Biological effects of prostaglandin E2-EP4 antagonist (AAT-008) in murine colon cancer in vivo: enhancement of immune response to radiotherapy and potential as a radiosensitizer
Highlight box
Key findings
• A newly developed antagonist of PGE2-EP4 (AAT-008) has the potential to enhance the radiosensitivity of colon cancer cells by stimulating the immune system against the cancer cells.
What is known and what is new?
• Some studies showed that cyclooxygenase-2 inhibitors had the radiosensitizing effect.
• Regarding the subtypes of PGE2 receptors (EP1-4), we demonstrated that a PGE2-EP4 antagonist (AAT-008) with a high selectivity and a potent-binding affinity to EP4 receptors potentially had the radiosensitizing effect.
What is the implication, and what should change now?
• In future, clinical results of radiotherapy might improve by adding AAT-008 in the treatment of colon cancer.
Introduction
Radiotherapy is an important treatment modality for various malignant tumors, but recurrences after radiotherapy are often encountered and some tumors are resistant to the combination with chemotherapy and/or molecular-targeted therapy. Radiotherapy activates anti-tumoral immune responses against tumors, and effector CD8+ T cells (Teff) play an important role (1). The theory of cancer-immunity cycle, i.e., release of tumor-derived antigen by radiotherapy, antigen presentation for dendritic cells (DC), priming and proliferation of Teff, infiltration of Teff to tumors, and killing tumor cells by Teff, provides the rationale for the immunological effect of radiotherapy (2,3). Therefore, combination of radiotherapy and drugs activating the cancer-immunity cycle may be a promising strategy (4).
Prostaglandin E2 (PGE2) is an eicosanoid produced by cyclooxygenases. Some studies including a clinical trial demonstrated the radiosensitizing effect of cyclooxygenase-2 inhibitors (5,6). These studies suggested that PGE2 receptors were related to the radiosensitizing effect. To our knowledge, however, published data that indicate which subtype of PGE2 receptors (EP1–4) plays the role remain scarce (7).
The relationship between PGE2 and cancer progression has been reported via malignancy signaling with a subtype of PGE2 receptor EP4 (3,8-10). The PGE2 receptor EP4 expresses on the surface of immune cells in addition to tumor cells and the signaling takes part in the regulation of anti-tumoral immune responses, e.g., inhibition of DC recruitment in and around the tumor. The DCs promote the production of Teff and infiltration of Teff into the tumor, while the EP4 signal activates the regulatory T cells (Treg) (3). As a result, the EP4 signal weakens anti-tumoral immune responses by blocking some points of the cancer-immunity cycle induced by radiotherapy.
In recent years, an antagonist of PGE2-EP4 (AAT-008, Figure 1) was newly developed for possible use in clinics (11). We hypothesized that activating the cancer-immunity cycle using AAT-008 would potentiate anti-tumoral effect by radiotherapy. The primary purpose of the present study was to investigate the biological effects of AAT-008 as a radiosensitizer in the treatment of mouse colon cancer (CT26WT) cells in vivo. The secondary purpose was to explore the radiosensitizing mechanism by examining Teff and Treg in the tumor using flow cytometry (FCM). We present the following article in accordance with the ARRIVE reporting checklist (available at https://tcr.amegroups.com/article/view/10.21037/tcr-22-1857/rc).
Methods
Tumor line, mouse, and drugs
CT26WT cells (a mouse colon cancer line) purchased from the American Type Culture Collection were used throughout the study (12). For in vivo studies, 6-week-old female Balb/c mice purchased from Nihon SLC Co. Ltd. (Hamamatsu, Japan) were bred under semi-sterilized conditions. CT26WT cells were cultured in a humidified atmosphere at 37°C and 5% CO2 with Nissui’s Roswell Park Memorial Institute medium containing 10% fetal bovine serum. AAT-008 was provided by AskAt Inc., Nagoya, Japan. For oral administration in vivo, the drug was dissolved in 0.5% methyl cellulose. All animal experiments were performed under project licenses (Nos. H29M-10 and 30-012-007) granted by the Animal Ethics Committees of Nagoya City University and Osaka University, respectively, in compliance with Japanese Fundamental Guidelines for Proper Conduct of Animal Experiment and Related Activities in Academic Research.
Tumor growth delay assay
Exponentially growing 5×105 CT26WT tumor cells cultured in vitro were injected into the right hind legs of 7-week-old Balb/c mice. Treatments were initiated when the mean tumor diameter reached approximately 10 or 15 mm for tumor growth delay assay (day 0). The tumor-bearing mice were randomly allocated to various treatment groups, with differing tumor volumes evenly distributed among the groups. AAT-008 at doses of 3, 10, and 30 mg/kg/day was administered orally once daily in the first experiment and twice daily in the second experiment until termination of tumor size measurement (from day 0 to up to day 18). The counterpart groups of mice received oral vehicle (methyl cellulose) administration with the same schedules. The tumors were locally irradiated at 9 Gy on day 3 for the radiotherapy (RT) group using an X‐ray machine (210 kVp, 10 mA with a 2‐mm Al filter; Chubu Medical Co., Matsusaka, Japan) at a dose rate of 2.2 Gy/min as described previously (Figure S1) (13-15). On the day of irradiation, AAT-008 was administered 3 hours before irradiation to avoid any radioprotective effect caused by oral vehicle administration (16). In the tumor growth delay assay, 6 or 12 mice were used for each group. The three dimensions of each tumor were measured every other day using a caliper, and the tumor volumes were calculated as V = π/6 × products of the three dimensions as described in detail previously (13-15). The tumor doubling times were calculated from these data.
Flow cytometry
We conducted two series of flow cytometric analysis. The mean tumor size was 6–7 mm on day 0 and 8–11 mm on day 13–19. For the first series, the dose of AAT-008 was fixed at 10 mg/kg/day, and AAT-008 was administered twice a day from day 0 to day 18. Radiation (9 Gy) was given on day 3. Six mice were scheduled to be used for each group, but mice that weakened due to oral gavage and those with a tumor that was too large were excluded before analysis for humane endpoint. Each tumor was surgically removed on day 19, and the population of effector T cells (CD45+CD8+CD69+: Teff) in the tumor was investigated as described previously (17). Briefly, tumor cells digested by collagenase IV (Sigma Aldrich; Tokyo, Japan) with DNAse were incubated in Hanks’ balanced salt solution to prepare a single cell suspension. Anti-mouse CD16/32 antibody (Biolegend, San Diego, CA, USA) was added to block Fc receptors. Then anti-mouse rat CD45-FITC, anti-mouse rat CD69-PE, and anti-mouse rat CD8-APC antibodies (Clone 53-6.7; eBioscience, San Diego, CA, USA) were reacted on ice for 30 min in the dark. The stained cells were analyzed with a FACS Verse™ (Beckton Dickinson, Franklin Lakes, NJ, USA) flow cytometer. Analysis was performed using FlowJo ver. 10 (Tommy Digital Biology, Tokyo, Japan).
For the second FCM series, the dose of AAT-008 was fixed to 30 mg/kg/day to increase the immune responses. AAT-008 was administered twice daily from day 0 to 12, and the tumors were removed on day 13. Five mice were scheduled for use in each group. To analyze Treg, the anti-mouse rat CD4-APC antibody (RM4-5, eBioscience, CA, USA) was reacted on ice for 30 min in the dark. The cells were then fixed, permeabilized using FOXP3/Transcription Factor Staining Buffer Set (eBioscience), and reacted with anti-FoxP3-PE antibody (clone: FJK-16s, eBioscience, CA, USA). The population of Teff and the ratio of Teff/Treg cells [forkhead box protein p3 (FoxP3+), CD4+] were investigated using the tumor cells on day 13. The FCM procedure was the same as in the first series.
Statistical analysis
All data represent the mean ± standard error. In the tumor growth delay assay, differences in tumor doubling times between the AAT-008 alone and no treatment groups and between the combination therapy (AAT-008 + RT) and RT alone groups were evaluated using Williams’ multiple comparisons test. To estimate whether the combined effects of RT and AAT-008 were additive, supra-additive (synergistic), or sub-additive, two-way analysis of covariance was used. In the flow cytometry, Student’s t-test with Bonferroni correction was used to evaluate the differences in cell populations between the combination therapy and RT alone groups, or between the vehicle alone and AAT-008 groups. The software R Version 4.0.3 (The R Foundation for Statistical Computing, Vienna, Austria) was used to perform statistical analyses. Differences with a P value <0.05 were considered significant.
Results
Tumor growth delay assay
Figure 2 shows the results of the first growth delay assay to test the effects of AAT-008 alone given once daily at doses of 10 and 30 mg/kg/day and in combination with radiation (9 Gy). The mean tumor size on day 0 was 15 mm. The mean tumor doubling times in mice receiving vehicle, 10 mg/kg AAT-008, and 30 mg/kg AAT-008 were 5.9, 6.3, and 6.9 days, respectively, in the unirradiated group and 8.8, 11.0, and 18.2 days, respectively, in the irradiated group. The growth delay effect seemed minimal when 10 or 30 mg/kg/day of AAT-008 was given alone. However, the growth delay effects of RT plus 30 mg/kg/day of AAT-008 were significant compared with RT alone (P<0.05). The combined effect was considered additive at the AAT-008 dose of 30 mg/kg/day.
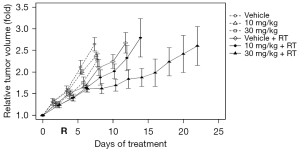
Figure 3 shows the results of the second growth delay assay to test the effects of AAT-008 given alone twice daily at doses of 3, 10, and 30 mg/kg/day and in combination with radiation (9 Gy). The mean tumor size on day 0 was 10 mm. The mean tumor doubling times in mice receiving vehicle, 3 mg/kg AAT-008, 10 mg/kg AAT-008, and 30 mg/kg AAT-008 were 4.0, 4.4, 4.6, and 5.5 days, respectively, in the unirradiated group and 6.1, 7.7, 16.5, and 21.1 days, respectively, in the irradiated group. The growth delay effect of AAT-008 (30 mg/kg/day) administered alone was mild but statistically significant (P<0.05). The growth delay effects of RT plus AAT-008 (especially at 10 and 30 mg) were significant compared with RT alone (P<0.05). The combined effect was considered additive at the AAT-008 doses of 3 and 10 mg/kg/day, and supra-additive at 30 mg/kg/day.
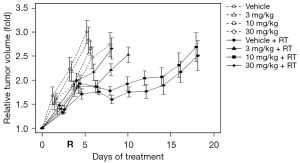
Flow cytometry
In the first FCM series, the mean Teff proportions in the irradiated tumors were 43% and 31% in the 10 mg + RT (n=4) and 0 mg + RT groups (n=5), respectively. Notably, ≥60% Teff was observed in responsive mice (n=2) in the 10 mg + RT group (Figure 4). In the second FCM series (Figure 5, Figure S2), the mean Treg proportions in the irradiated tumors were 4.0% and 1.5% in the 0 mg + RT (n=5) and 30 mg + RT groups (n=5), respectively (P=0.04). The corresponding Teff/Treg ratios were 10 and 22, respectively (P=0.04).
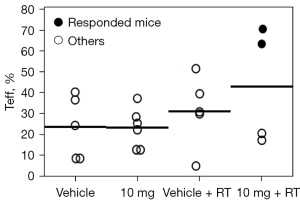
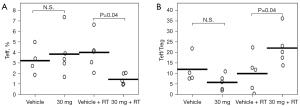
Discussion
To the best of our knowledge, this is the first study to evaluate the radiosensitizing ability of AAT-008. Based on the results, AAT-008 has potential as a radiosensitizer. Since AAT-008 has a high binding selectivity and a potent binding affinity for EP4 receptor (11), it was assumed that this effect was obtained via EP4 receptor. In the first growth delay experiment, the combined effect of RT and AAT-008 was not sufficient, so in the second experiment we used smaller tumors and AAT-008 was administered twice daily; the combined effect appeared to be supra-additive at the dose of 30 mg/kg/day and it appeared that at least 10 mg/kg/day is required to obtain a sufficient tumor growth delay effect when combined with radiotherapy in this murine colon cancer model. Our data also suggest that the immune system was stimulated by AAT-008 against the cancer cells when combined with radiotherapy.
A few recent studies have demonstrated that radiation enhances antitumor immune response through several mechanisms, including induction of damage associated molecular patterns and activation of the type-I interferon pathway (18,19). The enhanced immune response was highly dependent on total radiation doses to tumors and fractionation schedules as well as tumor types (18-21). Because the primary purpose of the present study was to investigate the radiosensitizing effect of AAT-008 on irradiated tumor growth, we employed a radiation dose that did not control the tumors. Additionally, extremely high dose irradiation in a single fraction (e.g., 30 Gy) will not induce the type-I interferon pathway and recruit adequate numbers of Teff due to severe vascular damage and degradation of inflammatory cytokines (18). Therefore, we used 9 Gy in a single fraction in all experiments.
Previous clinical reports demonstrated that neoadjuvant chemoradiotherapy increased the proportion of CD8+ T cells in the whole lymphocytes in other tumors (22,23). A recent preclinical study using a pancreatic ductal carcinoma mouse model demonstrated that local irradiation at 16 Gy in a single fraction and three fractions of 8 Gy increased not only Teff but also Treg in irradiated tumors, indicating that radiation altered the tumor immune microenvironment to immune evasion as well as immune activation (24). Similarly, in a colon cancer mouse model, radiation at 12 Gy in a single fraction clearly promoted the accumulation of Treg (25). These findings suggest that an additional strategy to reduce Treg and increase Teff is likely to enhance the radiosensitizing effect.
EP4 antagonists stimulate CD8+ T cell infiltration to tumors and reduce Treg (3). Thus, we hypothesized that AAT-008 may improve the antitumor effect with RT. A clinical study demonstrated that patients with high CD8+ T cell infiltration either pre-RT or post-10 Gy exhibited positive associations with overall survival, progression-free survival, and local control rates (26). In 115 patients with cervical cancer, a high number of Treg and a low CD8+ T cell/Treg ratio in the intraepithelial tumor-infiltrating lymphocytes were reported to be poor prognostic factors (27). Similarly, our results demonstrated that addition of AAT-008 to radiation enhanced the local antitumor response with increased CD8+ T cell/Treg ratios.
The combination of chemoradiotherapy and immune checkpoint inhibitors (ICI) is now a standard treatment in locally-advanced non-small cell lung cancer (28). Results from some ongoing clinical studies of combined radiotherapy and ICI in the treatment of colorectal cancer are awaited (29). However, the targets of ICI are limited in the cancer-immunity cycle. PGE2-EP4 antagonists such as AAT-008 were effective on other points of the cancer-immunity cycle: stimulating dendritic cells and CD8+ T cell infiltration to the tumor, inhibiting suppressive immune cells, and rescuing CD8+ T cell functions (3). Thus, AAT-008 is expected to enhance the therapeutic effects of combined radiotherapy and ICI. AAT-008 has been developed for clinical use, and further investigation including clinical trials is warranted. EP4 is reported to play critical roles in hematopoiesis and blood cell trafficking (9,30). However, no hematological safety concerns were observed regarding AAT-008 in in vivo safety and toxicity studies (data not shown). Similar safety evidence of a selective EP4 antagonist (grapiprant/Galliprant® for animal health) was also demonstrated in field studies using dogs with disease (31). Recently, other EP4 antagonists have been developed (32,33). AAT-008 has a great binding potency for EP4 receptors and has more than 1,000-fold higher selectivity than other prostaglandin receptors (11). We assumed that AAT-008 was useful to bring out the effectiveness via suppression of EP4 receptors compared to other EP4 antagonists. Moreover, AAT-008 is ready to be used in clinical situations. These are the benefits to use AAT-008 compared to other EP4 antagonists.
A limitation of the present study is that only one tumor cell line was used. The results could be different with other cell lines. Another issue is that other immunologic mechanisms could also be related to the radiosensitizing ability. Further investigation would be warranted using more detailed flow cytometry analysis, transgenic mouse models, and additional cancer models including human cancer cell lines. To strengthen the evidence of radiosensitivity, further investigations using lower radiation doses are desirable. In this study, some mice were weakened due to oral gavage and excluded for humane endpoint. We could reduce the stress to mice using a novel oral gavage technique (34).
Conclusions
AAT-008 has the potential to enhance radiosensitivity in the treatment of colon cancer cells, by stimulating the immune system against the cancer cells.
Acknowledgments
We are grateful to Takako Okumura and Yukinori Take (AskAt Inc.) for technical assistance with the experiments. AAT-008 was provided by AskAt Inc. without any financial support.
Funding: This work was supported in part by JSPS KAKENHI (No. JP18K15285 to Dr. Y Manabe).
Footnote
Reporting Checklist: The authors have completed the ARRIVE checklist. Available at https://tcr.amegroups.com/article/view/10.21037/tcr-22-1857/rc
Data Sharing Statement: Available at https://tcr.amegroups.com/article/view/10.21037/tcr-22-1857/dss
Peer Review File: Available at https://tcr.amegroups.com/article/view/10.21037/tcr-22-1857/prf
Conflicts of Interest: All authors have completed the ICMJE uniform disclosure form (available at https://tcr.amegroups.com/article/view/10.21037/tcr-22-1857/coif). YM reports grants from JSPS KAKENHI (No. JP18K15285) during the conduct of the study and that AAT-008 was provided by AskAt Inc. The other authors have no conflicts of interest to declare.
Ethical Statement: The authors are accountable for all aspects of the work in ensuring that questions related to the accuracy or integrity of any part of the work are appropriately investigated and resolved. All animal experiments were performed under project licenses (Nos. H29M-10 and 30-012-007) granted by the Animal Ethics Committees of Nagoya City University and Osaka University, respectively, in compliance with Japanese Fundamental Guidelines for Proper Conduct of Animal Experiment and Related Activities in Academic Research.
Open Access Statement: This is an Open Access article distributed in accordance with the Creative Commons Attribution-NonCommercial-NoDerivs 4.0 International License (CC BY-NC-ND 4.0), which permits the non-commercial replication and distribution of the article with the strict proviso that no changes or edits are made and the original work is properly cited (including links to both the formal publication through the relevant DOI and the license). See: https://creativecommons.org/licenses/by-nc-nd/4.0/.
References
- Gupta A, Probst HC, Vuong V, et al. Radiotherapy promotes tumor-specific effector CD8+ T cells via dendritic cell activation. J Immunol 2012;189:558-66. [Crossref] [PubMed]
- Chen DS, Mellman I. Oncology meets immunology: the cancer-immunity cycle. Immunity 2013;39:1-10. [Crossref] [PubMed]
- Take Y, Koizumi S, Nagahisa A, Prostaglandin E. Receptor 4 Antagonist in Cancer Immunotherapy: Mechanisms of Action. Front Immunol 2020;11:324. [Crossref] [PubMed]
- Gajiwala S, Torgeson A, Garrido-Laguna I, et al. Combination immunotherapy and radiation therapy strategies for pancreatic cancer-targeting multiple steps in the cancer immunity cycle. J Gastrointest Oncol 2018;9:1014-26. [Crossref] [PubMed]
- Cheki M, Yahyapour R, Farhood B, et al. COX-2 in Radiotherapy: A Potential Target for Radioprotection and Radiosensitization. Curr Mol Pharmacol 2018;11:173-83. [Crossref] [PubMed]
- Liao Z, Komaki R, Milas L, et al. A phase I clinical trial of thoracic radiotherapy and concurrent celecoxib for patients with unfavorable performance status inoperable/unresectable non-small cell lung cancer. Clin Cancer Res 2005;11:3342-8. [Crossref] [PubMed]
- Bao X, Albu DI, Huang KC, et al. Combination of a novel EP4 antagonist E7046 and radiation therapy promotes anti-tumor immune response and tumor rejection in preclinical tumor models. Int J Radiol Oncol Biol Phys 2016;96:S128. [Crossref]
- Ching MM, Reader J, Fulton AM. Eicosanoids in Cancer: Prostaglandin E(2) Receptor 4 in Cancer Therapeutics and Immunotherapy. Front Pharmacol 2020;11:819. [Crossref] [PubMed]
- Yokoyama U, Iwatsubo K, Umemura M, et al. The prostanoid EP4 receptor and its signaling pathway. Pharmacol Rev 2013;65:1010-52. [Crossref] [PubMed]
- Fujino H. The Roles of EP4 Prostanoid Receptors in Cancer Malignancy Signaling. Biol Pharm Bull 2016;39:149-55. [Crossref] [PubMed]
- Okumura Y, Yamagishi T, Nukui S, et al. Discovery of AAT-008, a novel, potent, and selective prostaglandin EP4 receptor antagonist. Bioorg Med Chem Lett 2017;27:1186-92. [Crossref] [PubMed]
- Wang M, Bronte V, Chen PW, et al. Active immunotherapy of cancer with a nonreplicating recombinant fowlpox virus encoding a model tumor-associated antigen. J Immunol 1995;154:4685-92. [Crossref] [PubMed]
- Shibamoto Y, Murata R, Miyauchi S, et al. Combined effect of clinically relevant doses of emitefur, a new 5-fluorouracil derivative, and radiation in murine tumours. Br J Cancer 1996;74:1709-13. [Crossref] [PubMed]
- Takaoka T, Shibamoto Y, Matsuo M, et al. Biological effects of hydrogen peroxide administered intratumorally with or without irradiation in murine tumors. Cancer Sci 2017;108:1787-92. [Crossref] [PubMed]
- Kondo T, Shibamoto Y, Kawai T, et al. Effects of a combined treatment regimen consisting of Hsp90 inhibitor DS-2248 and radiation in vitro and in a tumor mouse model. Transl Cancer Res 2021;10:2767-76. [Crossref] [PubMed]
- Shibamoto Y, Miyauchi S, Kiyokawa H, et al. Oral vehicle administration before radiation causes radioprotection of murine SCCVII tumors. Oncol Rep 1997;4:787-9. [Crossref] [PubMed]
- Takenaka W, Takahashi Y, Tamari K, et al. Radiation Dose Escalation is Crucial in Anti-CTLA-4 Antibody Therapy to Enhance Local and Distant Antitumor Effect in Murine Osteosarcoma. Cancers (Basel) 2020.
- Vanpouille-Box C, Alard A, Aryankalayil MJ, et al. DNA exonuclease Trex1 regulates radiotherapy-induced tumour immunogenicity. Nat Commun 2017;8:15618. [Crossref] [PubMed]
- Sharabi AB, Lim M, DeWeese TL, et al. Radiation and checkpoint blockade immunotherapy: radiosensitisation and potential mechanisms of synergy. Lancet Oncol 2015;16:e498-509. [Crossref] [PubMed]
- Brix N, Tiefenthaller A, Anders H, et al. Abscopal, immunological effects of radiotherapy: Narrowing the gap between clinical and preclinical experiences. Immunol Rev 2017;280:249-79. [Crossref] [PubMed]
- Lee BM, Seong J. Radiotherapy as an immune checkpoint blockade combination strategy for hepatocellular carcinoma. World J Gastroenterol 2021;27:919-27. [Crossref] [PubMed]
- Teng F, Mu D, Meng X, et al. Tumor infiltrating lymphocytes (TILs) before and after neoadjuvant chemoradiotherapy and its clinical utility for rectal cancer. Am J Cancer Res 2015;5:2064-74. [PubMed]
- Yoneda K, Kuwata T, Kanayama M, et al. Alteration in tumoural PD-L1 expression and stromal CD8-positive tumour-infiltrating lymphocytes after concurrent chemo-radiotherapy for non-small cell lung cancer. Br J Cancer 2019;121:490-6. [Crossref] [PubMed]
- Yamamoto J, Takahashi Y, Minami K, et al. High dose local photon irradiation is crucial in anti-CTLA-4 antibody therapy to enhance the abscopal response in a curine pancreatic carcinoma model. Cancers 2022;14:2087. [Crossref] [PubMed]
- Ji D, Song C, Li Y, et al. Combination of radiotherapy and suppression of Tregs enhances abscopal antitumor effect and inhibits metastasis in rectal cancer. J Immunother Cancer 2020;8:e000826. [Crossref] [PubMed]
- Mori Y, Sato H, Kumazawa T, et al. Analysis of radiotherapy-induced alteration of CD8(+) T cells and PD-L1 expression in patients with uterine cervical squamous cell carcinoma. Oncol Lett 2021;21:446. [Crossref] [PubMed]
- Jordanova ES, Gorter A, Ayachi O, et al. Human leukocyte antigen class I, MHC class I chain-related molecule A, and CD8+/regulatory T cell ratio: which variable determines survival of cervical cancer patients? Clin Cancer Res 2008;14:2028-35. [Crossref] [PubMed]
- Antonia SJ, Villegas A, Daniel D, et al. Overall Survival with Durvalumab after Chemoradiotherapy in Stage III NSCLC. N Engl J Med 2018;379:2342-50. [Crossref] [PubMed]
- Manz SM, Losa M, Fritsch R, et al. Efficacy and side effects of immune checkpoint inhibitors in the treatment of colorectal cancer. Therap Adv Gastroenterol 2021;14:17562848211002018. [Crossref] [PubMed]
- Ikushima YM, Arai F, Hosokawa K, et al. Prostaglandin E(2) regulates murine hematopoietic stem/progenitor cells directly via EP4 receptor and indirectly through mesenchymal progenitor cells. Blood 2013;121:1995-2007. [Crossref] [PubMed]
- Rausch-Derra L, Huebner M, Wofford J, et al. A Prospective, Randomized, Masked, Placebo-Controlled Multisite Clinical Study of Grapiprant, an EP4 Prostaglandin Receptor Antagonist (PRA), in Dogs with Osteoarthritis. J Vet Intern Med 2016;30:756-63. [Crossref] [PubMed]
- Wang W, He J, Yang J, et al. Scaffold Hopping Strategy to Identify Prostanoid EP4 Receptor Antagonists for Cancer Immunotherapy. J Med Chem 2022;65:7896-917. [Crossref] [PubMed]
- Peng S, Hu P, Xiao YT, et al. Single-Cell Analysis Reveals EP4 as a Target for Restoring T-Cell Infiltration and Sensitizing Prostate Cancer to Immunotherapy. Clin Cancer Res 2022;28:552-67. [Crossref] [PubMed]
- Hoggatt AF, Hoggatt J, Honerlaw M, et al. A spoonful of sugar helps the medicine go down: a novel technique to improve oral gavage in mice. J Am Assoc Lab Anim Sci 2010;49:329-34. [PubMed]