CTBP2 contributes to cisplatin resistance in lung adenocarcinoma by inhibiting generation of reactive oxygen species
Highlight box
Key findings
• C-terminal binding protein 2 (CTBP2) is involved in conferring resistance to cisplatin (CP) in non-small cell lung cancer and that CTBP2 may be an innovative therapeutic target for managing CP resistance.
What is known and what is new?
• High expression of CTBP2 was associated with the CP resistance in lung adenocarcinoma.
• Downregulation of CTBP2 increased the sensitivity of drug-resistant to CP, which is attributed to a reduction in mitochondrial membrane potential levels and an elevation in reactive oxygen species production.
What is the implication, and what should change now?
• The role of CTBP2 in CP resistance needs further study.
Introduction
Cisplatin (CP), a platinum-based compound, is commonly utilized as a primary chemotherapeutic agent in treating lung cancer (1). However, the frequent utilization of CP has resulted in the emergence of resistance, which has been recognized as a contributing factor to the ineffectiveness of lung cancer treatments. Resistance to CP is associated with multiple complex system properties, such as enhanced drug efflux transporter activity (2), overexpression of copper transporter 1 (CTR1) (3), and importantly nucleotide excision repair (NER) mechanism (4). However, the molecular basis of CP resistance is still poorly understood. It is imperative to carry out further investigations to elucidate the fundamental mechanisms of CP resistance.
C-terminal binding protein 2 (CTBP2), a transcriptional co-repressor, has been found to participate in several physiological and pathological processes (5). Several recent studies have found that the excessive expression of CTBP2 enhances tumor cell proliferation, invasion, and poor outcome in various types of tumor (6-8). Furthermore, CTBP2 has been revealed to be a promising therapeutic target that can be used to treat various neoplasms (8-10). In a recent study, CTBP2 was found to be a poor prognostic marker for lung adenocarcinomas (LUADs). Patients with CTBP2 overexpression tended to live shorter than those with low levels of CTBP2 (11). Moreover, overexpression of CTBP2 inhibited the apoptosis of esophageal cancer cells induced by CP through decrease in p53, Caspase 3, Caspase 8, and Bax levels, while enhancing Bcl-2 expression (12). Nevertheless, the exact function of CTBP2 in conferring CP resistance in LUAD remains unelucidated.
We aimed to enhance our comprehension of the LUAD CP resistance mechanism by examining the impact of CTBP2 on CP resistance in CP-resistant cells (A549/DDP). Our results indicated that A549/DDP cells exhibited an upregulation of CTBP2. Downregulation of CTBP2 resulted in a reduction in mitochondrial membrane potential (MMP) and an elevation in cellular reactive oxygen species (ROS) production, thereby augmenting A549/DDP cell sensitivity to CP. The findings indicate that CTBP2 is involved in conferring resistance to CP in non-small cell lung cancer (NSCLC) and that CTBP2 may be an innovative therapeutic target for managing CP resistance in this context. We present this article in accordance with the MDAR reporting checklist (available at https://tcr.amegroups.com/article/view/10.21037/tcr-23-2135/rc).
Methods
Examining the CTBP2 expression in LUAD
The Gene Expression Profiling Interactive Analysis (GEPIA) database was employed to validate CTBP2 expression levels in both LUAD and normal tissue samples obtained from The Cancer Genome Atlas (TCGA) and Genotype-Tissue Expression (GTEx), with a significance threshold of P≤0.05 and an absolute value of log2FC ≥2. Additionally, the University of ALabama at Birmingham CANcer data analysis Portal (UALCAN) was employed to investigate CTBP2 expression replying on the data obtained from the TCGA database. The study was conducted in accordance with the Declaration of Helsinki (as revised in 2013).
Survival analysis of CTBP2 in patients with LUAD
The study employed the Kaplan-Meier (K-M) plot for evaluating the differences in overall survival (OS), first progression survival (FPS), and post-progression survival (PPS) among two groups categorized as high and low CTBP2 expression groups. The statistical significance level was established at a threshold of P<0.05.
CP sensitivity analysis of CTBP2 in LUAD
The Cancer Cell Line Encyclopedia was employed to analyze the CTBP2 gene expression in non-small cell lung cancer (NSCLC) cells. Furthermore, the CP sensitivity of these NSCLC cells were detected by the Genomics of Drug Sensitivity in Cancer Project.
Cell lines and antibodies
A549 cells were procured from Nanjing Cobioer Co., Ltd. (Nanjing, China, Cat No. CBP60084) and went through culture in complete F12K medium (Boster, Wuhan, China, Cat No. PYG0055) at a temperature of 37 ℃ and with a 5% CO2 environment. A549/DDP cells, CP-resistant cells, were established following the previously described method (13), where the cells were subjected to culture in a complete F12K medium containing 2.5 µM CP (Beyotime, Shanghai, China, S1552). The following antibodies were utilized in our study CTBP2 (ZEN BIO, Chengdu, China, Cat No. R26639), Bcl-2 [Cell Signaling Technology (CST), MA, USA, Cat No. 3498S], Bax (CST, Cat No. 41162S), Cleaved-PARP (CST, Cat No. 32563S), Cleaved-Caspase 3 (ZEN BIO, Cat No. 341034), beta-tubulin (ZEN BIO, Cat No. 250175), GAPDH (ZEN BIO, Cat No. R24404).
Short hairpin RNA (shRNA) and transfection
The CTBP2 expression was suppressed through the utilization of shRNA. The sequences employed in our study were as follows: shCTBP2-1 (5′GCCTTTGGATTCAGCGTCATA3′), shCTBP2-2 (5′CCTGAGAGTGATCGTGCGGAT3′). Lentiviruses containing shCTBP2 vectors were packaged in 293T cells. The A549/DDP cells were seeded into six-well plates at 5×105 cells per well and subjected to inoculation of lentiviruses mixed with polybrene (Beyotime, Cat No. C0351). Following this, the cells were treated with 5 µg puromycin dihydrochloride (Beyotime, Cat No. ST551) to ensure stable integration.
Cell proliferation assay
A549/DDP and A549/DDP-shCTBP2 cells went through seeding in 96-well plates (5×103 cells/well) and followed by overnight incubation. Subsequently, the cells underwent a 48 h exposure to different amounts of CP (0, 0.625, 1.25, 2.5, 5, 10, 20, 40 µM). Upon completion of the incubation period, the cell viability was conducted using 3-(4,5-dimethylthiazole)-2,5-diphenyltetrazolium bromide (MTT) assay.
Colony formation
A549/DDP or A549/DDP-shCTBP2 cells were maintained in a 6-well dish with 1,000 cells per well. To promote the formation of colonies, the cells underwent a 14-day exposure to CP at a concentration of 5 µM. The colonies were subjected to fixation and staining using a 0.1% solution of crystal violet at room temperature (RT). Subsequently, the colonies were imaged and counted using an Epson scanner and Image J, respectively.
RNA extraction and quantitative reverse transcription polymerase chain reaction (qRT-PCR)
The total RNA extraction was conducted through the RNA Easy Fast kit (TIANGEN, Beijing, China, Cat No. DP451) following the protocol. Subsequently, qRT-PCR was performed by BeyoFast™ SYBR Green One-Step qRT-PCR Kit (Beyotime, Cat No. D7268). Real-time PCR was conducted using the following primers: CTBP2_qF (5′GCAGGATTTGCTGTATCAGAGCG3′), CTBP2_qR (5′CTGCGTTCACAAGGAATGCTCC3′), GAPDH_qF (5′GTCTCCTCTGACTTCAACAGCG3′), and GAPDH_qR (5′ACCACCCTGTTGCTGTAGCCAA3′). The LightCycler96 detection system (Roche Molecular Systems, Inc., Basel, Switzerland) was utilized to identify SYBR fluorescence. A fold change was calculated by comparing CTBP2 relative messenger RNA (mRNA) levels to GAPDH mRNA levels.
Flow cytometry assay
The transfected cells went through seeding in a 6-well plate (2×105 cells/well) and subsequently received treatment with 5 µM CP. Following a 24-h incubation, the cells received trypsin digestion and were subsequently sensed twice with cold phosphate buffer saline (PBS). Following that, the cells were resuspended again in 500 µL of binding buffer followed by 15 min of incubation with 5 µL of FITC-Annexin V and 10 µl of propidium iodide (PI) at RT in darkness. To identify cell apoptosis, flow cytometry was employed using a BD Accuri C6 flow cytometer (BD Biosciences, San Jose, CA, USA).
Western blot (WB)
The RIPA lysis buffer (Beyotime, Cat No. P0013) was supplemented with a protease mixture inhibitor (MedChemExpress, Monmouth Junction, NJ, USA, Cat No. HY-K0010) for the purpose of extracting total cellular proteins. Afterward, a quantity of 20 µg of total proteins was separated on a 12% SDS-PAGE gel and subsequently transferred and fixed onto polyvinylidene Fluoride (PVDF) membranes (Millipore, Billerica, MA, USA, Cat No. ISEQ00010). Following this, the membranes were subjected to a blocking step using 5% nonfat milk at RT for 2 h. This was followed by overnight incubation at 4 ℃ with specific primary antibodies. Subsequently, the membranes were incubated with the appropriate IRDye® secondary antibodies at RT for an additional hour. The acquisition of blot images was carried out using an Odyssey® CLx imaging system (LI-COR, Lincoln, Nebraska, USA).
Measurement of intracellular ROS
Intracellular ROS was measured using 2’,7’-dichlorodihydrofluorescein diacetate (DCFH-DA, Beyotime, Cat No. S0033). A 12-well plate was utilized to seed cells at 1×105 cells/well density. Following overnight incubation, cells were subjected to a 24-h treatment with 5 µM CP. Subsequently, the cells received treatment with a concentration of 10 µM DCFH-DA for 30 min at a temperature of 37 ℃. The stained cells were instantly imaged using an inverted fluorescence microscope (Olympus BX53, Tokyo, Japan).
Measurement of MMP
The MMP of A549/DDP or A549/DDP-shCTBP2 cells was detected using 5,5′,6,6′-tetrachloro-1,1′,3,3′-tetraethyl-imidacarbocyanine iodide (JC-1) reagent (Beyotime, Cat No. C2005). The transfected cells were subjected to incubation with JC-1 solution at a temperature of 37 ℃ for 30 min. The nucleus was subsequently stained using a 4’,6-diamidino-2-phenylindole (DAPI) solution (Beyotime, Cat No. C1002) following the protocols. The fluorescence variations of JC-1 were observed and documented through the utilization of a fluorescence microscope from Olympus (Model BX53, Tokyo, Japan).
Statistical analysis
The statistical analyses were performed utilizing GraphPad Prism 9.0.0 (GraphPad Software, Inc. La Jolla, CA, USA). The statistical analysis of the differences between groups was performed through Student’s t-test. P<0.05 was determined to be significant for statistical comparisons.
Results
CTBP2 expression was higher in LUAD tissues than in normal lung tissues
The results obtained from both the GEPIA and UALCAN databases revealed that CTBP2 exhibited a significant elevation in LUAD tissues, in contrast to non-malignant tissues (Figure 1A,1B). Furthermore, the results obtained from the UALCAN data revealed that the expression of CTBP2 was higher in stage 1 to stage 4 compared to normal tissues, furthermore, the expression of CTBP2 in stage 3 and stage 4 increased compared to that in stage 1, illustrating that CTBP2 was associated with the LUAD stages (Figure 1C). CTBP2 expression was elevated in Caucasian, African-American, and Asian patients relative to all race normal (Figure 1D). However, there were no differences in CTBP2 expression between the sexes (Figure 1E). Figure 1F illustrates that CTBP2 was significantly overexpressed in patients with smoking and reformed smoking compared to patients with non-smoking. Importantly, CTBP2 was significantly overexpressed in metastatic foci compared to normal tissues; however, nodal metastasis status did not show significant differences (Figure 1G). Additionally, CTBP2 expression in patients with TP53 mutation was no different in contrast with that in patients with non-TP53 mutation (Figure 1H).
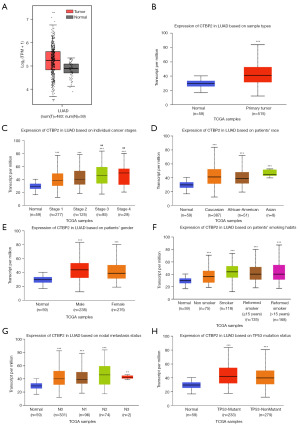
CTBP2 overexpression level in patients with LUAD was associated with a poor prognosis
The KM plot was utilized in the context of survival analysis to assess the relation between CTBP2 expression level and the outcome of patients diagnosed with LUAD. The OS curves revealed that in patients with LUAD, CTBP2 overexpression resulted in a significantly low survival probability (Figure 2A). Additionally, the FPS and PPS curves also indicated that overexpressed CTBP2 reflected a poor prognosis. Figure 2B,2C demonstrate that elevated CTBP2 content was linked to poor FPS and PPS, respectively. In summary, the analysis of survival curves demonstrated that CTBP2 overexpression is a reliable predictor of unfavorable prognosis among patients with LUAD.
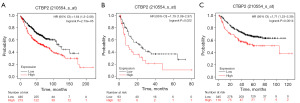
The relationship between CTBP2 expression and CP sensitivity in LUAD
We wondered whether different expression of CTBP2 in lung cancer cells were related to the sensitivity of CP. The data showed the expression of CTBP2 was different in various cell types (Figure 3A). Based on the median value of CTBP2 mRNA expression, the cells were divided into low- and high-expression groups (Figure 3B). Furthermore, CP sensitivity was evaluated on these cells by the datasets form Genomics of Drug Sensitivity in Cancer Project. As shown in Figure 3C,3D, the CP half maximal inhibitory concentration (IC50) values in high-expression CTBP2 cells were higher than that in low-expression cells. However, CTBP2 high expression cell lines had low IC50 values compared to CTBP2 low expression cells, the relationship between CTBP2 expression and CP sensitivity is not absolute.
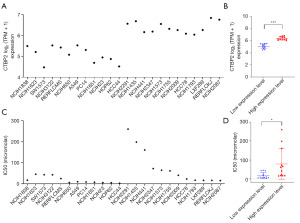
CTBP2 overexpression correlated to CP resistance in A549/DDP cell lines
For investigating the correlation between CTBP2 and CP resistance, A549/DDP cells were subjected to transfection with CTBP2 shRNA lentivirus, resulting in generating A549/DDP-shCTBP2 cells. Initially, the quantification of CTBP2 mRNA and protein expression was conducted through qRT-PCR and WB in both A549 and A549/DDP cell lines, revealing that both CTBP2 mRNA and protein expression levels were significantly higher in A549/DDP cells than in A549 cells (Figure 4A-4C). Meanwhile, a significant decrease in CTBP2 protein expression was observed in A549/DDP cells after the introduction of CTBP2 shRNA lentivirus (Figure 4D,4E). The sensitivity of CP in A549/DDP and A549/DDP-shCTBP2 cells was evaluated through the utilization of the MTT assay, revealing that A549/DDP-shCTBP2 cells exhibited a significant enhancement in their responsiveness to CP in comparison to A549/DDP cells (Figure 4F). The clone-formation assay results indicated that the presence of CTBP2 did not exert any influence on the colony count in A549/DDP in the absence of CP. Additionally, the number of A549/DDP-shCTBP2 cell colonies was observed to reduced significantly after treatment with CP compared to A549/DDP cells when exposed to 5 µM CP (Figure 4G,4H). The findings suggest that CTBP2 plays a pivotal role in the modulation of resistance to CP in NSCLC.
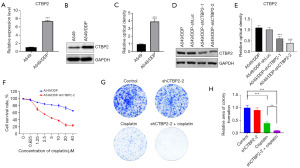
CTBP2 knockdown induced A549/DDP cell apoptosis in treatment with CP
The MTT assay results revealed that CTBP2 downregulation significantly enhanced A549/DDP cell sensitivity towards CP. Therefore, to validate the inhibitory effect of CTBP2 knockdown on A549/DDP cell proliferation in CP presence, the apoptotic status of cells was evaluated by annexin V-FITC/PI double staining and flow cytometry. The findings indicated that the transfection of shCTBP2 in A549/DDP cells led to a rise of ~22% in cell apoptosis, as compared to the transfection of shLuc when exposed to 5 µM CP (Figure 5A,5B). Furthermore, WB was employed for determining the proteins related to cell apoptosis, revealing that Cleaved-Caspase 3 was significantly overexpressed in the A549/DDP cells subjected to a 24-h treatment with 5 µM CP compared to the untreated control cells (Figure 5C,5D). It should be noted that Cleaved-Caspase 3 was significantly overexpressed in A549/DDP-shCTBP2 cells treated with 5 µM CP compared to A549/DDP cells treated with 5 µM CP. In addition, the A549/DDP-shCTBP2 cells treated with 5 µM CP exhibited a Bcl-2 suppression and Bax and Cleaved-PARP overexpression. The Bcl-2/Bax ratio exhibited a significant decrease in the A549/DDP-shCTBP2+CP group in comparison to the A549/DDP, A549/DDP+CP, and A549/DDP-shCTBP2 groups. Our findings suggest that CTBP2 inhibition increased A549/DDP cell apoptotic response to CP treatment. This effect was attributed to Bcl-2 suppression as well as Bax, Cleaved-PARP, and Cleaved-Caspase 3 overexpression.
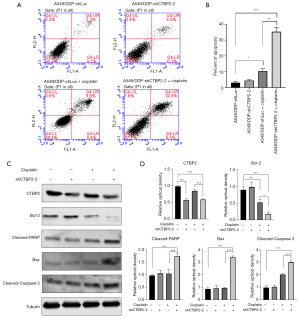
CTBP2 knockdown induced ROS-dependent mitochondrial dysfunction in the presence of CP
ROS detection was carried out to assess CTBP2-induced apoptotic mechanisms, revealing that shCTBP2 transfection and CP treatment induced a significant elevation in ROS production in A549/DDP cells (Figure 6A,6B). Furthermore, a JC-1 kit was utilized for detecting the MMP, indicating that transfection with shCTBP2 resulted in a significant reduction in the relative JC-1 polymer monomer fluorescence ratio when compared to A549/DDP cells treated with 5µM CP (Figure 6C,6D). The findings indicate that the downregulation of CTBP2 increased A549/DDP cell sensitivity to CP, which is attributed to a reduction in MMP levels and an elevation in ROS production.
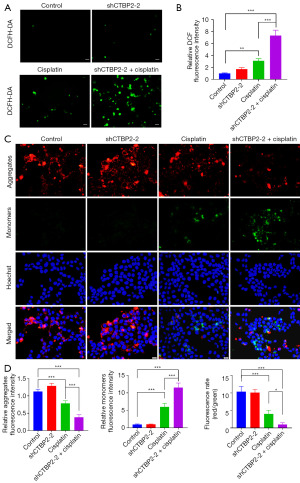
Discussion
The formation of a cross-linked complex between CP and DNA has been observed to elicit DNA damage, ultimately resulting in apoptosis and subsequent cell death in cancer cells. CP is a platinum-based chemotherapeutic agent that is commonly utilized as a primary treatment option for LUAD (14). Nevertheless, the frequent utilization of CP has led to an increased prevalence of resistance, which has been identified as a contributing factor to the ineffectiveness of LUAD treatment. Hence, it is necessary to identify a potential therapeutic target to address the issue of CP resistance in LUAD.
Prior research has demonstrated that the upregulation of CTBP2 expression was observed in LUAD tissues in comparison to adjacent non-tumorous tissues (10,11,15). The findings of our study, which employed bioinformatics analyses, indicated a significantly overexpressed CTBP2 in patients with LUAD as compared to those without malignancy and was correlated to LUAD stages I to IV. It should be noted that CTBP2 was found to be significantly overexpressed in patients with LUAD who smoke cigarettes. Based on the Cancer Cell Line Encyclopedia and the Genomics of Drug Sensitivity in Cancer Project databases, high expression of CTBP2 was associated with the CP resistance in LUAD. Furthermore, CTBP2 was shown to be significantly overexpressed in A549/DDP cells compared to parental cells. Previous study indicated that loss of CTBP expression activated P53 and then suppressed cell cycle progression and promoted cell apoptosis (16). CTBP2 was negatively regulated by ALDOB, which induced apoptosis in colon cancer and inhibited intrahepatic metastasis and lung metastases in hepatic cancer (17,18). Furthermore, Shi et al. also found that CTBP2 overexpression in esophageal cancer cells mitigated the decrease in cell viability and the prevention of cell apoptosis initiated by CP (12). On the contrary, CTBP2 suppression was observed to result in increased sensitivity of esophageal cancer cells to CP by decreasing cell viability and promoting CP-induced cell apoptosis induced by CP. In the present study, CTBP2 knockdown promoted A549/DDP cell sensitivity to CP. The A549/DDP-shCTBP2 cell proliferation was significantly inhibited at low concertation of CP compared with A549/DDP cells. Taken together, CTBP2 serves a crucial role in tumorigenesis and angiogenesis of tumors and is a primary factor driving CP resistance in LUAD.
ROS are molecular entities that exhibit chemical reactivity and are characterized by the presence of oxygen. The interaction of ROS interaction with proteins and cancer cell generation, survival, metastasis, and drug resistance have been reported (19,20). The basal ROS level in CP-resistant ovarian cancer cells is significantly higher than that observed in the corresponding parental cells. However, Li et al. demonstrated that CAMK2G inhibitor significantly sensitizes ovarian cancer cells to CP treatment by regulating ITPKB activity to modulate CP-induced high ROS (21). Zhong et al. reported that tripartite motif 17-mediated CP resistance was associated with attenuation of ROS production (22). In this study, the knockdown of CTBP2 did not increase intracellular ROS production. However, the ROS level was significantly increased by treating CP after the knockdown of CTBP2. The mitochondria constitute a significant origin of ROS, and the mitochondria that have been damaged by ROS produce a greater quantity of ROS. The generation of excessive ROS results in MMP loss, leading to mitochondrial pathway-dependent apoptosis (23). Our study showed that elevated ROS generation induced a decrease in MMP and triggered mitochondrial pathway-dependent apoptosis. Taken together, CTBP2 knockdown enhances CP-induced ROS production and restores CP sensitivity in CP-resistant LUAD cells.
Conclusions
The findings of the current investigation suggest that CTBP2 was overexpressed in LUAD tissues compared to non-cancerous tissues. Moreover, CTBP2 has also been observed to be overexpressed in LUAD cells that are resistant to CP compared to their parental counterparts. CTBP2 overexpression could serve as a specific prognostic biomarker for individuals diagnosed with LUAD, indicating a poorer prognosis. Moreover, CTBP2 knockdown enhances CP sensitivity in CP-resistant LUAD cells by inducing ROS production. This information could potentially offer a novel approach to mitigating chemoresistance to CP during the management of LUAD.
Acknowledgments
Funding: This research was funded by
Footnote
Reporting Checklist: The authors have completed the MDAR reporting checklist. Available at https://tcr.amegroups.com/article/view/10.21037/tcr-23-2135/rc
Data Sharing Statement: Available at https://tcr.amegroups.com/article/view/10.21037/tcr-23-2135/dss
Peer Review File: Available at https://tcr.amegroups.com/article/view/10.21037/tcr-23-2135/prf
Conflicts of Interest: All authors have completed the ICMJE uniform disclosure form (available at https://tcr.amegroups.com/article/view/10.21037/tcr-23-2135/coif). The authors have no conflicts of interest to declare.
Ethical Statement: The authors are accountable for all aspects of the work in ensuring that questions related to the accuracy or integrity of any part of the work are appropriately investigated and resolved. The study was conducted in accordance with the Declaration of Helsinki (as revised in 2013).
Open Access Statement: This is an Open Access article distributed in accordance with the Creative Commons Attribution-NonCommercial-NoDerivs 4.0 International License (CC BY-NC-ND 4.0), which permits the non-commercial replication and distribution of the article with the strict proviso that no changes or edits are made and the original work is properly cited (including links to both the formal publication through the relevant DOI and the license). See: https://creativecommons.org/licenses/by-nc-nd/4.0/.
References
- Khine HEE, Ecoy GAU, Roytrakul S, et al. Chemosensitizing activity of peptide from Lentinus squarrosulus (Mont.) on cisplatin-induced apoptosis in human lung cancer cells. Sci Rep 2021;11:4060. [Crossref] [PubMed]
- Qian J, LeSavage BL, Hubka KM, et al. Cancer-associated mesothelial cells promote ovarian cancer chemoresistance through paracrine osteopontin signaling. J Clin Invest 2021;131:e146186. [Crossref] [PubMed]
- Zhang P, Li B, Chen Q, et al. Glucose restriction induces ROS-AMPK-mediated CTR1 expression and increases cisplatin efficiency in NSCLC. Cancer Lett 2022;543:215793. [Crossref] [PubMed]
- Morledge-Hampton B, Kalyanaraman A, Wyrick JJ. Analysis of cytosine deamination events in excision repair sequencing reads reveals mechanisms of incision site selection in NER. Nucleic Acids Res 2024;52:1720-35. [Crossref] [PubMed]
- Chen X, Wang C, Zhou D, et al. Accumulation of advanced glycation end products promotes atrophic nonunion incidence in mice through a CtBP1/2-dependent mechanism. Exp Cell Res 2023;432:113765. [Crossref] [PubMed]
- Liu X, Zhu C, Li J, et al. HBV Upregulates CtBP2 Expression via the X Gene. Biomed Res Int 2018;2018:6960573. [Crossref] [PubMed]
- Li H, Zhou F, Cao X, et al. C-terminal binding protein 2 promotes high-glucose-triggered cell proliferation, angiogenesis and cellular adhesion of human retinal endothelial cell line. Int Ophthalmol 2022;42:2975-85. [Crossref] [PubMed]
- Ju Q, Jiang M, Huang W, et al. CtBP2 interacts with TGIF to promote the progression of esophageal squamous cell cancer through the Wnt/beta catenin pathway. Oncol Rep 2022;47:29. [Crossref] [PubMed]
- Chen L, Wang L, Qin J, et al. CtBP2 interacts with ZBTB18 to promote malignancy of glioblastoma. Life Sci 2020;262:118477. [Crossref] [PubMed]
- Singh V, Katiyar A, Malik P, et al. Identification of molecular biomarkers associated with non-small-cell lung carcinoma (NSCLC) using whole-exome sequencing. Cancer Biomark 2023; Epub ahead of print. [Crossref] [PubMed]
- Li B, Xiang Z, Xiong F, et al. C-terminal binding protein-2 is a prognostic marker for lung adenocarcinomas. Medicine (Baltimore) 2020;99:e20076. [Crossref] [PubMed]
- Shi H, Mao Y, Ju Q, et al. C-terminal binding protein 2 mediates cisplatin chemoresistance in esophageal cancer cells via the inhibition of apoptosis. Int J Oncol 2018;53:167-76. [Crossref] [PubMed]
- Hu C, Zhou A, Hu X, et al. LMNA Reduced Acquired Resistance to Erlotinib in NSCLC by Reversing the Epithelial-Mesenchymal Transition via the FGFR/MAPK/c-fos Signaling Pathway. Int J Mol Sci 2022;23:13237. [Crossref] [PubMed]
- Shen L, Xia M, Zhang Y, et al. Mitochondrial integration and ovarian cancer chemotherapy resistance. Exp Cell Res 2021;401:112549. [Crossref] [PubMed]
- Wang DP, Gu LL, Xue Q, et al. CtBP2 promotes proliferation and reduces drug sensitivity in non-small cell lung cancer via the Wnt/beta-catenin pathway. Neoplasma 2018;65:888-97. [Crossref] [PubMed]
- Birts CN, Banerjee A, Darley M, et al. p53 is regulated by aerobic glycolysis in cancer cells by the CtBP family of NADH-dependent transcriptional regulators. Sci Signal 2020;13:eaau9529. [Crossref] [PubMed]
- Lian J, Xia L, Chen Y, et al. Aldolase B impairs DNA mismatch repair and induces apoptosis in colon adenocarcinoma. Pathol Res Pract 2019;215:152597. [Crossref] [PubMed]
- Tan M, Pan Q, Wu Q, et al. Aldolase B attenuates clear cell renal cell carcinoma progression by inhibiting CtBP2. Front Med 2023;17:503-17. [Crossref] [PubMed]
- Sun T, Xu J, Chen T, et al. A self-amplified ROS-responsive chemodrug-inhibitor conjugate for multi-drug resistance tumor therapy. Biomater Sci 2022;10:997-1007. [Crossref] [PubMed]
- Bekeschus S. Acquired cancer tyrosine kinase inhibitor resistance: ROS as critical determinants. Signal Transduct Target Ther 2021;6:437. [Crossref] [PubMed]
- Li J, Zheng C, Wang M, et al. ROS-regulated phosphorylation of ITPKB by CAMK2G drives cisplatin resistance in ovarian cancer. Oncogene 2022;41:1114-28. [Crossref] [PubMed]
- Zhong T, Zhang J, Liu X, et al. TRIM17-mediated ubiquitination and degradation of RBM38 promotes cisplatin resistance in non-small cell lung cancer. Cell Oncol (Dordr) 2023;46:1493-507. [Crossref] [PubMed]
- Huang J, Wang J, Song G, et al. Antiproliferative Evaluation of Novel 4-Imidazolidinone Derivatives as Anticancer Agent Which Triggers ROS-Dependent Apoptosis in Colorectal Cancer Cell. Molecules 2022;27:8844. [Crossref] [PubMed]