and metastasis (292,293). In general, the diffusion limit of oxygen is approximately 100 µm, which requires that all mammalian cells be located within 100-200 µm of blood vessels (294). Without a blood supply, tumors must depend solely on diffusion for oxygen and nutrients, which results in their maintaining a size of less than 1 mm in diameter in order for survive (64). Thus, the cancer cells in microscopic solitary tumors without angiogenesis generally remain dormant and occult for long periods of time. Only angiogenic macroscopic primary tumors and metastases are clinically detectable (64).
Factors responsible for angiogenesis include VEGF, interleukin-8 (IL-8), PDGF, basic fibroblast growth factor (bFGF), the angiopoietin/Tie2 receptor complex, angiogenin, insulin-like growth factor (IGF), IGF-binding protein 1 (IGFBP-1), CXC chemokine receptor 4 (CXCR4) and its ligand CXCL12 (129,295,296). IL-8 and VEGF are the two most potent tumor-derived angiogenic factors (297-300).
VEGF as an angiogenic factor
VEGF expression participates in carcinogenesis (210,278,290,301-305). For human cancer, VEGF is the most important pro-angiogenic factor (293). In a study which examined the immnoexpression of VEGF in CIN, it was found that a progressive increase in VEGF immunoexpression was present as the tumor grade intensified (306). VEGF was distinguished by its being induced by either hypoxia or glucose deficiency (307,308). In solid tumors, some cancer cells expand outside the area of oxygen diffusion during tumor growth and become hypoxic, resulting in upregulation of VEGF and thus the occurrence of angiogenesis (309). After exposure to hypoxia for a few hours, different cell cultures showed dramatically increased VEGF messenger RNA levels which returned to background levels when a normal oxygen supply was resumed. Moreover, the production of VEGF was specifically induced by immediate proximity to necrotic tumor foci (307). Hypoxia inducible factor-1 (HIF-1) is considered a crucial mediator for the cellular response to hypoxia (309).
IL-8 as an angiogenic factor
HIF-independent mechanisms for angiogenesis have been described which involve a number of other molecules and transcription factors (309). IL-8 can be activated to preserve the tumor angiogenic response when VEGF production is suppressed. In HIF-1α knockdown DLD-1 colon cancer cells, induction of IL-8 was also found to preserve the angiogenic response. Moreover, an antibody that neutralized IL-8 substantially inhibited angiogenesis and tumor growth in HIF-knock down DLD-1 but not in wild type DLD-1 xenografts (310). These studies suggest a compensatory angiogenic role for IL-8 when VEGF production is suppressed.
IL-8, in addition to being a tumor-derived pro-angiogenic factor, is also a proinflammatory chemokine which can be induced by thrombin, HPV infection, pseudomonas or chlamydia trachomatis, gastric inflammation, or by chemical substances like palmitic acid, IL-1β, eicosapentaenoic acid, or tumor necrosis factor-α (TNFα) (311-323). Turpin et al. reported that thrombin may drive tumorigenesis in colitis-associated colon cancer (324). Zhong et al. reported that thrombin promoted IL-8 secretion, epithelial ovarian cancer cell invasion and induction of epithelial-mesenchymal transition (325). The leading cause of cervical cancer is latent infection by oncogenic HPV. In culture cell lines positive for HPV-16 oncoproteins, the expression of angiogenic modulators, which includes pro-angiogenic molecules such as bFGF, IL-8, transforming growth factor, TNFα, and VEGF, was higher in these cells when compared to control keratinocytes (326). Also, overexpression of HPV-16 E6 and E7 oncoproteins in non-small cell lung cancer cells from never-smokers significantly promoted angiogenesis and an enhanced expression of HIF-1α, VEGF, and IL-8 (327). These studies support the hypothesis that IL-8’s pro-inflammatory effect may also contribute to angiogenesis.
LPA and angiogenic factors
LPA may contribute to angiogenic homeostasis by producing angiogenic factors (328). The most important LPA down-stream angiogenic factors are VEGF and IL-8. The other LPA-downstream factor is epidermal growth factor receptor (EGFR), whose pathway may also be linked with VEGF-directed angiogenesis (329). LPA may transactivate EGFR expression in ovarian, colon or prostate cancers (212,330,331). In cervical malignancy, EGFR was expressed abnormally when compared with normal squamous epithelium (332). However, for a number of epidermal cancers upregulation of EGFR was primarily associated with tumor cell growth due to uncontrollable division of cancer cells, not angiogenesis (333-340).
LPA and VEGF
LPA may induce VEGF expression in cancer cells (210,218,273,288,301-305,341-348). This finding is usually based on studies concerned primarily with ovarian cancer cells (210,301-304,342,343,345), and less frequently with studies of prostate cancer cells (278,305), colon cancer cells (344) or lung cancer cells (346). In ovarian cancer cells, besides hypoxia LPA may induce VEGF expression and stimulate ovarian tumor growth, migration, and invasion through transcriptional activation (210,273,286,290,301-304,343,345). In fact, treating ovarian cancer cells with LPA resulted in VEGF protein levels that were elevated 1.5-fold in SKOV-3 cells, 1.9-fold in CAOV-3 cells, and threefold in OVCAR-3 cells (302). All the Edg-family LPA receptors—LPA1, LPA2, and LPA3—were detected in ovarian cancer cells; however, only LPA2 and LPA3 were found to play a role in LPA’s stimulation of ovarian tumor growth (273,302). In contrast to the observation that most ovarian cancer cells express elevated levels of LPA2 and LPA3 mRNA and variable levels of LPA1 mRNA, normal ovarian epithelial cells were found to express LPA1 mRNA but to have low levels of LPA2 and LPA3 mRNA (349). These findings—that the expression of LPA2 or LPA3 during ovarian carcinogenesis contributed to ovarian cancer aggressiveness—suggest that the targeting of LPA production and action may possess potential for the treatment of ovarian cancer (273).
LPA and IL8
In ovarian cancer cells, besides VEGF LPA may also induce IL-8 secretion (286,348,350,351). Knockdown of either LPA2 or LPA3 receptors inhibited the production of IL-6, IL-8, and VEGF in SKOV-3 and OVCAR-3 cells (273). IL-8 has also been identified as an LPA-regulated factor in ovarian cancer cells and, through its up-regulation, as a contributor to cancer development and progression (286,350).
LPA may also induce secretion of both VEGF and IL-8 proteins in colon cancer cells. However, LPA was found to induce the secretion of more IL-8 than VEGF (352). In colon cancer DLD1 cells, Shida et al. found that LPA induced a dose-dependent increase in the secretion of both IL-8 (19-fold increase at 20 µM) and VEGF (1.4-fold increase at 20 µM). LPA, at concentrations that are present physiologically, enhanced DLD1 cell migration, proliferation, and adhesion, along with enhancing the secretion of angiogenic factors, all of which are crucial for cancer metastasis (352).
In cervical cancer, it was reported that LPA induced IL-8 secretion, angiogenesis, and tumor growth. VEGF levels induced by LPA receptor expression were quite low. LPA/IL-8 signaling was mediated through activation of LPA2 and LPA3 through the Gi/nuclear factor-κB (NF-κB) pathway (72).
For other angiogenic factors, that LPA induces their expression in human cancer cells has been reported rarely. For example, in one study LPA did not significantly alter matrix metallopeptidase 2 secretion or activation in DLD1 cells (352). On the other hand, most ovarian cancer cell lines, including OVCAR-3, Caov-3, and SKOV-3, do not seem to express functional levels of PDGF receptors (353). PDGF and bFGF do not seem connected with angiogenesis due to LPA receptor over-expression (72).
Targeted therapy of angiogenic factors
The clinical use of angiogenesis inhibitors may be a promising direction in pharmacological research (43,293). For advanced and recurrent cervical cancer, targeted agent therapy, alone or combined with chemotherapy, has been under evaluation and shows promise. Several LPA receptor analogues and small molecules which target LPA receptors have been discovered which were found to be efficacious in attenuating tumor pathology (354,355). Agents targeting VEGF and EGFR signaling pathways are the most investigated molecular targeting agents for cervical cancer (355). However, EGFR inhibitors have not shown promise (41).
VEGF as an anti-angiogenic target
Currently, VEGF and its receptors are the only targets used in anti-angiogenesis therapy (356). For advanced cervical cancer, in 2014 a prospective randomized Phase III clinical trial (GOG 240) which explored the impact of adding the antiangiogenic agent bevacizumab to a combination of cisplatin and either paclitaxel or topotecan showed a small but significant therapeutic benefit of 3.7 months added to median OS without significant deterioration in health-related quality of life (357,358). Bevacizumab, a monoclonal antibody for VEGF, was associated with an increased incidence of hypertension, thromboembolic events, and gastrointestinal fistula when compared with chemotherapy alone. On August 14, 2014, the US Food and Drug Administration approved bevacizumab for the treatment of persistent, recurrent, and metastatic cervical cancer when used in combination with other chemotherapeutic agents (cisplatin-paclitaxel-bevacizumab or topotecan-paclitaxel-bevacizumab regimens) (357,359).
However, in general the clinical effects of antiangiogenic agents may easily cause drug resistance, and thus their usefulness is usually transient (360). For most tumors, anti-angiogenesis treatments targeting VEGF had only a limited OS benefit compared with conventional chemotherapy alone; moreover, these treatments tended to induce resistance and tumor cell invasion by selecting for highly invasive tumor cells or hypoxia-resistant cells (361). Specifically, intrinsic resistance has also been associated with bevacizumab (362,363). Although the GOG 240 clinical trial report on bevacizumab has encouraged its use for advanced cervical cancer treatment, in view of its high cost, limited OS benefit, and rapid induction of resistance, further exploration and evaluation of anti-angiogenic agents must be taken into consideration (41,363-367). Moreover, in a study to determine whether markers of tumor angiogenesis were associated with cervical cancer survival, high CD31 MVD but not VEGF was found to be an independent prognostic factor for progression-free survival (PFS) and OS (62).
The other VEGF-directed multi-targeted anti-angiogenic tyrosine kinase inhibitors such as cediranib and pazopanib have shown some therapeutic benefits in ovarian cancer, with both resulting in improvements in PFS and OS (39). However, prospects for their use as treatments for cervical cancer remain uncertain (366). The usefulness of novel anti-angiogenic agents thus merits further investigation.
IL-8 as an anti-angiogenic target
LPA may promote angiogenesis in both normal and cancer cells via up-regulation of IL-8. Table 2 lists reports that simultaneously investigate both angiogenesis and LPA-induced IL-8 up-regulation.
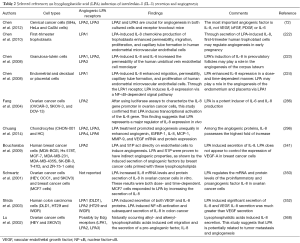
Full table
LPA treatment increased the angiogenic capability of cultured chondrocytes and resulted in enhanced capillary tube formation, monolayer permeability, migration, and cell growth in human umbilical vein endothelial cells (HUVECs). Angiogenin, IGFBP-1, IL-8, monocyte chemoattractant protein 1 (MCP-1), matrix metallopeptidase 9, and VEGF mRNA protein expression were significantly enhanced in LPA-treated chondrocytes. LPA2, 3, 4 and 6 were expressed in chondrocytes (296).
In cervical cancer, all three Edg receptors are expressed in cervical cancer cells. A study of the LPA receptor signaling cascade that used a mice model showed for the first time that knocking out the receptors LPA2 and LPA3, but not LPA1, decreased cervical cancer tumor growth and tumor MVD (72). Priming cervical cancer cells with LPA increased angiogenesis, as was shown by endothelial growth via HUVEC assay, and by endothelial migration via permeability assays. In cell cultures, knocking out the receptors LPA2 and LPA3 also inhibited angiogenesis, whereas cell proliferation was not influenced by knocking down any two or all the three Edg-family receptors. This study revealed that LPA may promote angiogenesis, and that angiogenic suppression that blocks the receptors LPA2 and LPA3 may inhibit tumor growth in vivo (72). Overall, in cervical cancer cells the most important LPA-related angiogenic factor was IL-8.
In first-trimester placental trophoblasts, the primary LPA receptor is LPA1. LPA enhanced growth-regulated oncogene—α, IL-8 and MCP-1 expression in a time- and dose-dependent manner (222).
In breast cancer cell lines (Hs 578T, MCF-7, MDA-MB-231, MDA-MB-435S, SK-BR-3, T-47D, and ZR-75-1 cells), sphingosine-1-phosphate (S1P), but not LPA, controlled the expression of VEGF. LPA and S1P had indirect angiogenic properties, as was shown by induced secretion of angiogenic factors by breast cancer cells primed with these LPLs (341).
LPA mediates IL-8 expression in human endometrial stromal cells through its LPA receptors. In endometrial specimens obtained from 38 premenopausal women undergoing hysterectomy for leiomyoma, and in decidua or placenta specimens that were obtained from 12 women who had received an elective termination of pregnancy, LPA enhanced IL-8 expression in a dose- and time-dependent manner and enhanced capillary tube formation and proliferation of human endometrial microvascular endothelial cells (224). Through the LPA1 receptor, LPA induced IL-8 expression and thus may have played a role in the angiogenesis of the endometrium and placenta (224).
In ovarian cancer cells, Fang et al. found that LPA induced expression of IL-8 mRNA mainly through transcriptional activation. Using IL-8 gene promoter luciferase assays in which cell lines were transfected with the plasmid pIL-8-Luc, they confirmed that LPA activates the IL-8 gene promoter in ovarian cancer cells. By contrast, in the control there was only a limited increase in luciferase activity in OVCAR-3 cells transfected with pGL2-Luc. The LPA2 receptor was identified as the one that most efficiently linked LPA to IL-6 and IL-8 production (286).
In in vitro ovarian cancer cells (HEY, OCC1, and SKOV3), Schwartz et al. found that LPA at concentrations of 5-15 µM induced increases in mRNA levels (2- to 7-fold increase) and in protein secretion (2- to 12-fold increase) of IL-8. In a breast cancer cell line, MCF7 cells responded to LPA by increasing the secretion of IL-8. LPA was found to regulate the mRNA and protein levels of the proinflammatory and proangiogenic factor IL-8 in ovarian cancer cells (350).
In cultured granulosa-lutein cells, LPA-induced IL-8 mediated tube formation in HUVEC assay, which suggests that in preovulatory follicles LPA may play a role in the angiogenesis of corpus luteum (223).
Additionally, in ovarian cancer cells LPA induced cell migration and the secretion of IL-8, a pro-angiogenic factor (368). This study suggests that LPA is potentially related to both tumor metastasis and angiogenesis. Also, it found that Al-LPAs induced diverse signaling pathways in ovarian cancer cells.
Overall, LPA may induce angiogenesis mediated by IL-8 in normal or cancer cells. In cervical cancer, IL-8 is the most important, if not the exclusive, mediator. Targeting the LPA/IL-8 axis may thus be a promising pharmacological approach for cervical cancer treatment.
Conclusions
Because of recent advances in cervical cancer prevention by HPV vaccination, better screening and earlier treatment for CIN, and more aggressive management of invasive cancer, cervical cancer has become more preventable and treatable. However, its incidence and mortality remain high. For metastatic or recurrent disease, targeted molecular translation may be either an alternative to cytotoxic chemotherapy or an additional treatment. Angiogenesis has a clinical, pathological and molecular meaning for cervical carcinoma. Bevacizumab, a recombinant humanized monoclonal antibody which binds with the angiogenic factor VEGF, has been demonstrated to have a significant clinical effect, with 3.7 months of OS improvement for cervical cancer patients. The ATX-LPA-IL-8 axis signaling cascade may also have potential for the anti-angiogenic treatment of cervical cancer (Figure 1). Molecular therapy that targets this pathway may improve the prognosis of patients with advanced cervical cancer, and thus merits further investigation.
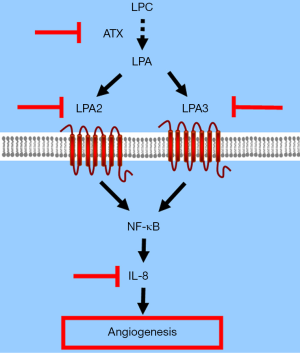
Acknowledgments
Funding: This work was supported by the National Science Council, Grant number 102-2314-B-002-110-MY2, and by National Taiwan University Hospital, Grant numbers ME-109 and MG-164.
Footnote
Provenance and Peer Review: This article was commissioned by the editorial office, Translational Cancer Research for the series “Lysophospholipids on Immunity and Cancer”. The article has undergone external peer review.
Conflicts of Interest: All authors have completed the ICMJE uniform disclosure form (available at http://dx.doi.org/10.3978/j.issn.2218-676X.2015.10.09). The series “Lysophospholipids on Immunity and Cancer” was commissioned by the editorial office without any funding or sponsorship. HL served as the unpaid Guest Editor of the series. The authors have no other conflicts of interest to declare.
Ethical Statement: The authors are accountable for all aspects of the work in ensuring that questions related to the accuracy or integrity of any part of the work are appropriately investigated and resolved.
Open Access Statement: This is an Open Access article distributed in accordance with the Creative Commons Attribution-NonCommercial-NoDerivs 4.0 International License (CC BY-NC-ND 4.0), which permits the non-commercial replication and distribution of the article with the strict proviso that no changes or edits are made and the original work is properly cited (including links to both the formal publication through the relevant DOI and the license). See: https://creativecommons.org/licenses/by-nc-nd/4.0/.
References
- Shirley MH, Barnes I, Sayeed S, et al. Incidence of breast and gynaecological cancers by ethnic group in England, 2001-2007: a descriptive study. BMC Cancer 2014;14:979. [PubMed]
- Jung KW, Won YJ, Kong HJ, et al. Cancer statistics in Korea: incidence, mortality, survival, and prevalence in 2012. Cancer Res Treat 2015;47:127-41. [PubMed]
- Bassal R, Schejter E, Bachar R, et al. Recent trends of cervical cancer and Cervical Intraepithelial Neoplasia 3 (CIN3) in Israel. Arch Gynecol Obstet 2015;292:405-13. [PubMed]
- Katanoda K, Hori M, Matsuda T, et al. An updated report on the trends in cancer incidence and mortality in Japan, 1958-2013. Jpn J Clin Oncol 2015;45:390-401. [PubMed]
- Henley SJ, Singh S, King J, et al. Invasive cancer incidence - United States, 2010. MMWR Morb Mortal Wkly Rep 2014;63:253-9. [PubMed]
- Chen W, Zheng R, Zhang S, et al. Annual report on status of cancer in China, 2010. Chin J Cancer Res 2014;26:48-58. [PubMed]
- Kachuri L, De P, Ellison LF, et al. Cancer incidence, mortality and survival trends in Canada, 1970-2007. Chronic Dis Inj Can 2013;33:69-80. [PubMed]
- Benard VB, Thomas CC, King J, et al. Vital signs: cervical cancer incidence, mortality, and screening - United States, 2007-2012. MMWR Morb Mortal Wkly Rep 2014;63:1004-9. [PubMed]
- Vaccarella S, Franceschi S, Engholm G, et al. 50 years of screening in the Nordic countries: Quantifying the effects on cervical cancer incidence. Br J Cancer 2014;111:965-9. [PubMed]
- Watson M, Benard V, Thomas C, et al. Cervical cancer incidence and mortality among American Indian and Alaska native women, 1999-2009. Am J Public Health 2014;104:S415-22. [PubMed]
- Zhan FB, Lin Y. Racial/ethnic, socioeconomic, and geographic disparities of cervical cancer advanced-stage diagnosis in Texas. Womens Health Issues 2014;24:519-27. [PubMed]
- Siegel R, Naishadham D, Jemal A. Cancer statistics for Hispanics/Latinos, 2012. CA Cancer J Clin 2012;62:283-98. [PubMed]
- Lin Y, Schootman M, Zhan FB. Racial/ethnic, area socioeconomic, and geographic disparities of cervical cancer survival in Texas. Appl Geogr 2015;56:21-8.
- Aye PS, Elwood M, Stevanovic V. Comparison of cancer survival in New Zealand and Australia, 2006-2010. N Z Med J 2014;127:14-26. [PubMed]
- Bouchbika Z, Haddad H, Benchakroun N, et al. Cancer incidence in Morocco: report from Casablanca registry 2005-2007. Pan Afr Med J 2013;16:31. [PubMed]
- Katanoda K, Matsuda T, Matsuda A, et al. An updated report of the trends in cancer incidence and mortality in Japan. Jpn J Clin Oncol 2013;43:492-507. [PubMed]
- Vaccarella S, Lortet-Tieulent J, Plummer M, et al. Worldwide trends in cervical cancer incidence: Impact of screening against changes in disease risk factors. Eur J Cancer 2013;49:3262-73. [PubMed]
- Rauh-Hain JA, Clemmer JT, Bradford LS, et al. Racial disparities in cervical cancer survival over time. Cancer 2013;119:3644-52. [PubMed]
- Gomez SL, Noone AM, Lichtensztajn DY, et al. Cancer incidence trends among Asian American populations in the United States, 1990-2008. J Natl Cancer Inst 2013;105:1096-110. [PubMed]
- Grover S, Showalter S, Kraft KH, et al. The impact of race and partner status on patterns of care and survival in young women with early-stage cervical cancer. Int J Gynecol Cancer 2013;23:494-9. [PubMed]
- Torre LA, Bray F, Siegel RL, et al. Global cancer statistics, 2012. CA Cancer J Clin 2015;65:87-108. [PubMed]
- Salicrú SR, de la Torre JF, Gil-Moreno A. The surgical management of early-stage cervical cancer. Curr Opin Obstet Gynecol 2013;25:312-9. [PubMed]
- Roque DR, Wysham WZ, Soper JT. The surgical management of cervical cancer: an overview and literature review. Obstet Gynecol Surv 2014;69:426-41. [PubMed]
- Salicrú S, Gil-Moreno A, Montero A, et al. Laparoscopic Radical Hysterectomy with Pelvic Lymphadenectomy in Early Invasive Cervical Cancer. J Minim Invasive Gynecol 2011;18:555-68. [PubMed]
- Chen RJ, Lin YH, Chen CA, et al. Influence of histologic type and age on survival rates for invasive cervical carcinoma in Taiwan. Gynecol Oncol 1999;73:184-90. [PubMed]
- Chen RJ, Chang DY, Yen ML, et al. Prognostic factors of primary adenocarcinoma of the uterine cervix. Gynecol Oncol 1998;69:157-64. [PubMed]
- Hanna TP, Shafiq J, Delaney GP, et al. The population benefit of radiotherapy for cervical cancer: Local control and survival estimates for optimally utilized radiotherapy and chemoradiation. Radiother Oncol 2015;114:389-94. [PubMed]
- Krebs L, Maillard S, Gaillot-Petit N, et al. Total radiation dose and overall treatment time are predictive for tumor sterilization in cervical carcinoma treated with chemoradiation and pulsed-dose-rate brachytherapy. Brachytherapy 2015;14:16-22. [PubMed]
- Legge F, Chiantera V, MacChia G, et al. Clinical outcome of recurrent locally advanced cervical cancer (LACC) submitted to primary multimodality therapies. Gynecol Oncol 2015;138:83-8. [PubMed]
- Mazeron R, Castelnau-Marchand P, Dumas I, et al. Impact of treatment time and dose escalation on local control in locally advanced cervical cancer treated by chemoradiation and image-guided pulsed-dose rate adaptive brachytherapy. Radiother Oncol 2015;114:257-63. [PubMed]
- Sonoda K, Yahata H, Ichinoe A, et al. Retrospective analysis of concurrent chemoradiation with triweekly cisplatin plus 5-fluorouracil versus weekly cisplatin in cervical cancer. Anticancer Res 2015;35:3447-54. [PubMed]
- Chen JL, Huang CY, Huang YS, et al. Differential clinical characteristics, treatment response and prognosis of locally advanced adenocarcinoma/adenosquamous carcinoma and squamous cell carcinoma of cervix treated with definitive radiotherapy. Acta Obstet Gynecol Scand 2014;93:661-8. [PubMed]
- Chao A, Lin CT, Lai CH. Updates in systemic treatment for metastatic cervical cancer. Curr Treat Options Oncol 2014;15:1-13. [PubMed]
- Eskander RN, Tewari KS. Chemotherapy in the treatment of metastatic, persistent, and recurrent cervical cancer. Curr Opin Obstet Gynecol 2014;26:314-21. [PubMed]
- Mountzios G, Soultati A, Pectasides D, et al. Developments in the systemic treatment of metastatic cervical cancer. Cancer Treat Rev 2013;39:430-43. [PubMed]
- Moore DH. Chemotherapy for advanced, recurrent, and metastatic cervical cancer. J Natl Compr Canc Netw 2008;6:53-7. [PubMed]
- Monk BJ, Sill MW, McMeekin DS, et al. Phase III trial of four cisplatin-containing doublet combinations in stage IVB, recurrent, or persistent cervical carcinoma: a Gynecologic Oncology Group study. J Clin Oncol 2009;27:4649-55. [PubMed]
- Tokunaga H, Nakanishi T, Iwata T, et al. Effects of chemotherapy on patients with recurrent cervical cancer previously treated with concurrent chemoradiotherapy: a retrospective multicenter survey in Japan. Int J Clin Oncol 2015;20:561-5. [PubMed]
- Schmid BC, Oehler MK. Improvements in progression-free and overall survival due to the use of anti-angiogenic agents in gynecologic cancers. Curr Treat Options Oncol 2015;16:318. [PubMed]
- Leitao MM Jr. The highs and lows of targeted therapies. Oncology 2015;29:330. (Willison Park). [PubMed]
- Elit LM, Hirte H. Management of advanced or recurrent cervical cancer: chemotherapy and beyond. Expert Rev Anticancer Ther 2014;14:319-32. [PubMed]
- Folkman J. Fundamental concepts of the angiogenic process. Curr Mol Med 2003;3:643-51. [PubMed]
- Folkman J. What is the evidence that tumors are angiogenesis dependent? J Natl Cancer Inst 1990;82:4-6. [PubMed]
- Misra JS, Srivastava AN, Das V. Single life time cytological screening in high risk women as an economical and feasible approach to control cervical cancer in developing countries like India. Asian Pac J Cancer Prev 2015;16:859-62. [PubMed]
- Rai B, Dhanireddy B, Patel FD, et al. Vaginal dose, toxicity and sexual outcomes in patients of cervical cancer undergoing image based brachytherapy. Asian Pac J Cancer Prev 2014;15:3619-23. [PubMed]
- Lloyd PA, Briggs EV, Kane N, et al. Women’s experiences after a radical vaginal trachelectomy for early stage cervical cancer. A descriptive phenomenological study. Eur J Oncol Nurs 2014;18:362-71. [PubMed]
- Gyenwali D, Pariyar J, Onta SR. Factors associated with late diagnosis of cervical cancer in Nepal. Asian Pac J Cancer Prev 2013;14:4373-7. [PubMed]
- Saslow D, Solomon D, Lawson HW, et al. American Cancer Society, American Society for Colposcopy and Cervical Pathology, and American Society for Clinical Pathology screening guidelines for the prevention and early detection of cervical cancer. J Low Genit Tract Dis 2012;16:175-204. [PubMed]
- Hellberg D, Nilsson S. 20-Year experience of follow-up of the abnormal smear with colposcopy and histology and treatment by conization or cryosurgery. Gynecol Oncol 1990;38:166-9. [PubMed]
- O’Connor DM. A tissue basis for colposcopic findings. Obstet Gynecol Clin North Am 2008;35:565-82. [PubMed]
- Tjalma W, Sonnemans H, Weyler J, et al. Angiogenesis in cervical intraepithelial neoplasia and the risk of recurrence. Am J Obstet Gynecol 1999;181:554-9. [PubMed]
- Chen RJ, Chang DY, Yen ML, et al. Independent clinical factors which correlate with failures in diagnosing early cervical cancer. Gynecol Oncol 1995;58:356-61. [PubMed]
- Alcázar JL, Jurado M, Lopez-García G. Tumor vascularization in cervical cancer by 3-dimensional power Doppler angiography correlation with tumor characteristics. Int J Gynecol Cancer 2010;20:393-7. [PubMed]
- Suren A, Osmers R, Kuhn W. 3D Color Power Angio imaging: a new method to assess intracervical vascularization in benign and pathological conditions. Ultrasound Obstet Gynecol 1998;11:133-7. [PubMed]
- Belitsos P, Papoutsis D, Rodolakis A, et al. Three-dimensional power Doppler ultrasound for the study of cervical cancer and precancerous lesions. Ultrasound Obstet Gynecol 2012;40:576-81. [PubMed]
- Hammes LS, Tekmal RR, Naud P, et al. Up-regulation of VEGF, c-fms and COX-2 expression correlates with severity of cervical cancer precursor (CIN) lesions and invasive disease. Gynecol Oncol 2008;110:445-51. [PubMed]
- Landt S, Wehling M, Heidecke H, et al. Prognostic significance of angiogenic factors in uterine cervical cancer. Anticancer Res 2011;31:2589-95. [PubMed]
- Kim YH, Kim MA, Park IA, et al. VEGF polymorphisms in early cervical cancer susceptibility, angiogenesis, and survival. Gynecol Oncol 2010;119:232-6. [PubMed]
- Phoophitphong T, Hanprasertpong J, Dechsukhum C, et al. Correlation of angiogenesis and recurrence-free survival of early stage cervical cancer patients undergoing radical hysterectomy with pelvic lymph node dissection. J Obstet Gynaecol Res 2007;33:840-8. [PubMed]
- Dunst J, Kuhnt T, Strauss HG, et al. Anemia in cervical cancers: Impact on survival, patterns of relapse, and association with hypoxia and angiogenesis. Int J Radiat Oncol Biol Phys 2003;56:778-87. [PubMed]
- Cooper RA, Wilks DP, Logue JP, et al. High tumor angiogenesis is associated with poorer survival in carcinoma of the cervix treated with radiotherapy. Clin Cancer Res 1998;4:2795-800. [PubMed]
- Randall LM, Monk BJ, Darcy KM, et al. Markers of angiogenesis in high-risk, early-stage cervical cancer: A Gynecologic Oncology Group study. Gynecol Oncol 2009;112:583-9. [PubMed]
- Obermair A, Wanner C, Bilgi S, et al. Tumor angiogenesis in stage IB cervical cancer: correlation of microvessel density with survival. Am J Obstet Gynecol 1998;178:314-9. [PubMed]
- Naumov GN, Akslen LA, Folkman J. Role of angiogenesis in human tumor dormancy: animal models of the angiogenic switch. Cell Cycle 2006;5:1779-87. [PubMed]
- Sharma B, Singh N, Gupta N, et al. Diagnostic Modalities of Precancerous and Cancerous Cervical Lesions with Special Emphasis on CD31 Angiogenesis Factor as a Marker. Patholog Res Int 2013;2013:243168.
- Dellas A, Moch H, Schultheiss E, et al. Angiogenesis in cervical neoplasia: Microvessel quantitation in precancerous lesions and invasive carcinomas with clinicopathological correlations. Gynecol Oncol 1997;67:27-33. [PubMed]
- Biedka M, Makarewicz R, Marszałek A, et al. Labeling of microvessel density, lymphatic vessel density and potential role of proangiogenic and lymphangiogenic factors as a predictive/prognostic factors after radiotherapy in patients with cervical cancer. Eur J Gynaecol Oncol 2012;33:399-405. [PubMed]
- Huang TH, Chu TY. Repression of miR-126 and upregulation of adrenomedullin in the stromal endothelium by cancer-stromal cross talks confers angiogenesis of cervical cancer. Oncogene 2014;33:3636-47. [PubMed]
- Song EL, Hou YP, Yu SP, et al. EFEMP1 expression promotes angiogenesis and accelerates the growth of cervical cancer in vivo. Gynecol Oncol 2011;121:174-80. [PubMed]
- Wang Z, Lv J, Zhang T. Combination of IL-24 and cisplatin inhibits angiogenesis and lymphangiogenesis of cervical cancer xenografts in a nude mouse model by inhibiting VEGF, VEGF-C and PDGF-B. Oncol Rep 2015;33:2468-76. [PubMed]
- Vieira SC, Silva BB, Pinto GA, et al. CD34 as a marker for evaluating angiogenesis in cervical cancer. Pathol Res Pract 2005;201:313-8. [PubMed]
- Chen RJ, Chen SU, Chou CH, et al. Lysophosphatidic acid receptor 2/3-mediated IL-8-dependent angiogenesis in cervical cancer cells. Int J Cancer 2012;131:789-802. [PubMed]
- Mandic A, Usaj Knezevic S, Kapicl Ivkovic T. Tissue expression of VEGF in cervical intraepithelial neoplasia and cervical cancer. J BUON 2014;19:958-64. [PubMed]
- Dobbs SP, Hewett PW, Johnson IR, et al. Angiogenesis is associated with vascular endothelial growth factor expression in cervical intraepithelial neoplasia. Br J Cancer 1997;76:1410-5. [PubMed]
- Smith-McCune KK, Weidner N. Demonstration and characterization of the angiogenic properties of cervical dysplasia. Cancer Res 1994;54:800-4. [PubMed]
- Hosogaya S, Yatomi Y, Nakamura K, et al. Measurement of plasma lysophosphatidic acid concentration in healthy subjects: Strong correlation with lysophospholipase D activity. Ann Clin Biochem 2008;45:364-8. [PubMed]
- Eder AM, Sasagawa T, Mao M, et al. Constitutive and lysophosphatidic acid (LPA)-induced LPA production: Role of phospholipase D and phospholipase A2. Clin Cancer Res 2000;6:2482-91. [PubMed]
- Luquain C, Singh A, Wang L, et al. Role of phospholipase D in agonist-stimulated lysophosphatidic acid synthesis by ovarian cancer cells. J Lipid Res 2003;44:1963-75. [PubMed]
- Shen Z, Belinson J, Morton RE, et al. Phorbol 12-myristate 13-acetate stimulates lysophosphatidic acid secretion from ovarian and cervical cancer cells but not from breast or leukemia cells. Gynecol Oncol 1998;71:364-8. [PubMed]
- van der Bend RL, de Widt J, van Corven EJ, et al. The biologically active phospholipid, lysophosphatidic acid, induces phosphatidylcholine breakdown in fibroblasts via activation of phospholipase D. Comparison with the response to endothelin. Biochem J 1992;285:235-40. [PubMed]
- Gesta S, Simon MF, Rey A, et al. Secretion of a lysophospholipase D activity by adipocytes: Involvement in lysophosphatidic acid synthesis. J Lipid Res 2002;43:904-10. [PubMed]
- Rancoule C, Dusaulcy R, Tréguer K, et al. Involvement of autotaxin/lysophosphatidic acid signaling in obesity and impaired glucose homeostasis. Biochimie 2014;96:140-3. [PubMed]
- Federico L, Ren H, Mueller PA, et al. Autotaxin and its product lysophosphatidic acid suppress brown adipose differentiation and promote diet-induced obesity in mice. Mol Endocrinol 2012;26:786-97. [PubMed]
- Ren J, Xiao YJ, Singh LS, et al. Lysophosphatidic acid is constitutively produced by human peritoneal mesothelial cells and enhances adhesion, migration, and invasion of ovarian cancer cells. Cancer Res 2006;66:3006-14. [PubMed]
- Boucharaba A, Serre CM, Grès S, et al. Platelet-derived lysophosphatidic acid supports the progression of osteolytic bone metastases in breast cancer. J Clin Invest 2004;114:1714-25. [PubMed]
- Sano T, Baker D, Virag T, et al. Multiple mechanisms linked to platelet activation result in lysophosphatidic acid and sphingosine 1-phosphate generation in blood. J Biol Chem 2002;277:21197-206. [PubMed]
- Jesionowska A, Cecerska E, Dolegowska B. Methods for quantifying lysophosphatidic acid in body fluids: a review. Anal Biochem 2014;453:38-43. [PubMed]
- Shan L, Jaffe K, Li S, et al. Quantitative determination of lysophosphatidic acid by LC/ESI/MS/MS employing a reversed phase HPLC column. J Chromatogr B Analyt Technol Biomed Life Sci 2008;864:22-8. [PubMed]
- Wijesinghe DS, Mayton EK, Mietla JA, et al. Characterization of lysophosphatidic acid subspecies produced by autotaxin using a modified HPLC ESI-MS/MS method. Anal Methods 2011;3:2822-8. [PubMed]
- Zhao Z, Xu Y. Measurement of endogenous lysophosphatidic acid by ESI-MS/MS in plasma samples requires pre-separation of lysophosphatidylcholine. J Chromatogr B Analyt Technol Biomed Life Sci 2009;877:3739-42. [PubMed]
- Bernhart E, Kollroser M, Rechberger G, et al. Lysophosphatidic acid receptor activation affects the C13NJ microglia cell line proteome leading to alterations in glycolysis, motility, and cytoskeletal architecture. Proteomics 2010;10:141-58. [PubMed]
- Liu XW, Sok DE, Yook HS, et al. Inhibition of lysophospholipase D activity by unsaturated lysophosphatidic acids or seed extracts containing 1-linoleoyl and 1-oleoyl lysophosphatidic acid. J Agric Food Chem 2007;55:8717-22. [PubMed]
- Wang J, Sibrian-Vazquez M, Escobedo JO, et al. Simple enrichment and analysis of plasma lysophosphatidic acids. Analyst 2013;138:6852-9. [PubMed]
- Yoon HR, Kim H, Cho SH. Quantitative analysis of acyl-lysophosphatidic acid in plasma using negative ionization tandem mass spectrometry. J Chromatogr B Analyt Technol Biomed Life Sci 2003;788:85-92. [PubMed]
- Xu Y, Shen Z, Wiper DW, et al. Lysophosphatidic acid as a potential biomarker for ovarian and other gynecologic cancers. JAMA 1998;280:719-23. [PubMed]
- Xiao Y, Chen Y, Kennedy AW, et al. Evaluation of plasma lysophospholipids for diagnostic significance using electrospray ionization mass spectrometry (ESI-MS) analyses. Ann N Y Acad Sci 2000;905:242-59. [PubMed]
- Shen Z, Wu M, Elson P, et al. Fatty acid composition of lysophosphatidic acid and lysophosphatidylinositol in plasma from patients with ovarian cancer and other gynecological diseases. Gynecol Oncol 2001;83:25-30. [PubMed]
- Xiao YJ, Schwartz B, Washington M, et al. Electrospray ionization mass spectrometry analysis of lysophospholipids in human ascitic fluids: comparison of the lysophospholipid contents in malignant vs nonmalignant ascitic fluids. Anal Biochem 2001;290:302-13. [PubMed]
- Meleh M, Požlep B, Mlakar A, et al. Determination of serum lysophosphatidic acid as a potential biomarker for ovarian cancer. J Chromatogr B Analyt Technol Biomed Life Sci 2007;858:287-91. [PubMed]
- Kobayashi E, Ueda Y, Matsuzaki S, et al. Biomarkers for screening, diagnosis, and monitoring of ovarian cancer. Cancer Epidemiol Biomarkers Prev 2012;21:1902-12. [PubMed]
- Li YY, Zhang WC, Zhang JL, et al. Plasma levels of lysophosphatidic acid in ovarian cancer versus controls: A meta-analysis. Lipids Health Dis 2015;14:72. [PubMed]
- Baker DL, Morrison P, Miller B, et al. Plasma lysophosphatidic acid concentration and ovarian cancer. JAMA 2002;287:3081-2. [PubMed]
- Lao M, Zhu B, Pan ZM, et al. Expression of plasma lysophosphatidic acid in patients with cervical carcinoma and its clinical significance. Chin J Cancer Prev Treat 2013;20:203-5.
- Westermann AM, Havik E, Postma FR, et al. Malignant effusions contain lysophosphatidic acid (LPA)-like activity. Ann Oncol 1998;9:437-42. [PubMed]
- Aoki J. Mechanisms of lysophosphatidic acid production. Semin Cell Dev Biol 2004;15:477-89. [PubMed]
- Aoki J, Inoue A, Okudaira S. Two pathways for lysophosphatidic acid production. Biochim Biophys Acta 2008;1781:513-8.
- Aoki J, Taira A, Takanezawa Y, et al. Serum lysophosphatidic acid is produced through diverse phospholipase pathways. J Biol Chem 2002;277:48737-44. [PubMed]
- Umezu-Goto M, Kishi Y, Taira A, et al. Autotaxin has lysophospholipase D activity leading to tumor cell growth and motility by lysophosphatidic acid production. J Cell Biol 2002;158:227-33. [PubMed]
- Barbayianni E, Kaffe E, Aidinis V, et al. Autotaxin, a secreted lysophospholipase D, as a promising therapeutic target in chronic inflammation and cancer. Prog Lipid Res 2015;58:76-96. [PubMed]
- Benesch MG, Ko YM, McMullen TP, et al. Autotaxin in the crosshairs: taking aim at cancer and other inflammatory conditions. FEBS Lett 2014;588:2712-27. [PubMed]
- Benesch MG, Tang X, Maeda T, et al. Inhibition of autotaxin delays breast tumor growth and lung metastasis in mice. FASEB J 2014;28:2655-66. [PubMed]
- Nakanaga K, Hama K, Aoki J. Autotaxin-An LPA producing enzyme with diverse functions. J Biochem 2010;148:13-24. [PubMed]
- Boutin JA, Ferry G. Autotaxin. Cell Mol Life Sci 2009;66:3009-21. [PubMed]
- van Meeteren LA, Ruurs P, Stortelers C, et al. Autotaxin, a secreted lysophospholipase D, is essential for blood vessel formation during development. Mol Cell Biol 2006;26:5015-22. [PubMed]
- Kishi Y, Okudaira S, Tanaka M, et al. Autotaxin is overexpressed in glioblastoma multiforme and contributes to cell motility of glioblastoma by converting lysophosphatidylcholine to lysophosphatidic acid. J Biol Chem 2006;281:17492-500. [PubMed]
- van Meeteren LA, Brinkmann V, Saulnier-Blache JS, et al. Anticancer activity of FTY720: Phosphorylated FTY720 inhibits autotaxin, a metastasis-enhancing and angiogenic lysophospholipase D. Cancer Lett 2008;266:203-8. [PubMed]
- Murph MM, Jiang GW, Altman MK, et al. Vinyl sulfone analogs of lysophosphatidylcholine irreversibly inhibit autotaxin and prevent angiogenesis in melanoma. Bioorg Med Chem 2015;23:5999-6013. [PubMed]
- David M, Ribeiro J, Descotes F, et al. Targeting lysophosphatidic acid receptor type 1 with Debio 0719 inhibits spontaneous metastasis dissemination of breast cancer cells independently of cell proliferation and angiogenesis. Int J Oncol 2012;40:1133-41. [PubMed]
- Gaaya A, Poirier O, Mougenot N, et al. Plasticity-related gene-1 inhibits lysophosphatidic acid-induced vascular smooth muscle cell migration and proliferation and prevents neointima formation. Am J Physiol Cell Physiol 2012;303:C1104-14. [PubMed]
- Goldsmith ZG, Ha JH, Jayaraman M, et al. Lysophosphatidic Acid Stimulates the Proliferation of Ovarian Cancer Cells via the gep Proto-Oncogene Gα(12). Genes Cancer 2011;2:563-75. [PubMed]
- Hayashi M, Okabe K, Kato K, et al. Differential function of lysophosphatidic acid receptors in cell proliferation and migration of neuroblastoma cells. Cancer Lett 2012;316:91-6. [PubMed]
- Jeong GO, Shin SH, Seo EJ, et al. TAZ mediates lysophosphatidic acid-induced migration and proliferation of epithelial ovarian cancer cells. Cell Physiol Biochem 2013;32:253-63. [PubMed]
- Kawashima Y, Kushida N, Kokubun S, et al. Possible effect of lysophosphatidic acid on cell proliferation and involvement of lysophosphatidic acid and lysophosphatidic acid receptors in mechanical stretch-induced mitogen-activated protein kinase. Int J Urol 2015;22:778-84. [PubMed]
- Lee SJ, Yun CC. Colorectal cancer cells - Proliferation, survival and invasion by lysophosphatidic acid. Int J Biochem Cell Biol 2010;42:1907-10. [PubMed]
- Nobusue H, Kondo D, Yamamoto M, et al. Effects of lysophosphatidic acid on the in vitro proliferation and differentiation of a novel porcine preadipocyte cell line. Comp Biochem Physiol B Biochem Mol Biol 2010;157:401-7. [PubMed]
- Olianas MC, Dedoni S, Onali P. Antidepressants activate the lysophosphatidic acid receptor LPA(1) to induce insulin-like growth factor-I receptor transactivation, stimulation of ERK1/2 signaling and cell proliferation in CHO-K1 fibroblasts. Biochem Pharmacol 2015;95:311-23. [PubMed]
- Ramachandran S, Shida D, Nagahashi M, et al. Lysophosphatidic acid stimulates gastric cancer cell proliferation via ERK1-dependent upregulation of sphingosine kinase 1 transcription. FEBS Lett 2010;584:4077-82. [PubMed]
- Shin SH, Kwon YW, Heo SC, et al. Kröppel-like factor 4 mediates lysophosphatidic acid-stimulated migration and proliferation of PC3M prostate cancer cells. Exp Mol Med 2014;46:e104 [PubMed]
- Wang H, Liu W, Wei D, et al. Effect of the LPA-mediated CXCL12-CXCR4 axis in the tumor proliferation, migration and invasion of ovarian cancer cell lines. Oncol Lett 2014;7:1581-5. [PubMed]
- Guo R, Kasbohm EA, Arora P, et al. Expression and function of lysophosphatidic acid LPA1 receptor in prostate cancer cells. Endocrinology 2006;147:4883-92. [PubMed]
- Nakayama J, Raines TA, Lynch KR, et al. Decreased peritoneal ovarian cancer growth in mice lacking expression of lipid phosphate phosphohydrolase 1. PLoS One 2015;10:e0120071 [PubMed]
- Coon M, Ball A, Pound J, et al. Inhibition of lysophosphatidic acid acyltransferase β disrupts proliferative and survival signals in normal cells and induces apoptosis of tumor cells. Mol Cancer Ther 2003;2:1067-78. [PubMed]
- Deng W, Poppleton H, Yasuda S, et al. Optimal lysophosphatidic acid-induced DNA synthesis and cell migration but not survival require intact autophosphorylation sites of the epidermal growth factor receptor. J Biol Chem 2004;279:47871-80. [PubMed]
- Hurst-Kennedy J, Boyan BD, Schwartz Z. Lysophosphatidic acid signaling promotes proliferation, differentiation, and cell survival in rat growth plate chondrocytes. Biochim Biophys Acta 2009;1793:836-46.
- Lapierre DM, Tanabe N, Pereverzev A, et al. Lysophosphatidic acid signals through multiple receptors in osteoclasts to elevate cytosolic calcium concentration, evoke retraction, and promote cell survival. J Biol Chem 2010;285:25792-801. [PubMed]
- Li Y, Gonzalez MI, Meinkoth JL, et al. Lysophosphatidic acid promotes survival and differentiation of rat Schwann cells. J Biol Chem 2003;278:9585-91. [PubMed]
- Lin FT, Lai YJ, Makarova N, et al. The lysophosphatidic acid 2 receptor mediates down-regulation of Siva-1 to promote cell survival. J Biol Chem 2007;282:37759-69. [PubMed]
- Raj GV, Sekula JA, Guo R, et al. Lysophosphatidic acid promotes survival of androgen-insensitive prostate cancer PC3 cells via activation of NF-κB. Prostate 2004;61:105-13. [PubMed]
- Tanyi JL, Morris AJ, Wolf JK, et al. The human lipid phosphate phosphatase-3 decreases the growth, survival, and tumorigenesis of ovarian cancer cells: validation of the lysophosphatidic acid signaling cascade as a target for therapy in ovarian cancer. Cancer Res 2003;63:1073-82. [PubMed]
- Binder BY, Genetos DC, Leach JK. Lysophosphatidic acid protects human mesenchymal stromal cells from differentiation-dependent vulnerability to apoptosis. Tissue Eng Part A 2014;20:1156-64. [PubMed]
- Boruszewska D, Torres AC, Kowalczyk-Zieba I, et al. The effect of lysophosphatidic acid during in vitro maturation of bovine oocytes: embryonic development and mRNA abundances of genes involved in apoptosis and oocyte competence. Mediators Inflamm 2014;2014:670670.
- Funke M, Zhao Z, Xu Y, et al. The lysophosphatidic acid receptor LPA 1 promotes epithelial cell apoptosis after lung injury. Am J Respir Cell Mol Biol 2012;46:355-64. [PubMed]
- Hurst-Kennedy J, Zhong M, Gupta V, et al. 24R,25-Dihydroxyvitamin D3, lysophosphatidic acid, and p53: a signaling axis in the inhibition of phosphate-induced chondrocyte apoptosis. J Steroid Biochem Mol Biol 2010;122:264-71. [PubMed]
- Hwang SM, Jin MH, Shin YH, et al. Role of LPA and the Hippo pathway on apoptosis in salivary gland epithelial cells. Exp Mol Med 2014;46:e125 [PubMed]
- Li Z, Wei H, Liu X, et al. LPA rescues ER stress-associated apoptosis in hypoxia and serum deprivation-stimulated mesenchymal stem cells. J Cell Biochem 2010;111:811-20. [PubMed]
- Orosa B, González A, Mera A, et al. Lysophosphatidic acid receptor 1 suppression sensitizes rheumatoid fibroblast-like synoviocytes to tumor necrosis factor-induced apoptosis. Arthritis Rheum 2012;64:2460-70. [PubMed]
- Pan H, Cheng L, Yang H, et al. Lysophosphatidic acid rescues human dental pulp cells from ischemia-induced apoptosis. J Endod 2014;40:217-22. [PubMed]
- Sun Y, Kim NH, Ji L, et al. Lysophosphatidic acid activates β-catenin/T cell factor signaling, which contributes to the suppression of apoptosis in H19-7 cells. Mol Med Rep 2013;8:1729-33. [PubMed]
- Wang XY, Fan XS, Cai L, et al. Lysophosphatidic acid rescues bone mesenchymal stem cells from hydrogen peroxide-induced apoptosis. Apoptosis 2015;20:273-84. [PubMed]
- Zhang R, Wang J, Ma S, et al. Requirement of Osteopontin in the migration and protection against Taxol-induced apoptosis via the ATX-LPA axis in SGC7901 cells. BMC Cell Biol 2011;12:11. [PubMed]
- Hama K, Aoki J, Fukaya M, et al. Lysophosphatidic acid and autotaxin stimulate cell motility of neoplastic and non-neoplastic cells through LPA1. J Biol Chem 2004;279:17634-9. [PubMed]
- Jeong KJ, Park SY, Seo JH, et al. Lysophosphatidic acid receptor 2 and Gi/Src pathway mediate cell motility through cyclooxygenase 2 expression in CAOV-3 ovarian cancer cells. Exp Mol Med 2008;40:607-16. [PubMed]
- Khurana S, Tomar A, George SP, et al. Autotaxin and lysophosphatidic acid stimulate intestinal cell motility by redistribution of the actin modifying protein villin to the developing lamellipodia. Exp Cell Res 2008;314:530-42. [PubMed]
- Liao Y, Mu G, Zhang L, et al. Lysophosphatidic acid stimulates activation of focal adhesion kinase and paxillin and promotes cell motility, via LPA1-3, in human pancreatic cancer. Dig Dis Sci 2013;58:3524-33. [PubMed]
- Seo JH, Jeong KJ, Oh WJ, et al. Lysophosphatidic acid induces STAT3 phosphorylation and ovarian cancer cell motility: Their inhibition by curcumin. Cancer Lett 2010;288:50-6. [PubMed]
- Yamada T, Sato K, Komachi M, et al. Lysophosphatidic acid (LPA) in malignant ascites stimulates motility of human pancreatic cancer cells through LPA1. J Biol Chem 2004;279:6595-605. [PubMed]
- Yamada T, Yano S, Ogino H, et al. Lysophosphatidic acid stimulates the proliferation and motility of malignant pleural mesothelioma cells through lysophosphatidic acid receptors, LPA1 and LPA2. Cancer Sci 2008;99:1603-10. [PubMed]
- Zhang Y, Chen YC, Krummel MF, et al. Autotaxin through lysophosphatidic acid stimulates polarization, motility, and transendothelial migration of naive T cells. J Immunol 2012;189:3914-24. [PubMed]
- Alemayehu M, Dragan M, Pape C, et al. β-Arrestin2 regulates lysophosphatidic acid-induced human breast tumor cell migration and invasion via Rap1 and IQGAP1. PLoS One 2013;8:e56174 [PubMed]
- Anliker B, Choi JW, Lin ME, et al. Lysophosphatidic acid (LPA) and its receptor, LPA1, influence embryonic schwann cell migration, myelination, and cell-to-axon segregation. Glia 2013;61:2009-22. [PubMed]
- Brusevold IJ, Tveteraas IH, Aasrum M, et al. Role of LPAR3, PKC and EGFR in LPA-induced cell migration in oral squamous carcinoma cells. BMC Cancer 2014;14:432. [PubMed]
- Cai H, Xu Y. The role of LPA and YAP signaling in long-term migration of human ovarian cancer cells. Cell Commun Signal 2013;11:31. [PubMed]
- Cencetti F, Bruno G, Blescia S, et al. Lysophosphatidic acid stimulates cell migration of satellite cells. A role for the sphingosine kinase/sphingosine 1-phosphate axis. FEBS J 2014;281:4467-78. [PubMed]
- Wu DD, Zhang F, Hao F, et al. Matricellular protein Cyr61 bridges lysophosphatidic acid and integrin pathways leading to cell migration. J Biol Chem 2014;289:5774-83. [PubMed]
- Gardner JA, Ha JH, Jayaraman M, et al. The gep proto-oncogene Gα13 mediates lysophosphatidic acid-mediated migration of pancreatic cancer cells. Pancreas 2013;42:819-28. [PubMed]
- Harrison SM, Knifley T, Chen M, et al. LPA, HGF, and EGF utilize distinct combinations of signaling pathways to promote migration and invasion of MDA-MB-231 breast carcinoma cells. BMC Cancer 2013;13:501. [PubMed]
- Jans R, Mottram L, Johnson DL, et al. Lysophosphatidic acid promotes cell migration through STIM1- and Orai1-mediated Ca2+(i) mobilization and NFAT2 activation. J Invest Dermatol 2013;133:793-802. [PubMed]
- Kim EK, Ha JM, Kim YW, et al. Inhibitory role of polyunsaturated fatty acids on lysophosphatidic acid-induced cancer cell migration and adhesion. FEBS Lett 2014;588:2971-7. [PubMed]
- Kubohara Y, Komachi M, Homma Y, et al. Derivatives of Dictyostelium differentiation-inducing factors inhibit lysophosphatidic acid-stimulated migration of murine osteosarcoma LM8 cells. Biochem Biophys Res Commun 2015;463:800-5. [PubMed]
- Willier S, Butt E, Grunewald TG. Lysophosphatidic acid (LPA) signalling in cell migration and cancer invasion: a focussed review and analysis of LPA receptor gene expression on the basis of more than 1700 cancer microarrays. Biol Cell 2013;105:317-33. [PubMed]
- Yang D, Yang W, Zhang Q, et al. Migration of gastric cancer cells in response to lysophosphatidic acid is mediated by LPA receptor 2. Oncol Lett 2013;5:1048-52. [PubMed]
- Yoshikawa K, Tanabe E, Shibata A, et al. Involvement of oncogenic K-ras on cell migration stimulated by lysophosphatidic acid receptor-2 in pancreatic cancer cells. Exp Cell Res 2013;319:105-12. [PubMed]
- Badri L, Lama VN. Lysophosphatidic acid induces migration of human lung-resident mesenchymal stem cells through the β-catenin pathway. Stem Cells 2012;30:2010-9. [PubMed]
- Kim JH, Adelstein RS. LPA 1-induced migration requires nonmuscle myosin II light chain phosphorylation in breast cancer cells. J Cell Physiol 2011;226:2881-93. [PubMed]
- Kitayoshi M, Kato K, Tanabe E, et al. Enhancement of endothelial cell migration by constitutively active LPA 1-expressing tumor cells. Biochem Biophys Res Commun 2012;422:339-43. [PubMed]
- Swamydas M, Nguyen D, Allen LD, et al. Progranulin stimulated by LPA promotes the migration of aggressive breast cancer cells. Cell Commun Adhes 2011;18:119-30. [PubMed]
- Tanabe E, Kitayoshi M, Yoshikawa K, et al. Loss of lysophosphatidic acid receptor-3 suppresses cell migration activity of human sarcoma cells. J Recept Signal Transduct Res 2012;32:328-34. [PubMed]
- Sengupta S, Xiao YJ, Xu Y. A novel laminin-induced LPA autocrine loop in the migration of ovarian cancer cells. FASEB J 2003;17:1570-2. [PubMed]
- Edwards JG, Campbell G, Carr M, et al. Shapes of cells spreading on fibronectin: Measurement of the stellation of BHK21 cells induced by raising cyclic AMP, and of its reversal by serum and lysophosphatidic acid. J Cell Sci 1993;104:399-407. [PubMed]
- Khandoga AL, Pandey D, Welsch U, et al. GPR92/LPA5 lysophosphatidate receptor mediates megakaryocytic cell shape change induced by human atherosclerotic plaques. Cardiovasc Res 2011;90:157-64. [PubMed]
- David M, Machuca-Gayet I, Kikuta J, et al. Lysophosphatidic acid receptor type 1 (LPA1) plays a functional role in osteoclast differentiation and bone resorption activity. J Biol Chem 2014;289:6551-64. [PubMed]
- Frisca F, Crombie DE, Dottori M, et al. Rho/ROCK pathway is essential to the expansion, differentiation, and morphological rearrangements of human neural stem/progenitor cells induced by lysophosphatidic acid. J Lipid Res 2013;54:1192-206. [PubMed]
- Liu YB, Kharode Y, Bodine PV, et al. LPA induces osteoblast differentiation through interplay of two receptors: LPA1 and LPA4. J Cell Biochem 2010;109:794-800. [PubMed]
- Lv GM, Li P, Wang WD, et al. Lysophosphatidic acid (LPA) and endothelial differentiation gene (Edg) receptors in human pancreatic cancer. J Surg Oncol 2011;104:685-91. [PubMed]
- Spohr TC, Choi JW, Gardell SE, et al. Lysophosphatidic acid receptor-dependent secondary effects via astrocytes promote neuronal differentiation. J Biol Chem 2008;283:7470-9. [PubMed]
- Tang N, Zhao Y, Feng R, et al. Lysophosphatidic acid accelerates lung fibrosis by inducing differentiation of mesenchymal stem cells into myofibroblasts. J Cell Mol Med 2014;18:156-69. [PubMed]
- Valentine WJ, Fells JI, Perygin DH, et al. Subtype-specific residues involved in ligand activation of the endothelial differentiation gene family lysophosphatidic acid receptors. J Biol Chem 2008;283:12175-87. [PubMed]
- Boruszewska D, Sinderewicz E, Kowalczyk-Zieba I, et al. The effect of lysophosphatidic acid during in vitro maturation of bovine cumulus-oocyte complexes: Cumulus expansion, glucose metabolism and expression of genes involved in the ovulatory cascade, oocyte and blastocyst competence. Reprod Biol Endocrinol 2015;13:44. [PubMed]
- Fujita R, Ma Y, Ueda H. Lysophosphatidic acid-induced membrane ruffling and brain-derived neurotrophic factor gene expression are mediated by ATP release in primary microglia. J Neurochem 2008;107:152-60. [PubMed]
- Garrido JM, Esteban M, Roda O, et al. Lysophosphatidic acid pretreatment prevents micromolar atorvastatin-induced endothelial cell death and ensures the beneficial effects of high-concentration statin therapy on endothelial gene expression. Ann Vasc Surg 2012;26:549-58. [PubMed]
- Kamińska K, Wasielak M, Bogacka I, et al. Quantitative expression of lysophosphatidic acid receptor 3 gene in porcine endometrium during the periimplantation period and estrous cycle. Prostaglandins Other Lipid Mediat 2008;85:26-32. [PubMed]
- Massé K, Kyuno J, Bhamra S, et al. The lysophosphatidic acid (LPA) and sphingosine-1-phosphate (S1P) receptor gene families: cloning and comparative expression analysis in Xenopus laevis. Int J Dev Biol 2010;54:1361-74. [PubMed]
- Okabe K, Hayashi M, Wakabayashi N, et al. Different expressions and DNA methylation patterns of lysophosphatidic acid receptor genes in mouse tumor cells. Pathobiology 2010;77:309-14. [PubMed]
- Rhim JH, Jang IS, Choi JS, et al. Time-dependent differential gene expression in lysophosphatidic acid-treated young and senescent human diploid fibroblasts. Mech Ageing Dev 2009;130:648-51. [PubMed]
- Stortelers C, Kerkhoven R, Moolenaar WH. Multiple actions of lysophosphatidic acid on fibroblasts revealed by transcriptional profiling. BMC Genomics 2008;9:387. [PubMed]
- Tsujino M, Fujii M, Okabe K, et al. Differential expressions and DNA methylation patterns of lysophosphatidic acid receptor genes in human colon cancer cells. Virchows Arch 2010;457:669-76. [PubMed]
- Tsujiuchi T, Shimizu K, Onishi M, et al. Involvement of aberrant DNA methylation on reduced expression of lysophosphatidic acid receptor-1 gene in rat tumor cell lines. Biochem Biophys Res Commun 2006;349:1151-5. [PubMed]
- Uchida H, Matsumoto M, Ueda H. Profiling of BoNT/C3-reversible gene expression induced by lysophosphatidic acid: ephrinB1 gene up-regulation underlying neuropathic hyperalgesia and allodynia. Neurochem Int 2009;54:215-21. [PubMed]
- Venkatraman G, Benesch MG, Tang X, et al. Lysophosphatidate signaling stabilizes Nrf2 and increases the expression of genes involved in drug resistance and oxidative stress responses: Implications for cancer treatment. FASEB J 2015;29:772-85. [PubMed]
- Waters KM, Jacobs JM, Gritsenko MA, et al. Regulation of gene expression and subcellular protein distribution in MLO-Y4 osteocytic cells by lysophosphatidic acid: Relevance to dendrite outgrowth. Bone 2011;48:1328-35. [PubMed]
- Wu J, Mukherjee A, Lebman DA, et al. Gene expression of the lysophosphatidic acid receptor 1 is a target of transforming growth factor beta. Oncogene 2013;32:3198-206. [PubMed]
- Zeng Y, Kakehi Y, Nouh MA, et al. Gene expression profiles of lysophosphatidic acid-related molecules in the prostate: relevance to prostate cancer and benign hyperplasia. Prostate 2009;69:283-92. [PubMed]
- Afink GB, Van Alewijk DC, De Roos AD, et al. Lysophosphatidic acid and bradykinin have opposite effects on phenotypic transformation of normal rat kidney cells. J Cell Biochem 1994;56:480-9. [PubMed]
- Taghavi P, Verhoeven E, Jacobs JJ, et al. In vitro genetic screen identifies a cooperative role for LPA signaling and c-Myc in cell transformation. Oncogene 2008;27:6806-16. [PubMed]
- Jonkers J, Moolenaar WH. Mammary Tumorigenesis through LPA Receptor Signaling. Cancer Cell 2009;15:457-9. [PubMed]
- Li H, Wang D, Zhang H, et al. Lysophosphatidic acid stimulates cell migration, invasion, and colony formation as well as tumorigenesis/metastasis of mouse ovarian cancer in immunocompetent mice. Mol Cancer Ther 2009;8:1692-701. [PubMed]
- Lin S, Lee SJ, Shim H, et al. The absence of LPA receptor 2 reduces the tumorigenesis by Apc Min mutation in the intestine. Am J Physiol Gastrointest Liver Physiol 2010;299:G1128-38. [PubMed]
- Liu S, Umezu-Goto M, Murph M, et al. Expression of autotaxin and lysophosphatidic acid receptors increases mammary tumorigenesis, invasion, and metastases. Cancer Cell 2009;15:539-50. [PubMed]
- Su SC, Hu X, Kenney PA, et al. Autotaxin-lysophosphatidic acid signaling axis mediates tumorigenesis and development of acquired resistance to Sunitinib in renal cell carcinoma. Clin Cancer Res 2013;19:6461-72. [PubMed]
- Dutta S, Wang FQ, Wu HS, et al. The NF-κB pathway mediates lysophosphatidic acid (LPA)-induced VEGF signaling and cell invasion in epithelial ovarian cancer (EOC). Gynecol Oncol 2011;123:129-37. [PubMed]
- Harper K, Arsenault D, Boulay-Jean S, et al. Autotaxin promotes cancer invasion via the lysophosphatidic acid receptor 4: Participation of the cyclic AMP/EPAC/Rac1 signaling pathway in invadopodia formation. Cancer Res 2010;70:4634-43. [PubMed]
- Jeong KJ, Cho KH, Panupinthu N, et al. EGFR mediates LPA-induced proteolytic enzyme expression and ovarian cancer invasion: Inhibition by resveratrol. Mol Oncol 2013;7:121-9. [PubMed]
- Jeong KJ, Park SY, Cho KH, et al. The Rho/ROCK pathway for lysophosphatidic acid-induced proteolytic enzyme expression and ovarian cancer cell invasion. Oncogene 2012;31:4279-89. [PubMed]
- Komachi M, Sato K, Tobo M, et al. Orally active lysophosphatidic acid receptor antagonist attenuates pancreatic cancer invasion and metastasis in vivo. Cancer Sci 2012;103:1099-104. [PubMed]
- Lou L, Chen YX, Jin L, et al. Enhancement of invasion of hepatocellular carcinoma cells through lysophosphatidic acid receptor. J Int Med Res 2013;41:55-63. [PubMed]
- Park SY, Jeong KJ, Panupinthu N, et al. Lysophosphatidic acid augments human hepatocellular carcinoma cell invasion through LPA1 receptor and MMP-9 expression. Oncogene 2011;30:1351-9. [PubMed]
- Sun K, Duan X, Cai H, et al. Curcumin inhibits LPA-induced invasion by attenuating RhoA/ROCK/MMPs pathway in MCF7 breast cancer cells. Clin Exp Med 2015; [Epub ahead of print]. [PubMed]
- Wang FQ, Barfield E, Dutta S, et al. VEGFR-2 silencing by small interference RNA (siRNA) suppresses LPA-induced epithelial ovarian cancer (EOC) invasion. Gynecol Oncol 2009;115:414-23. [PubMed]
- Zhu B, Shi S, Ma YG, et al. Lysophosphatidic acid enhances human hepatocellular carcinoma cell migration, invasion and adhesion through P38 MAPK pathway. Hepatogastroenterology 2012;59:785-9. [PubMed]
- Lee SC, Fujiwara Y, Liu J, et al. Autotaxin and LPA1 and LPA5 receptors exert disparate functions in tumor cells versus the host tissue microenvironment in melanoma invasion and metastasis. Mol Cancer Res 2015;13:174-85. [PubMed]
- Binder BY, Sondergaard CS, Nolta JA, et al. Lysophosphatidic acid enhances stromal cell-directed angiogenesis. PLoS One 2013;8:e82134 [PubMed]
- Chen SU, Chou CH, Chao KH, et al. Lysophosphatidic acid up-regulates expression of growth-regulated oncogene-alpha, interleukin-8, and monocyte chemoattractant protein-1 in human first-trimester trophoblasts: possible roles in angiogenesis and immune regulation. Endocrinology 2010;151:369-79. [PubMed]
- Chen SU, Chou CH, Lee H, et al. Lysophosphatidic acid up-regulates expression of interleukin-8 and -6 in granulosa-lutein cells through its receptors and nuclear factor-kappaB dependent pathways: implications for angiogenesis of corpus luteum and ovarian hyperstimulation syndrome. J Clin Endocrinol Metab 2008;93:935-43. [PubMed]
- Chen SU, Lee H, Chang DY, et al. Lysophosphatidic acid mediates interleukin-8 expression in human endometrial stromal cells through its receptor and nuclear factor-kappaB-dependent pathway: a possible role in angiogenesis of endometrium and placenta. Endocrinology 2008;149:5888-96. [PubMed]
- Chen Y, Ramakrishnan DP, Ren B. Regulation of angiogenesis by phospholipid lysophosphatidic acid. Front Biosci (Landmark Ed) 2013;18:852-61. [PubMed]
- English D, Kovala AT, Welch Z, et al. Induction of endothelial cell chemotaxis by sphingosine 1-phosphate and stabilization of endothelial monolayer barrier function by lysophosphatidic acid, potential mediators of hematopoietic angiogenesis. J Hematother Stem Cell Res 1999;8:627-34. [PubMed]
- Ren B, Hale J, Srikanthan S, et al. Lysophosphatidic acid suppresses endothelial cell CD36 expression and promotes angiogenesis via a PKD-1-dependent signaling pathway. Blood 2011;117:6036-45. [PubMed]
- Rivera-Lopez CM, Tucker AL, Lynch KR. Lysophosphatidic acid (LPA) and angiogenesis. Angiogenesis 2008;11:301-10. [PubMed]
- Xu X, Prestwich GD. Inhibition of tumor growth and angiogenesis by a lysophosphatidic acid antagonist in an engineered three-dimensional lung cancer xenograft model. Cancer 2010;116:1739-50. [PubMed]
- Balogh A, Shimizu Y, Lee SC, et al. The autotaxin-LPA2 GPCR axis is modulated by γ-irradiation and facilitates DNA damage repair. Cell Signal 2015;27:1751-62. [PubMed]
- Bandoh K, Aoki J, Hosono H, et al. Molecular cloning and characterization of a novel human G-protein-coupled receptor, EDG7, for lysophosphatidic acid. J Biol Chem 1999;274:27776-85. [PubMed]
- Cheng Y, Makarova N, Tsukahara R, et al. Lysophosphatidic acid-induced arterial wall remodeling: Requirement of PPARγ but not LPA1 or LPA2 GPCR. Cell Signal 2009;21:1874-84. [PubMed]
- Fincher J, Whiteneck C, Birgbauer E. G-protein-coupled receptor cell signaling pathways mediating embryonic chick retinal growth cone collapse induced by lysophosphatidic acid and sphingosine-1-phosphate. Dev Neurosci 2014;36:443-53. [PubMed]
- Iacovelli L, Capobianco L, D’Ancona GM, et al. Regulation of lysophosphatidic acid receptor-stimulated response by G-protein-coupled receptor kinase-2 and β-arrestin 1 in FRTL-5 rat thyroid cells. J Endocrinol 2002;174:103-10. [PubMed]
- Kotarsky K, Boketoft Å, Bristulf J, et al. Lysophosphatidic acid binds to and activates GPR92, a G protein-coupled receptor highly expressed in gastrointestinal lymphocytes. J Pharmacol Exp Ther 2006;318:619-28. [PubMed]
- Lee M, Choi S, Halldén G, et al. P2Y5 is a Gαi, Gα12/13 G protein-coupled receptor activated by lysophosphatidic acid that reduces intestinal cell adhesion. Am J Physiol Gastrointest Liver Physiol 2009;297:G641-54. [PubMed]
- Liliom K, Tsukahara T, Tsukahara R, et al. Farnesyl phosphates are endogenous ligands of lysophosphatidic acid receptors: inhibition of LPA GPCR and activation of PPARs. Biochim Biophys Acta 2006;1761:1506-14.
- Murakami M, Shiraishi A, Tabata K, et al. Identification of the orphan GPCR, P2Y10 receptor as the sphingosine-1-phosphate and lysophosphatidic acid receptor. Biochem Biophys Res Commun 2008;371:707-12. [PubMed]
- Murch O, Collin M, Thiemermann C. Lysophosphatidic acid reduces the organ injury caused by endotoxemia-A role for G-protein-coupled receptors and peroxisome proliferator-activated receptor-γ. Shock 2007;27:48-54. [PubMed]
- Noguchi K, Ishii S, Shimizu T. Identification of p2y9/GPR23 as a novel G protein-coupled receptor for lysophosphatidic acid, structurally distant from the Edg family. J Biol Chem 2003;278:25600-6. [PubMed]
- Omotuyi OI, Ueda H. A novel unified ab initio and template-based approach to GPCR modeling: Case of EDG-LPA receptors. Curr Bioinform 2013;8:603-10.
- Pasternack SM, Von Kügelgen I, Aboud KA, et al. G protein-coupled receptor P2Y5 and its ligand LPA are involved in maintenance of human hair growth. Nat Genet 2008;40:329-34. [PubMed]
- Tabata K, Baba K, Shiraishi A, et al. The orphan GPCR GPR87 was deorphanized and shown to be a lysophosphatidic acid receptor. Biochem Biophys Res Commun 2007;363:861-6. [PubMed]
- Lee MJ, Thangada S, Liu CH, et al. Lysophosphatidic acid stimulates the G-protein-coupled receptor EDG-1 as a low affinity agonist. J Biol Chem 1998;273:22105-12. [PubMed]
- Bandoh K, Aoki J, Taira A, et al. Lysophosphatidic acid (LPA) receptors of the EDG family are differentially activated by LPA species. Structure-activity relationship of cloned LPA receptors. FEBS Lett 2000;478:159-65. [PubMed]
- Fang X, Yu S, Tanyi JL, et al. Convergence of multiple signaling cascades at glycogen synthase kinase 3: Edg receptor-mediated phosphorylation and inactivation by lysophosphatidic acid through a protein kinase C-dependent intracellular pathway. Mol Cell Biol 2002;22:2099-110. [PubMed]
- Furui T, LaPushin R, Mao M, et al. Overexpression of Edg-2/vzg-1 induces apoptosis and anoikis in ovarian cancer cells in a lysophosphatidic acid-independent manner. Clin Cancer Res 1999;5:4308-18. [PubMed]
- Hooks SB, Santos WL, Im DS, et al. Lysophosphatidic acid-induced mitogenesis is regulated by lipid phosphate phosphatases and is Edg-receptor independent. J Biol Chem 2001;276:4611-21. [PubMed]
- Im DS, Heise CE, Harding MA, et al. Molecular cloning and characterization of a lysophosphatidic acid receptor, Edg-7, expressed in prostate. Mol Pharmacol 2000;57:753-9. [PubMed]
- Ishii I, Contos JJA, Fukushima N, et al. Functional comparisons of the lysophosphatidic acid receptors, LP(A1)/VZG-1/EDG-2, LP(A2)/EDG-4, and LP(A3)/EDG-7 in neuronal cell lines using a retrovirus expression system. Mol Pharmacol 2000;58:895-902. [PubMed]
- Kimura Y, Schmitt A, Fukushima N, et al. Two novel Xenopus homologs of mammalian LP(A1)/EDG-2 function as lysophosphatidic acid receptors in Xenopus oocytes and mammalian cells. J Biol Chem 2001;276:15208-15. [PubMed]
- Murph MM, Scaccia LA, Volpicelli LA, et al. Agonist-induced endocytosis of lysophosphatidic acid-coupled LPA1/EDG-2 receptors via a dynamin2- and Rab5-dependent pathway. J Cell Sci 2003;116:1969-80. [PubMed]
- Nakamoto T, Yasuda K, Yasuhara M, et al. Expression of the endothelial cell differentiation gene 7 (EDG-7), a lysophosphatidic acid receptor, in ovarian tumor. J Obstet Gynaecol Res 2005;31:344-51. [PubMed]
- Ohta H, Sato K, Murata N, et al. Ki16425, a subtype-selective antagonist for EDG-family lysophosphatidic acid receptors. Mol Pharmacol 2003;64:994-1005. [PubMed]
- Stankoff B, Barron S, Allard J, et al. Oligodendroglial expression of Edg-2 receptor: developmental analysis and pharmacological responses to lysophosphatidic acid. Mol Cell Neurosci 2002;20:415-28. [PubMed]
- Weiner JA, Hecht JH, Chun J. Lysophosphatidic acid receptor gene vzg-1/lpA1/edg-2 is expressed by mature oligodendrocytes during myelination in the postnatal murine brain. J Comp Neurol 1998;398:587-98. [PubMed]
- Xing Y, Ganji SH, Noh JW, et al. Cell density-dependent expression of EDG family receptors and mesangial cell proliferation: Role in lysophosphatidic acid-mediated cell growth. Am J Physiol Renal Physiol 2004;287:F1250-7. [PubMed]
- Young KW, Bootman MD, Channing DR, et al. Lysophosphatidic acid-induced Ca2+ mobilization requires intracellular sphingosine 1-phosphate production: Potential involvement of endogenous Edg-4 receptors. J Biol Chem 2000;275:38532-9. [PubMed]
- Erickson JR, Wu JJ, Goddard JG, et al. Edg-2/Vzg-1 couples to the yeast pheromone response pathway selectively in response to lysophosphatidic acid. J Biol Chem 1998;273:1506-10. [PubMed]
- Fukushima N, Kimura Y, Chun J. A single receptor encoded by vzg-1/lpA1/edg-2 couples to G proteins and mediates multiple cellular responses to lysophosphatidic acid. Proc Natl Acad Sci U S A 1998;95:6151-6. [PubMed]
- Peyruchaud O, Mosher DF. Differential stimulation of signaling pathways initiated by Edg-2 in response to lysophosphatidic acid or sphingosine-1-phosphate. Cell Mol Life Sci 2000;57:1109-16. [PubMed]
- Choi JW, Herr DR, Noguchi K, et al. LPA receptors: Subtypes and biological actions. Annu Rev Pharmacol Toxicol 2010;50:157-86. [PubMed]
- Haserück N, Erl W, Pandey D, et al. The plaque lipid lysophosphatidic acid stimulates platelet activation and platelet-monocyte aggregate formation in whole blood: Involvement of P2Y 1 and P2Y12 receptors. Blood 2004;103:2585-92. [PubMed]
- Hecht JH, Weiner JA, Post SR, et al. Ventricular zone gene-1 (vzg-1) encodes a lysophosphatidic acid receptor expressed in neurogenic regions of the developing cerebral cortex. J Cell Biol 1996;135:1071-83. [PubMed]
- An S, Bleu T, Hallmark OG, et al. Characterization of a novel subtype of human G protein-coupled receptor for lysophosphatidic acid. J Biol Chem 1998;273:7906-10. [PubMed]
- Kihara Y, Maceyka M, Spiegel S, et al. Lysophospholipid receptor nomenclature review: IUPHAR Review 8. Br J Pharmacol 2014;171:3575-94. [PubMed]
- Komachi M, Tomura H, Malchinkhuu E, et al. LPA1 receptors mediate stimulation, whereas LPA2 receptors mediate inhibition, of migration of pancreatic cancer cells in response to lysophosphatidic acid and malignant ascites. Carcinogenesis 2009;30:457-65. [PubMed]
- Park SJ, Lee KP, Kang S, et al. Lysophosphatidylethanolamine utilizes LPA1 and CD97 in MDA-MB-231 breast cancer cells. Cell Signal 2013;25:2147-54. [PubMed]
- Shida D, Fang X, Kordula T, et al. Cross-talk between LPA1 and epidermal growth factor receptors mediates up-regulation of sphingosine kinase 1 to promote gastric cancer cell motility and invasion. Cancer Res 2008;68:6569-77. [PubMed]
- Shin KJ, Kim YL, Lee S, et al. Lysophosphatidic acid signaling through LPA receptor subtype 1 induces colony scattering of gastrointestinal cancer cells. J Cancer Res Clin Oncol 2009;135:45-52. [PubMed]
- Shida D, Kitayama J, Yamaguchi H, et al. Dual mode regulation of migration by lysophosphatidic acid in human gastric cancer cells. Exp Cell Res 2004;301:168-78. [PubMed]
- Hurst JH, Mendpara N, Hooks SB. Regulator of G-protein signalling expression and function in ovarian cancer cell lines. Cell Mol Biol Lett 2009;14:153-74. [PubMed]
- Yu S, Murph MM, Lu Y, et al. Lysophosphatidic acid receptors determine tumorigenicity and aggressiveness of ovarian cancer cells. J Natl Cancer Inst 2008;100:1630-42. [PubMed]
- Oyesanya RA, Lee ZP, Wu J, et al. Transcriptional and post-transcriptional mechanisms for lysophosphatidic acid-induced cyclooxygenase-2 expression in ovarian cancer cells. FASEB J 2008;22:2639-51. [PubMed]
- Hurst JH, Hooks SB. Lysophosphatidic acid stimulates cell growth by different mechanisms in SKOV-3 and Caov-3 ovarian cancer cells: Distinct roles for Gi- and rho-dependent pathways. Pharmacology 2009;83:333-47. [PubMed]
- Hao F, Tan M, Xu X, et al. Lysophosphatidic acid induces prostate cancer PC3 cell migration via activation of LPA(1), p42 and p38alpha. Biochim Biophys Acta 2007;1771:883-92.
- Hasegawa Y, Murph M, Yu S, et al. Lysophosphatidic acid (LPA)-induced vasodilator-stimulated phosphoprotein mediates lamellipodia formation to initiate motility in PC-3 prostate cancer cells. Mol Oncol 2008;2:54-69. [PubMed]
- Lin CE, Chen SU, Lin CC, et al. Lysophosphatidic acid enhances vascular endothelial growth factor-C expression in human prostate cancer PC-3 cells. PLoS One 2012;7:e41096 [PubMed]
- Lee SJ, Ritter SL, Zhang H, et al. MAGI-3 competes with NHERF-2 to negatively regulate LPA2 receptor signaling in colon cancer cells. Gastroenterology 2011;140:924-34. [PubMed]
- Yun CC, Sun H, Wang D, et al. LPA2 receptor mediates mitogenic signals in human colon cancer cells. Am J Physiol Cell Physiol 2005;289:C2-11. [PubMed]
- Rusovici R, Ghaleb A, Shim H, et al. Lysophosphatidic acid prevents apoptosis of Caco-2 colon cancer cells via activation of mitogen-activated protein kinase and phosphorylation of Bad. Biochim Biophys Acta 2007;1770:1194-203.
- Zhang H, Bialkowska A, Rusovici R, et al. Lysophosphatidic acid facilitates proliferation of colon cancer cells via induction of Krüppel-like factor 5. J Biol Chem 2007;282:15541-9. [PubMed]
- Yang M, Zhong WW, Srivastava N, et al. G protein-coupled lysophosphatidic acid receptors stimulate proliferation of colon cancer cells through the β-catenin pathway. Proc Natl Acad Sci U S A 2005;102:6027-32. [PubMed]
- Mukherjee A, Wu J, Barbour S, et al. Lysophosphatidic acid activates lipogenic pathways and de novo lipid synthesis in ovarian cancer cells. J Biol Chem 2012;287:24990-5000. [PubMed]
- Wang P, Wu XH, Shan BE, et al. Effect of antisense oligodeoxynucleotides on inhibition of LPA receptors and regulation of MMP-2 and MMP-9 in ovarian cancer cells. Chin J Cancer Prev Treat 2010;17:50-4.
- Fang X, Yu S, Bast RC, et al. Mechanisms for lysophosphatidic acid-induced cytokine production in ovarian cancer cells. J Biol Chem 2004;279:9653-61. [PubMed]
- Schulte KM, Beyer A, Khrer K, et al. Lysophosphatidic acid, a novel lipid growth factor for human thyroid cells: Over-expression of the high-affinity receptor EDG4 in differentiated thyroid cancer. Int J Cancer 2001;92:249-56. [PubMed]
- Lin CC, Lin CE, Lin YC, et al. Lysophosphatidic acid induces reactive oxygen species generation by activating protein kinase C in PC-3 human prostate cancer cells. Biochem Biophys Res Commun 2013;440:564-9. [PubMed]
- Ishii S, Hirane M, Fukushima K, et al. Diverse effects of LPA4, LPA5 and LPA6 on the activation of tumor progression in pancreatic cancer cells. Biochem Biophys Res Commun 2015;461:59-64. [PubMed]
- Ptaszynska MM, Pendrak ML, Bandle RW, et al. Positive feedback between vascular endothelial growth factor-A and autotaxin in ovarian cancer cells. Mol Cancer Res 2008;6:352-63. [PubMed]
- Glatt S, Halbauer D, Heindl S, et al. hGPR87 contributes to viability of human tumor cells. Int J Cancer 2008;122:2008-16. [PubMed]
- Krill LS, Tewari KS. Exploring the therapeutic rationale for angiogenesis blockade in cervical cancer. Clin Ther 2015;37:9-19. [PubMed]
- Tomao F, Papa A, Rossi L, et al. Angiogenesis and antiangiogenic agents in cervical cancer. Onco Targets Ther 2014;7:2237-48. [PubMed]
- Torres Filho IP, Leunig M, Yuan F, et al. Noninvasive measurement of microvascular and interstitial oxygen profiles in a human tumor in SCID mice. Proc Natl Acad Sci U S A 1994;91:2081-5. [PubMed]
- Gavalas NG, Liontos M, Trachana SP, et al. Angiogenesis-related pathways in the pathogenesis of ovarian cancer. Int J Mol Sci 2013;14:15885-909. [PubMed]
- Chuang YW, Chang WM, Chen KH, et al. Lysophosphatidic acid enhanced the angiogenic capability of human chondrocytes by regulating Gi/NF-kB-dependent angiogenic factor expression. PLoS One 2014;9:e95180 [PubMed]
- Plate KH, Breier G, Weich HA, et al. Vascular endothelial growth factor is a potential tumour angiogenesis factor in human gliomas in vivo. Nature 1992;359:845-8. [PubMed]
- Li A, Dubey S, Varney ML, et al. IL-8 directly enhanced endothelial cell survival, proliferation, and matrix metalloproteinases production and regulated angiogenesis. J Immunol 2003;170:3369-76. [PubMed]
- Li A, Varney ML, Valasek J, et al. Autocrine role of interleukin-8 in induction of endothelial cell proliferation, survival, migration and MMP-2 production and angiogenesis. Angiogenesis 2005;8:63-71. [PubMed]
- Ning Y, Manegold PC, Hong YK, et al. Interleukin-8 is associated with proliferation, migration, angiogenesis and chemosensitivity in vitro and in vivo in colon cancer cell line models. Int J Cancer 2011;128:2038-49. [PubMed]
- Bermudez Y, Yang H, Saunders BO, et al. VEGF- and LPA-induced telomerase in human ovarian cancer cells is Sp1-dependent. Gynecol Oncol 2007;106:526-37. [PubMed]
- Hu YL, Tee MK, Goetzl EJ, et al. Lysophosphatidic acid induction of vascular endothelial growth factor expression in human ovarian cancer cells. J Natl Cancer Inst 2001;93:762-8. [PubMed]
- Jeon ES, Heo SC, Lee IH, et al. Ovarian cancer-derived lysophosphatidic acid stimulates secretion of VEGF and stromal cell-derived factor-1α from human mesenchymal stem cells. Exp Mol Med 2010;42:280-93. [PubMed]
- Song Y, Wu J, Oyesanya RA, et al. Sp-1 and c-Myc mediate lysophosphatidic acid-induced expression of vascular endothelial growth factor in ovarian cancer cells via a hypoxia-inducible factor-1-independent mechanism. Clin Cancer Res 2009;15:492-501. [PubMed]
- Wu PY, Lin YC, Lan SY, et al. Aromatic hydrocarbon receptor inhibits lysophosphatidic acid-induced vascular endothelial growth factor-A expression in PC-3 prostate cancer cells. Biochem Biophys Res Commun 2013;437:440-5. [PubMed]
- Belfort-Mattos PN, Focchi GR, Speck NM, et al. Immunohistochemical expression of granzyme B and vascular endothelial growth factor (VEGF) in normal uterine cervices and low and high grade squamous intraepithelial lesions. Eur J Gynaecol Oncol 2010;31:459-61. [PubMed]
- Shweiki D, Itin A, Soffer D, et al. Vascular endothelial growth factor induced by hypoxia may mediate hypoxia-initiated angiogenesis. Nature 1992;359:843-5. [PubMed]
- Shweiki D, Neeman M, Itin A, et al. Induction of vascular endothelial growth factor expression by hypoxia and by glucose deficiency in multicell spheroids: Implications for tumor angiogenesis. Proc Natl Acad Sci U S A 1995;92:768-72. [PubMed]
- North S, Moenner M, Bikfalvi A. Recent developments in the regulation of the angiogenic switch by cellular stress factors in tumors. Cancer Lett 2005;218:1-14. [PubMed]
- Mizukami Y, Jo WS, Duerr EM, et al. Induction of interleukin-8 preserves the angiogenic response in HIF-1α-deficient colon cancer cells. Nat Med 2005;11:992-7. [PubMed]
- Bouchelouche K, Alvarez S, Horn T, et al. Human detrusor smooth muscle cells release interleukin-6, interleukin-8, and RANTES in response to proinflammatory cytokines interleukin-1β and tumor necrosis factor-α. Urology 2006;67:214-9. [PubMed]
- Bruch-Gerharz D, Fehsel K, Suschek C, et al. A proinflammatory activity of interleukin 8 in human skin: Expression of the inducible nitric oxide synthase in psoriatic lesions and cultured keratinocytes. J Exp Med 1996;184:2007-12. [PubMed]
- Buchholz KR, Stephens RS. Activation of the host cell proinflammatory interleukin-8 response by Chlamydia trachomatis. Cell Microbiol 2006;8:1768-79. [PubMed]
- Elssner A, Jaumann F, Dobmann S, et al. Elevated levels of interleukin-8 and transforming growth factor-beta in bronchoalveolar lavage fluid from patients with bronchiolitis obliterans syndrome: Proinflammatory role of bronchial epithelial cells. Transplantation 2000;70:362-7. [PubMed]
- Fabbri E, Borgatti M, Montagner G, et al. Expression of microRNA-93 and interleukin-8 during Pseudomonas aeruginosa-mediated induction of proinflammatory responses. Am J Respir Cell Mol Biol 2014;50:1144-55. [PubMed]
- Joshi-Barve S, Barve SS, Amancherla K, et al. Palmitic acid induces production of proinflammatory cytokine interleukin-8 from hepatocytes. Hepatology 2007;46:823-30. [PubMed]
- Matsushita A, Son DO, Satsu H, et al. Inhibitory effect of lactoperoxidase on the secretion of proinflammatory cytokine interleukin-8 in human intestinal epithelial Caco-2 cells. Int Dairy J 2008;18:932-8.
- Pechkovsky DV, Zissel G, Ziegenhagen MW, et al. Effect of proinflammatory cytokines on interleukin-8 mRNA expression and protein production by isolated human alveolar epithelial cells type II in primary culture. Eur Cytokine Netw 2000;11:618-25. [PubMed]
- Shahbakhti H, Watson RE, Azurdia RM, et al. Influence of eicosapentaenoic acid, an omega-3 fatty acid, on ultraviolet-B generation of prostaglandin-E2 and proinflammatory cytokines interleukin-1β, tumor necrosis factor-α, interleukin-6 and interleukin-8 in human skin in vivo. Photochem Photobiol 2004;80:231-5. [PubMed]
- Shimada T, Watanabe N, Ohtsuka Y, et al. Polaprezinc down-regulates proinflammatory cytokine-induced nuclear factor-κB activation and interleukin-8 expression in gastric epithelial cells. J Pharmacol Exp Ther 1999;291:345-52. [PubMed]
- Simmons S, Lee RV, Möller T, et al. Thrombin induces release of proinflammatory chemokines interleukin-8 and interferon-γ-induced protein-10 from cultured human fetal astrocytes. Neuroreport 2013;24:36-40. [PubMed]
- Tiemessen CT, Kilroe B, Martin DJ. Interleukin-8 fails to induce human immunodeficiency virus-1 expression in chronically infected promonocytic U1 cells but differentially modulates induction by proinflammatory cytokines. Immunology 2000;101:140-6. [PubMed]
- Wilmer JL, Luster MI. Chemical induction of interleukin-8, a proinflammatory chemokine, in human epidermal keratinocyte cultures and its relation to cytogenetic toxicity. Cell Biol Toxicol 1995;11:37-50. [PubMed]
- Turpin B, Miller W, Rosenfeldt L, et al. Thrombin drives tumorigenesis in colitis-associated colon cancer. Cancer Res 2014;74:3020-30. [PubMed]
- Zhong YC, Zhang T, Di W, et al. Thrombin promotes epithelial ovarian cancer cell invasion by inducing epithelial-mesenchymal transition. J Gynecol Oncol 2013;24:265-72. [PubMed]
- Bequet-Romero M, López-Ocejo O. Angiogenesis modulators expression in culture cell lines positives for HPV-16 oncoproteins. Biochem Biophys Res Commun 2000;277:55-61. [PubMed]
- Li G, He L, Zhang E, et al. Overexpression of human papillomavirus (HPV) type 16 oncoproteins promotes angiogenesis via enhancing HIF-1α and VEGF expression in non-small cell lung cancer cells. Cancer Lett 2011;311:160-70. [PubMed]
- Kazlauskas A. Lysophosphatidic acid contributes to angiogenic homeostasis. Exp Cell Res 2015;333:166-70. [PubMed]
- Tabernero J. The role of VEGF and EGFR inhibition: Implications for combining Anti-VEGF and Anti-EGFR Agents. Mol Cancer Res 2007;5:203-20. [PubMed]
- Bektas M, Payne SG, Liu H, et al. A novel acylglycerol kinase that produces lysophosphatidic acid modulates cross talk with EGFR in prostate cancer cells. J Cell Biol 2005;169:801-11. [PubMed]
- Shida D, Kitayama J, Yamaguchi H, et al. Lysophosphatidic acid transactivates both c-Met and epidermal growth factor receptor, and induces cylooxygenase-2 expression in human colon cancer LoVo cells. World J Gastroenterol 2005;11:5638-43. [PubMed]
- Dellas A, Schultheiss E, Almendral AC, et al. Assessment of EGFR and TGF-alpha expression in relationship to HPV status and Ki-67 distribution in cervical intraepithelial neoplasms. Int J Cancer 1996;69:165-9. [PubMed]
- Galloway TJ, Wirth LJ, Colevas AD, et al. A phase I study of CUDC-101, a multitarget inhibitor of HDACs, EGFR, and HER2, in combination with chemoradiation in patients with head and neck squamous cell carcinoma. Clin Cancer Res 2015;21:1566-73. [PubMed]
- Khan H, Gupta S, Husain N, et al. Correlation between expressions of Cyclin-D1, EGFR and p53 with chemoradiation response in patients of locally advanced oral squamous cell carcinoma. BBA Clin 2015;3:11-7.
- Niehr F, Weichert W, Stenzinger A, et al. CCI-779 (Temsirolimus) exhibits increased anti-tumor activity in low EGFR expressing HNSCC cell lines and is effective in cells with acquired resistance to cisplatin or cetuximab. J Transl Med 2015;13:106. [PubMed]
- Walker F, Abramowitz L, Benabderrahmane D, et al. Growth factor receptor expression in anal squamous lesions: modifications associated with oncogenic human papillomavirus and human immunodeficiency virus. Hum Pathol 2009;40:1517-27. [PubMed]
- Yaeger R, Cercek A, O’Reilly EM, et al. Pilot trial of combined BRAF and EGFR inhibition in BRAF-mutant metastatic colorectal cancer patients. Clin Cancer Res 2015;21:1313-20. [PubMed]
- Khan Z, Bisen PS. Oncoapoptotic signaling and deregulated target genes in cancers: special reference to oral cancer. Biochim Biophys Acta 2013;1836:123-45.
- Lui VW, Grandis JR. EGFR-mediated cell cycle regulation. Anticancer Res 2002;22:1-11. [PubMed]
- Wang QS, Sabourin CL, Bijur GN, et al. Alterations in transforming growth factor-α and epidermal growth factor receptor expression during rat esophageal tumorigenesis. Mol Carcinog 1996;15:144-53. [PubMed]
- Boucharaba A, Guillet B, Menaa F, et al. Bioactive lipids lysophosphatidic acid and sphingosine 1-phosphate mediate breast cancer cell biological functions through distinct mechanisms. Oncol Res 2009;18:173-84. [PubMed]
- Folkman J. A new link in ovarian cancer angiogenesis: Lysophosphatidic acid and vascular endothelial growth factor expression. J Natl Cancer Inst 2001;93:734-5. [PubMed]
- Fujita T, Miyamoto S, Onoyama I, et al. Expression of lysophosphatidic acid receptors and vascular endothelial growth factor mediating lysophosphatidic acid in the development of human ovarian cancer. Cancer Lett 2003;192:161-9. [PubMed]
- Fukui R, Tanabe E, Kitayoshi M, et al. Negative regulation of cell motile and invasive activities by lysophosphatidic acid receptor-3 in colon cancer HCT116 cells. Tumour Biol 2012;33:1899-905. [PubMed]
- So J, Wang FQ, Navari J, et al. LPA-induced epithelial ovarian cancer (EOC) in vitro invasion and migration are mediated by VEGF receptor-2 (VEGF-R2). Gynecol Oncol 2005;97:870-8. [PubMed]
- Tanabe E, Kitayoshi M, Hirane M, et al. Downregulation of activation factors of endothelia and fibroblasts via lysophosphatidic acid signaling in a mouse lung cancer LL/2 cell line. J Recept Signal Transduct Res 2013;33:286-90. [PubMed]
- Tanyi JL, Hasegawa Y, Lapushin R, et al. Role of decreased levels of lipid phosphate phosphatase-1 in accumulation of lysophosphatidic acid in ovarian cancer. Clin Cancer Res 2003;9:3534-45. [PubMed]
- Fang X, Schummer M, Mao M, et al. Lysophosphatidic acid is a bioactive mediator in ovarian cancer. Biochim Biophys Acta 2002;1582:257-64. [PubMed]
- Erickson JR, Hasegawa Y, Fang X, et al. Lysophosphatidic acid and ovarian cancer: A paradigm for tumorogenesis and patient management. Prostaglandins Other Lipid Mediat 2001;64:63-81. [PubMed]
- Schwartz BM, Hong G, Morrison BH, et al. Lysophospholipids increase interleukin-8 expression in ovarian cancer cells. Gynecol Oncol 2001;81:291-300. [PubMed]
- So J, Navari J, Wang FQ, et al. Lysophosphatidic acid enhances epithelial ovarian carcinoma invasion through the increased expression of interleukin-8. Gynecol Oncol 2004;95:314-22. [PubMed]
- Shida D, Kitayama J, Yamaguchi H, et al. Lysophosphatidic acid (LPA) enhances the metastatic potential of human colon carcinoma DLD1 cells through LPA1. Cancer Res 2003;63:1706-11. [PubMed]
- Lee Z, Swaby RF, Liang Y, et al. Lysophosphatidic acid is a major regulator of growth-regulated oncogene α in ovarian cancer. Cancer Res 2006;66:2740-8. [PubMed]
- Stoddard NC, Chun J. Promising pharmacological directions in the world of lysophosphatidic acid signaling. Biomol Ther (Seoul) 2015;23:1-11. [PubMed]
- Vici P, Mariani L, Pizzuti L, et al. Emerging biological treatments for uterine cervical carcinoma. J Cancer 2014;5:86-97. [PubMed]
- Tarallo V, De Falco S. The vascular endothelial growth factors and receptors family: Up to now the only target for anti-angiogenesis therapy. Int J Biochem Cell Biol 2015;64:185-9. [PubMed]
- Penson RT, Huang HQ, Wenzel LB, et al. Bevacizumab for advanced cervical cancer: Patient-reported outcomes of a randomised, phase 3 trial (NRG Oncology-Gynecologic Oncology Group protocol 240). Lancet Oncol 2015;16:301-11. [PubMed]
- Tewari KS, Sill MW, Long HJ 3rd, et al. Improved survival with bevacizumab in advanced cervical cancer. N Engl J Med 2014;370:734-43. [PubMed]
- Tewari KS, Monk BJ. New strategies in advanced cervical cancer: From angiogenesis blockade to immunotherapy. Clin Cancer Res 2014;20:5349-58. [PubMed]
- Bergers G, Hanahan D. Modes of resistance to anti-angiogenic therapy. Nat Rev Cancer 2008;8:592-603. [PubMed]
- Bikfalvi A, Moenner M, Javerzat S, et al. Inhibition of angiogenesis and the angiogenesis/invasion shift. Biochem Soc Trans 2011;39:1560-4. [PubMed]
- Gökmen-Polar Y, Goswami CP, Toroni RA, et al. Gene expression analysis reveals distinct pathways of resistance to bevacizumab in xenograft models of human ER-positive breast cancer. J Cancer 2014;5:633-45. [PubMed]
- Mésange P, Poindessous V, Sabbah M, et al. Intrinsic bevacizumab resistance is associated with prolonged activation of autocrine VEGF signaling and hypoxia tolerance in colorectal cancer cells and can be overcome by nintedanib, a small molecule angiokinase inhibitor. Oncotarget 2014;5:4709-21. [PubMed]
- Denny LA. Questions we should be asking about bevacizumab for cervical cancer. Oncology (Williston Park) 2015;29:367-8. [PubMed]
- Minion LE, Bai J, Monk BJ, et al. A Markov model to evaluate cost-effectiveness of antiangiogenesis therapy using bevacizumab in advanced cervical cancer. Gynecol Oncol 2015;137:490-6. [PubMed]
- Schmidt C. Cediranib aims for a comeback. J Natl Cancer Inst 2015;107. [PubMed]
- Serrano-Olvera A, Cetina L, Coronel J, et al. Emerging drugs for the treatment of cervical cancer. Expert Opin Emerg Drugs 2015;20:165-82. [PubMed]
- Lu J, Xiao YJ, Baudhuin LM, et al. Role of ether-linked lysophosphatidic acids in ovarian cancer cells. J Lipid Res 2002;43:463-76. [PubMed]