Microbiome in gynecologic malignancies: a bibliometric analysis from 2012 to 2022
Introduction
Gynecologic malignancies pose a major threat to women’s quality of survival (1). Factors contributing to the onset of gynecologic cancers, both modifiable and non-modifiable, include genetic mutations, ethnic disparities, high-risk sexual behavior and fluctuating hormone levels, and the microbiome (2). The microbiome comprises of microorganisms, the genome, and their surroundings (3). Although microbial carcinogenesis is poorly understood, pathogens and dysbiosis appear to be key components. Pathogenic microbes play a carcinogenic role in chronic inflammation, cell proliferation, angiogenesis, genotoxicity, and epithelial barrier breakdown at the colonized site, contributing to carcinogenesis (4). Microbial dysbiosis in the local microenvironment can induce immune disorder and promote tumor growth (2).
The female reproductive tract (FRT), especially the lower reproductive tract (vagina and cervix), naturally harbors distinct microbial communities. The microenvironment, which primarily consists of Lactobacillus, is crucial for maintaining the homeostasis and health of the female reproductive system. Disrupted microbial signatures, on the other hand, have been discovered in some gynecological cancers. Specific microorganisms, such as Helicobacter pylori in gastric carcinoma and Streptococcus bovis in colorectal carcinoma, have been linked to carcinogenesis (5). Similarly, almost all cervical cancer patients have human papillomavirus (HPV) infection (6). Persistent infection of high-risk HPV (HR-HPV) is a prerequisite for the development of cervical cancer (7-9). Studies have indicated the involvement of certain bacterial species in HPV persistence and clearance (10,11). In addition, low-abundant microbial diversity has been verified to be present in many tissues of malignant state, such as the uterus, ovaries and fallopian tubes, which were previously considered to be sterile (12,13). The gut and vaginal microbiome share over 30% of commensal bacteria (14), and the gut-vaginal microbiome axis suggests possible crosstalk as a source of gynecological cancer (15). It can be concluded that the microbiome of the gut and FRT are associated with gynecological cancers, providing new possibilities for using the microbial signatures as preventive and therapeutic agents.
Bibliometric analysis is a systematic and objective method to measure scientific achievements in a certain field without prejudice of researchers. It quantitatively analyzes the scientific data extracted from the literature and presents it in the form of scientometric mapping. It combines performance analysis (examining the contributions of various research components) and science mapping (visually examining the relationships between each research component).
To our knowledge, this is the first one-stop overview of the microbiome in gynecologic malignancies from a bibliometric perspective. This review aims to assist young researchers in quickly identifying high-impact authors and papers, understanding current research directions and future trends, and generating new ideas for future research. We present this article in accordance with the PRIBA reporting checklist (available at https://tcr.amegroups.com/article/view/10.21037/tcr-23-1769/rc).
Methods
Terms related to bibliometric analysis
The bibliometric analysis serves two key functions: performance analysis and science mapping, implemented by bibliometric software dependently (16). Performance analysis helps to clarify the cumulative output of publications, countries, institutions, and journals during a defined period. Productivity and impact are evaluated using the number of publications and citations, respectively, through indicators like the average number of citation (ANC; referred to as citation/publication ratio), the annual global citation score (GCS), and the betweenness centrality. The GCS shows the peer recognition. The betweenness centrality indicates the potential to transform the research development in a particular field.
Science mapping displays the relationships between elements in two patterns: cooperative network and co-occurrence network. In a collaborative network, countries, institutions, or authors from topic-related papers are represented as nodes, and the cooperative relationships between them are represented as links. In this way, cooperative network analysis can reflect the main research force distribution, thus promoting scientific development. In this work, co-occurrence network refers to co-word network which uses author keywords as the analysis object. When two keywords appear in an article at the same time, it is called keyword co-occurrence. Each keyword is represented as a node in the network, and the correlation between keywords is represented as a line. The thickness of a line reflects the intensity of correlation, while node’s size reflects the frequency of keyword occurrence.
Clustering is the process of categorizing research elements (in this work, keywords) into groups (represented by different colors in the figure). Each cluster represents a distinct research topic, and the keywords within the same cluster are highly correlated. As a result, co-word analysis can uncover research directions and hotspots. Citation burst detection identifies nodes (in this work, keywords, and references) with citation spikes, indicating shifts in a field. In the citation burst detection network, the dark blue line represents the publication year of a node, while the red line represents the beginning and end of the burst cycle. The importance of the node is denoted by burst strength. Focusing on nodes with significant recent bursts can aid in forecasting future research frontiers. The reference analysis significantly compensates for the lack of publications in an emerging field.
Data search and screening
Data are the core to bibliometrics. Web of Science (WOS) is commonly utilized for its broad, regularly updated, and high-quality literature. It contains all bibliographic data except the main text. In this work, relevant data were retrieved from the Science Citation Index Expanded (SCI-EXPANDED) in WOS Core Collection (WoSCC) on January 12, 2023, with search terms on gynecologic malignancies and microbiome. The following search strategy was determined: topic search (TS) = (“gynecologi* malignant tumor*” OR “gynecologi* malignanc*” OR “cervical cancer*” OR “cervical carcino*” OR “uterine cervical neoplasm*” OR “endometrial carcinoma*” OR “endometrial cancer*” OR “ovarian cancer*” OR “ovarian carcinoma*” OR “vulvar cancer*” OR “vaginal cancer*” OR “fallopian tube cancer*”) AND TS = (dysbiosis NEAR/5 microbio* OR “vaginal microbio*” OR “gut microbio*” OR “cervical microbio*” OR “uterine microbio*” OR “endometrial microbio*” OR “cervicovaginal microbio*” OR “ovarian NEAR/5 oncobio*” OR “vulvar microbio*” OR “fallopian tube microbio*” OR “vaginal microenvironment*” OR “cervicovaginal microenvironment*” OR “cervical microenvironment*” OR “endometrial microenvironment*” OR “vaginal microeco*” OR probiotic* OR “commensal microbio*” OR host NEAR/5 microbio* OR “microbial composition”). The document type was refined into articles and review articles. The language was restricted to English. The time span in the retrieval process was set from 2012 to 2022. A raw dataset of 313 articles was obtained.
Data cleansing followed the exclusion criteria below: (I) literatures correlated to only one of the search terms; (II) papers from non-medical domains, such as studies on the design of probiotics or vaccine for cancer prevention; and (III) papers focusing on other microbiome-related cancers, e.g., breast cancer and colorectal cancer. In the process of exclusion, two writers independently conducted the literature review, and any disagreements were handled by a third party. After the manual exclusion, 204 articles were exported with full records and cited references in plain text format.
The flow chart of data collection and analysis is shown in Figure 1.
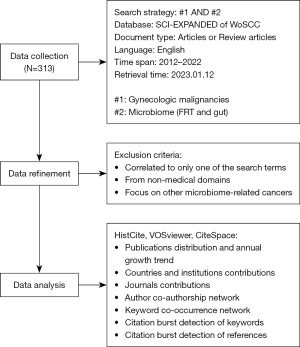
Data analysis and visualization
HistCite Pro 2.1 was used to extract basic information. As a result, 204 documents encompassed 1,383 authors affiliated with 456 institutions in 49 countries, and were published in 119 journals, citing 9,291 references.
VOSviewer 1.6.18 was used to synthesize science mappings including the co-occurrence network of keywords, and collaborative networks of countries, journals, and authors. Notably, a thesaurus file was applied to the process of keywords analysis. In addition, CiteSpace 6.1.R6 was utilized to assess the centrality of countries, references and keyword citation bursts.
Microsoft Office Excel 2019 (Microsoft, Redmond, Washington, DC, USA) was applied to generate statistical charts using the aforementioned data.
Results
Publications characteristics and annual growth trend
A total of 204 papers published between 2012 and 2022 were identified. Figure 2 displays the annual distribution of 204 papers, the annual GCS, and the trend line. It shows a steady growth trend of publications with a sharp increase in 2020, which implies a recent surge in interest in the microbiome’s role in gynecologic malignancies.
Typically, a higher GCS coupled with a smaller number of annual publications is indicative of breakthroughs in a specific field. In 2015 and 2016, the GCS/publication ratio reached remarkable heights.
Countries and institutions contributions
Figure 3 is a visualization mapping of descriptive information on countries and institutions. A total of 49 countries were extracted from 204 publications. Figure 3A shows the top 10 productive countries with their respective ANCs. The United States was the most prolific country accounting for almost one-third of the total output [64], followed by China [58], Italy [13], the United Kingdom [12], Canada [8], etc. The citation/publication ratio focuses on the quality of papers rather than the quantity. The United Kingdom (57.08), South Korea (27.5), and the United States (19.39) were ranked as the top three. China ranked second in the number of publications, but only ninth (5.09) in terms of citation/publication ratio. Figure 3C depicts countries with the centrality greater than 0.1, represented by a purple ring around nodes. The higher the centrality, the more likely it is to transform the direction of research. The United States (0.50) and the United Kingdom (0.16) were the only two countries that own the centrality, indicating that their publications have the potential to set research directions. Figure 3D presents the international collaborations by countries. The number of links reflect the number of cooperative partners, while the total link strength (TLS) and thickness reflect the cooperation strength. Strong economic strength and a solid academic foundation foster international cooperation. Similarly, the United States (link: 22; TLS: 36) and the United Kingdom (link: 14; TLS: 22) covers the majority of countries in the network, but communications between them are the most frequent and intense (the thickest line). Except for South Korea (link: 0), China is the least cooperative of the top ten productive countries, collaborating with only three countries: the United States, Norway, and Canada.
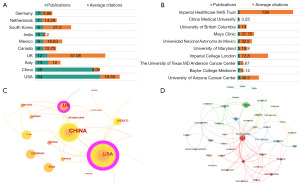
In general, institutional contribution corresponds to country distribution. Figure 3B illustrates the top 10 productive institutions, with University of Arizona Cancer Center ranked first (8). However, Imperial College London [72.8] and Imperial College Healthcare NHS Trust [139] showed impressive average citation rates. China Medical University is the only institution from China ranked in the top 10.
Journals contributions
In this work, there were 119 journals extracted from 204 papers. Table 1 displays a list of the top 10 prolific journals, with Frontiers in Cellular and Infection Microbiology published the most (15; ANC =2.07), while Scientific Reports had the highest average citations (8; ANC =41.88). There were six journals with impact factor (IF) greater than 5, including Frontiers in Cellular and Infection Microbiology (6.073), Frontiers in Oncology (5.738), Cancers (6.575), International Journal of Molecular Sciences (6.208), Gynecologic Oncology (5.304), and Frontiers in Microbiology (6.064). In total, 60% of the top 10 were classified as Q1 and the rest as Q2.
Table 1
Rank | Source journal | Number | Percentage (%) | IF 2022 | JCR category (quartile rank) | Citation counts | Average citation |
---|---|---|---|---|---|---|---|
1 | Frontiers in Cellular and Infection Microbiology | 15 | 5.1 | 6.073 | Microbiology (Q1); Immunology (Q2) | 31 | 2.07 |
2 | Frontiers in Oncology | 9 | 5.1 | 5.738 | Oncology (Q2) | 10 | 1.11 |
3 | Scientific Reports | 8 | 4.5 | 4.996 | Multidisciplinary Sciences (Q2) | 335 | 41.88 |
4 | PLoS One | 7 | 3.2 | 3.752 | Multidisciplinary Sciences (Q2) | 286 | 40.86 |
5 | Cancers | 7 | 2.5 | 6.575 | Oncology (Q1) | 27 | 3.86 |
6 | International Journal of Molecular Sciences | 6 | 2.5 | 6.208 | Biochemistry & Molecular Biology (Q1); Chemistry, Multidisciplinary (Q2) | 31 | 5.17 |
7 | Gynecologic Oncology | 4 | 1.9 | 5.304 | Obstetrics & Gynecology (Q1); Oncology (Q2) | 82 | 20.50 |
8 | Frontiers in Microbiology | 4 | 1.9 | 6.064 | Microbiology (Q1) | 33 | 8.25 |
9 | International Journal of Gynecological Cancer | 4 | 1.9 | 4.661 | Obstetrics & Gynecology (Q1); Oncology (Q2) | 26 | 6.50 |
10 | BMC Cancer | 4 | 1.9 | 4.638 | Oncology (Q2) | 22 | 5.50 |
IF, impact factor; JCR, journal citation reports.
Figure 4 presents the average citations of the top 15 high-producing journals in red to blue colors. As depicted in Figure 4, Bjog-an International Journal of Obstetrics and Gynecology has recently focused on the field of microbiome and gynecological cancers. Having only two reviews published, the ANC is as high as 63.50. One article from the journal reached a breakthrough conclusion that Lactobacillus spp., such as L. iners, cannot be considered protective as a whole. Besides, three high-IF reviews were published in Seminars in Cancer Biology in 2022 (4,17,18).
Co-authorship network
Figure 5 depicts a co-authorship network featuring authors who have published a minimum of three papers. Based on collaborative relationships, 37 authors were grouped into eight clusters (labelled from cluster 1 to cluster 8). Cluster 2 stood out as the largest cluster, containing 17 authors. Cluster 1 has the most output, including the top three most prolific authors, whereas cluster 3 has the highest ANC. The links within clusters aligns with the common practice of authors from the same institution collaborating on scholarly research. However, the eight clusters are independent of each other, suggesting that the majority of partnerships are intra-institutional.
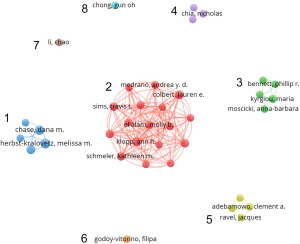
Table 2 was conducted from three perspectives: author name with their respective number of publications; most influential author with ANC; and research focus of each cluster. In accordance with Table 2, the top 5 most productive authors were Herbst-Kralovetz MM [8], Chase DM [7], Łaniewski P [6], Colbert LE [6], and Klopp AH [6]. The most influential author was Mitra A with 139 citations per document.
Table 2
Cluster | Author name [number of documents] | Most influential author [ANC] | Research focus of each cluster (reference number) |
---|---|---|---|
1 | Herbst-Kralovetz MM [8], Chase DM [7], Łaniewski P [6], Goulder A [3], Roe DJ [3] | Herbst-Kralovetz MM [46.5] | Cervicovaginal microenvironment features (e.g., genital inflammation, vaginal pH, and vaginal microbiota) (7); cancer biomarkers (10); metabolite (19); DNA virome (20); multi-omics integrated analysis & HPV-induced cervical carcinogenesis (21); high-quality reviews on microbiome in gynecologic malignancies (2,22); a review on uterine microbiota in uterine diseases (12) |
2 | Colbert LE [6], Klopp AH [6], Mezzari MP [5], Schmeler KM [5], Sims TT [5], Solley TN [5], Ramondetta L [4], Petrosino JF [4], El Alam MB [4], Jhingran A [4], Medrano AYD [4], Biegert GWG [3], Karpinets TV [3], Ahmed-Kaddar M [3], Lin LL [3], Wu XG [3], Yoshida-Court K [3] | Medrano AYD [10] | Gut microbiome differences in advanced CC patients versus healthy controls (23); cervical microbiota differences between cervical dysplasia and cervical cancer in Botswana (24); antibiotics affect CC progression in mice by microbial alteration of vagina rather than gut (25); gut microbiome & survival rates for CC patients receiving chemoradiation (26); gut microbiome changes & during and after pelvic CRT in gynecologic cancers (27); specific gut microbiome in rectal mucus layer may affect the initial tumor size and stage of CC (28) |
3 | Kyrgiou M [4], Mitra A [3], Macintyre DA [3], Bennett PR [3], Moscicki AB [3] | Mitra A [139] | Vaginal microbiome & HPV-related cervical carcinogenesis (11,18,29,30) |
4 | Walther-Antonio MRS [3], Chia N [3], Mariani A [3] | Mariani A [50.33] | Atopobium vaginae and P. somerae of uterine microbiome & endometrial cancer identification (13); postmenopausal status & ECbiome AND P. somerae as predictive biomarker of EC (31); P. somerae biology suggests its potential for intracellular invasion of EC cells (32) |
5 | Adebamowo CA [3], Adebamowo SN [3], Famooto A [3], Ravel J [3] | Ravel J [26] | Mycoplasma hominis & HPV persistence (33); a cohort study profile on HPV and CC with environmental, microbiomic, genetic and epigenetic factors considered (34); vaginal microbiota and persistent HR-HPV underlying HIV status (35) |
6 | Godoy-Vitorino F [3] | Godoy-Vitorino F [13.33] | Cervicovaginal fungi and bacteria & CIN and HR-HPV infections in Hispanic population (9); urinary metabolites paired with cervicovaginal microbiome as a detection of HPV infection & multi-omic integrated analysis (36); protocols for the multi-omic integration of cervical microbiota and urine metabolome (37) |
7 | Li C [3] | Li C [8.33] | Cervicovaginal microbiota changes after HPV16 clearance (38); endometrial microbiome & biomarker & hematological indicators (39); HR-HPV types related to specific species to SIL rather than abundance (40) |
8 | Chong GO [3] | Chong GO [4.33] | A diagnostic model with predicting utility & fetal microbiota & ICC (41); non-BV-associated bacterial communities & cervical carcinogenesis prediction (42); group B Streptococcus & gynecologic malignancies (43) |
ANC, average number of citation; HPV, human papillomavirus; CC, cervical cancer; CRT, chemoradiotherapy; ECbiome, EC microbiome; EC, endometrial cancer; HIV, human immunodeficiency virus; CIN, cervical intraepithelial neoplasia; HR-HPV, high-risk HPV; ICC, invasive cervical carcinoma; BV, bacterial vaginosis.
Keyword co-occurrence network and citation burst detection
A total of 42 out of 420 author keywords were extracted to analyze the co-occurrence network. Each keyword has a frequency of at least three occurrences. Topic-related keywords were grouped into three clusters (Figure 6A). Cluster Red referred to cervical cancer and the microbiome, which was mainly dominated by cervical cancer, HPV, vaginal microbiota, probiotics, cervical intraepithelial neoplasia (CIN), and Lactobacillus. Cluster green referred to endometrial cancer and the microbiome, which was mainly composed of microbiota, endometrial cancer, inflammation, dysbiosis, and estrobolome. Cluster blue referred to ovarian cancer and the microbiome, which mainly focused on ovarian cancer, gut microbiota, 16s ribosomal RNA (rRNA) sequencing, chemotherapy. “Cervical cancer”, “HPV” and “microbiota” were the most frequent keywords.
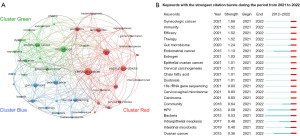
Figure 6B presents keywords with the strongest citation bursts during the period from 2021 to 2022, indicating the future research hotspots in microbiome-related research on gynecologic malignancies. “Endometrial cancer” and “ovarian cancer” have received the most attention in the last year. The importance of gut and cervicovaginal microbiota in gynecological cancers has gained significant discussion. The role of estrogen, short-chain fatty acids, and other molecular substances in the etiology of microbiome-induced gynecologic malignancies has gradually been recognized. Microbial treatment related to “therapy” and “efficacy” are also areas being addressed recently. The term “endometrial and ovarian microbiome” may arise soon.
Figure 7A lists the top 10 references that have strong citation bursts between 2021 and 2022, which provides a reliable way to track the latest research focus. Figure 7B presents the top 5 references with the strongest citation bursts during the period of 2020–2022, shedding light on research directions that have sustained considerable interest in recent years. The references were ordered by the strength of burst, all exceeding a threshold of 2.
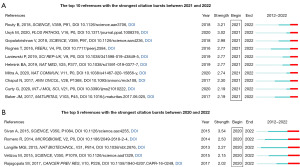
Discussion
Integrative Human Microbiome Project (iHMP) was initiated in 2013, completed in May 2019. It was proposed to examine the host-microbial interaction targeting illness. The microbiome of the gut and FRT is particularly significant based on the two major projects: “Pregnancy and preterm birth” and “Inflammatory bowel disease” (44). Gynecologic cancer and the microbiome are a relatively new field of research for academics, yet the growth rate, hot topics and research gaps remain unknown. Overall, a total of 204 papers published within a decade were identified.
General information
The total number of published papers may not be substantial, but it shows a promising growth trend. Within just 1 year, from 2019 to 2020, publications doubled, and the trendline predicts a growth of more than 100 by 2024. This suggests an increasing academic focus on the role of the microbiome in gynecological cancer. The GCS/publication ratio peaked remarkably in 2015 and 2016, which may be linked to the emergence of groundwork during this period.
A total of 49 countries have published relevant papers. The United States and the United Kingdom were the primary driving forces in this field, attributing to their prolificacy, high-quality output, and extensive cooperation. China, displaying remarkable productivity, ranked second within just 6 years, with 47 new publications in the last 2 years. Although China ranked ninth in terms of citation/publication ratio, the quality and publication year of the articles should be considered. China prefers intra-state cooperation over international collaboration, and would require enhanced global communication to elevate scientific research proficiency.
The University of Arizona Cancer Center, affiliated with the United States, secured the top position among the top ten most prolific institutions. This dominance could be attributed to the majority of members from the most active author’s group being affiliated with this institution. The most influential institutions are the National Institute of Technology and Imperial College Healthcare NHS Trust, both of which are situated in the United Kingdom. High-impact authors such as Mitra A and Kyrgiou M are from these two institutions, respectively. These findings align with national contributions.
Journal contribution analysis helps researchers find high-quality papers and select appropriate journals for submission. The most cited article across the entire dataset was the article “Cervical intraepithelial neoplasia (CIN) disease progression is associated with increased vaginal microbiome diversity” (11). Mitra et al. (11) suggested that a non-Lactobacillus dominated microbiome and high bacterial diversity [i.e., the community state type IV (CST-IV) community] are implicated in HPV persistence and worsening CIN disease severity, if exposure to invasive cervical carcinoma (ICC) is left untreated. This is the first cross-sectional study to relate vaginal microbiome diversity to CIN progression in women of reproductive age, laying the groundwork for future research. Nevertheless, the causal association between vaginal microbiome and HPV persistence is unknown. One of the high-IF articles published in Seminars in Cancer Biology were written by Kyrgiou et al. (18). The group investigated the interaction between vaginal microbiome and HPV-induced cervical diseases in depth. The highly diverse pro-inflammatory environment of the vagina may be a natural feature of CIN women that cannot be reconstructed by HPV clearance surgery. Local inflammation, on the other hand, prevents the restoration of Lactobacillus-rich communities, which has a promoting impact on the recurrence of precancerous lesions.
In general, author’s groups tend to collaborate on specific topics. Analyzing the characteristics of each cluster is helpful to understand the research direction in a certain field.
Cluster 4 is the only cluster that described uterine microbiota (particularly P. somerae) contributing to endometrial cancer (13,31,32). The remaining clusters investigated the role of the microbiome in HPV-mediated cervical neoplasia and cancer. The research topic, however, differed slightly. Cluster 1 mainly revealed the characteristics of the cervicovaginal microenvironment related to genital inflammation and cervical cancer, such as microbial taxa, metabolites and immune biomarkers (2,7,10,12,19-22). Cluster 2 examined the therapeutic potential of gut microbiome to cervical cancer (23-28). Cluster 3 reviewed the host-vaginal microbiome interaction (11,18,29,30). Cluster 5 explored the relationship between HPV persistence and Mycoplasma hominis and human immunodeficiency virus (HIV) viruses located in the vaginal microenvironment (33-35). Cluster 6 made an outstanding contribution by developing a non-invasive HPV infection prediction technique by combining urine metabolome data with the cervicovaginal microbiota (9,36,37). Cluster 7 illustrated that HR-HPV types are determined by vaginal microbial species rather than abundance (38-40). Cluster 8 focused on the ability of the fetal microbiota and non-bacterial vaginosis (BV)-associated vaginal bacteria to predict cervical cancer (41-43). New research perspectives are crucial for understanding the microbiome’s role in cervical carcinogenesis. Additionally, there is a need for expanded investigations into microbial connections with other gynecological cancers.
Research status
Keyword clustering highlights different research directions in a certain field. The larger the node, the more extensive the corresponding research content. In the realm of microbiome-related studies, “cervical cancer” emerges as the primary research focus. Research into “endometrial and ovarian cancer” is still at an early stage, and “vulvar and vaginal cancer” is poorly investigated with no corresponding nodes shown in the Figure 5.
Cervical cancer
Cervical cancer ranks fourth among female cancers worldwide. Persistent infection with HR-HPV is proved to be sufficient for the development of ICC (29). Modifications in microbes colonized in the cervicovaginal microenvironment may be a catalyst for persistent HPV infection by depleting Lactobacillus dominance like L. iners (rather than L. Crispatus and L. Asseri) and increasing CST-IV diversity (45). As a result, the local microenvironment’s symbiotic equilibrium is disrupted by the instability of microbiota. Microbial dysbiosis is considered to continuously modulate host defense responses in favor of HPV through altered immune and metabolic signaling resistance (2,18).
The sequence of bacterial flora changes and HPV infection may exist in chronological order during the progression of CIN. Mitra et al. reported in 2015 that HPV itself can induce a pro-inflammatory environment, adversely affect host immune defense and mucosal metabolism, and lead to abnormal vaginal microbiome (11). In 2021, Mitra et al. discovered that surgical resection of CIN lesions had no effect on vaginal microbiome composition or cytokine levels (46). Nevertheless, the vaginal microbiome alteration causes epithelial damage, apoptosis, inflammation, thereby promoting HPV colonization (47). More studies on microbiome of the lower FRT and cervical lesions are needed. It is also important to consider the impact of secondary bacterial colonization on subsequent HPV infection (48).
In the context of oncology treatment, the use of probiotics to promote HPV clearance is a treatment strategy. Probiotics towards Lactobacillus spp. have been proposed as an intervention for BV (49). An early study published in 2013 discovered that vaginal Lactobacillus spp. has an independent cytotoxic effect on cervical cells (50). Since then, Lactobacillus spp. has gradually gained attention for its potential as probiotics. Using protective species as probiotics may be a low-cost and promising therapy for cervical cancer.
Endometrial cancer
The term “uterine microbiome” has come into view since the consensus of a healthy uterus being sterile has been proven wrong. Emerging evidence suggests that the uterine microbiota may impact endometrial structure and function, thereby inducing inflammation or dysbiosis, potentially contributing to adverse birth outcomes and endometrial diseases (12,51).
The majority of studies have identified Lactobacillus spp. as the uterine microbiota. Although Lactobacillus dominance is a predictor of vaginal health, it may not be a predictor of uterine health (12). Lactobacillus spp. in the uterus is either the result of vaginal ascent or is a natural component of the uterine microenvironment (51,52). In one study, high vaginal pH is thought to be associated with endometrial cancer, which is age dependent and particularly observed in postmenopausal women (13). Interestingly, the rise in pH caused by vaginal Lactobacillus depletion increases microbial diversity independently (31). Cervical mucus plugs do not completely prevent vaginal bacteria movement, and the risk of sample contamination increases the likelihood of vaginal ascent, which could explain the concentration of Lactobacillus spp. found in many studies (12,53).
Deficiency of vaginal Lactobacillus has been linked to pelvic inflammation, a clear factor that accelerates the development of endometrial cancer (54). Moreno et al. discovered that a lower proportion of Lactobacillus spp. in vaginal samples influenced the production of pro-inflammatory cytokines in endometrial cells (55). As a result, vaginal Lactobacillus may disrupt the endometrium’s normal physiological processes by raising the pH of the local microenvironment or inducing inflammation, thus enhancing the risk of cancer. However, it is unclear how retrograde ascending Lactobacillus contributes to endometrial cancer development.
A 2016 study first strongly linked endometrial cancer with Atopobium and Porphyromonas species (when vaginal pH >4.5) (13). P. somerae was later confirmed as a potential biomarker for predicting early endometrial cancer, making it one of the field’s most notable achievements (31). As a consequence, exploring the mapping from vaginal microflora to the uterine microenvironment emerges as a compelling avenue for further investigation.
Ovarian cancer
Ovarian cancer, a formidable challenge in public health, necessitates a comprehensive understanding for effective prevention and early diagnosis (1). Examination of microbial signatures has revealed an array of overrepresented taxa in ovarian cancer tissue, including Chlamydia trachomatis, Mycoplasma, Brucella, Firmicute, Streptococcus, Aerococcus, Bacteroides, Proteobacteria, Acinetobacter, along with HPV and Cytomegalovirus (56-59).
Recent investigations have found an ovarian cancer microbiome characterized by the presence of Dialister, Corynebacterium, Prevotella, and Peptoniphilus (60). These microbes, more common in early-stage and low-grade ovarian cancer, hint at early detection potential in contrasting late-stage and high-grade cases.
The identification of microbial features indicates the presence of a non-Lactobacillus dominant microbiota in ovarian cancer (5). Further exploration is warranted.
Keyword citation burst
Keywords citation bursts sought to determine future research focus. The term “gut microbiome” is currently a highly popular topic in microbiome research, indicating a need for further innovative studies to address the current gaps in knowledge.
Extensive dysbiosis of the gut microbiota has been shown to induce changes in inflammation, metabolic disorders, and immune dysregulation, thereby altering disease progression and treatment outcomes (61,62). It is recognized that local disturbances in the microenvironment not only trigger sustained responses but also impact the responsiveness of other tissues (2). Numerous studies have focused on exploring the characteristics of the gut microbiota in gynecological cancer patients. The primary research methods are broadly applicable, involving the sequencing of fecal samples to identify differences in the structure and composition of the gut microbiota among cancer patients, benign lesions patients, and healthy controls. Additionally, mouse cancer models have been used to investigate the potential therapeutic effects of different interventions on the gut microbiota, such as fecal microbiota transplantation (FMT), dietary interventions, antibiotic treatments, and probiotic supplementation. Relevant studies have been prolific (63).
Recent investigations have indicated a potential correlation between the genera Prevotella, Lactobacillus, and Clostridium and the risk of early-stage cervical cancer, suggesting the gut microbiota as a predictive biomarker (41). Additionally, gut-derived circulating metabolites may trigger apoptosis in cervical cancer cell lines via immune pathways. Šarenac et al. explicated the protective effects of metabolites, such as ursodeoxycholic acid, on cervical cancer through three mechanisms: (I) activation of the c-Jun N-terminal kinase (JNK)/activator protein-1 (AP-1) signaling pathway; (II) regulation of the nuclear factor kappa-light-chain-enhancer of activated B cells (NF-κB); and (III) activation of pro-apoptotic genes, including Bax (64). The gut microbiota can also stimulate cervical cancer growth through Toll-like receptor (TLR)-mediated inflammatory responses (41). Besides, an animal experiment conducted by Wang and colleagues revealed that FMT from ovarian cancer patients accelerated tumor growth (65). The supplementation of Akkermansia in mice showed improved tumor control through upregulation of the T cell activation pathway and augmented secretion of interferon-γ (IFN-γ) by CD8+ T cells.
Pelvic chemoradiotherapy (CRT) is an essential component of gynecologic cancer treatment. Ongoing studies explore the gut microbiome’s role in immune tolerance and radiation resistance (5,66). El Alam et al. found sustained declines in gut microbiome richness and diversity during pelvic CRT for 58 women with cervical, vaginal, or vulvar cancer (27). Though alpha diversity returned to baseline after 12 weeks, the structure and composition remain altered. Sims et al. showed that high baseline gut diversity in cervical cancer patients correlated with increased CD4+ T cell infiltration post-CRT, enhancing therapeutic efficacy (26). Evidences suggest that higher gut diversity appears associated with reduced CRT-related issues in cervical cancer (66,67). FMT from healthy donors has successfully alleviated susceptibility to gastrointestinal toxicity (68). A recent study in “Cancer Research” revealed that antibiotics disturbed non-resistant gut microbiota in ovarian cancer mouse models (69). FMT from control-treated mice improved antibiotic-induced cisplatin resistance and overall survival, suggestive of gut-derived tumor-suppressive factors.
These results somewhat endorse the potential impact of gut-vaginal microbiome crosstalk on gynecological cancer progression, offering a therapeutic target. Immunoinflammatory mechanisms play a crucial role in these processes. Interesting findings from colorectal cancer metastasis research suggest that the gut microbiota and tumor cells jointly circulate and settle in metastatic sites. This phenomenon may warrant validation in ovarian cancer (70).
The proposal of the estrogen-gut microbiome axis provides another piece of evidence supporting this “cross-talk”, as it may serve as an indirect mechanism for inducing endometrial cancer development (15). Gut microbiota that metabolizes estrogen (e.g., Escherichia coli, Bacteroides fragilis, and Streptococcus agalactiae) secrete β-glucuronidase, enabling the deconjugation of estrogen and its reabsorption into the bloodstream (71,72). This triggers subsequent physiological reactions and pathological changes in the endometrium.
The innate immune responses and adaptability of the microbiota within the FRT are also gradually receiving attention. The native microbiota perceived by epithelial cells of the FRT through pattern recognition receptors promote host tolerance (73). It forms a defensive ecological barrier by secreting antimicrobial peptides and mucins (74). Estrogen and progesterone trigger the production of pro-inflammatory cytokines (e.g., IL-6 and IL-8) and antimicrobial peptides, regulating the immune response in vaginal epithelial cells (75). Vaginal homeostasis disruption results in Lactobacillus dominance loss, leading high microbial diversity, and local mucosal immune dysregulation. This disruption may contribute to carcinogenesis through sustained pro-inflammatory cytokine secretion, barrier disruption, and immune cell recruitment (76).
Research frontiers
Insight into the research frontiers was found by a detection of the references with significant citation burstness. Based on a total of 15 articles in Figure 7, two research hotspots were found worth discussing.
Gut microbiome & immune checkpoint inhibitors
Immunotherapy holds significant promise as a treatment approach for advanced or recurrent gynecological cancer. The establishment of the body’s autoimmune tolerance relies on the suppression of T cells at immune checkpoints. Checkpoint inhibitors, by reactivating T cells through modulation of checkpoint molecules on the surface of T cells or cancer cells, enable T cells to kill cancer cells and hinder cancer immune evasion (77).
In certain malignant tumors, the gut microbiota has been reported to boost therapeutic efficacy by inhibiting checkpoint molecules, with particularly notable findings in melanoma (78-80). An animal experiment conducted by Sivan et al. revealed that symbiotic gut microbiota was one of the factors influencing spontaneous anti-tumor immunity, with Bifidobacterium identified as a key marker of immune response (81). When melanoma mice received specific anti-programmed cell death protein 1 ligand 1 (PD-L1) therapy alone, those enriched in Bifidobacterium showed improved tumor control. This benefit could be eliminated by oral administration of Bifidobacterium or fecal transfer from a Bifidobacterium-rich to Bifidobacterium-poor one. Notably, mice that were previously unresponsive to immunotherapy showed a significant efficacy improvement. Some gut microbiotas have been found to alter host response to treatment, such as Akkermansia muciniphila (78), Bifidobacterium longum (81), Clostridiales, and Ruminococcaceae (79). Conversely, the study suggested that PD-1 non-responsive patients showed a higher relative abundance of Bacteroidales species that are implicated in the immunostimulatory effects of cytotoxic T lymphocyte-associated antigen-4 (CTLA-4) blockade (82). Additionally, Faecalibacterium was explored for its potential association with positive clinical responses to CTLA-4 inhibitors like ipilimumab (83). Besides, antibiotic therapy is thought to eliminate the immunotherapy benefit by reducing intestinal bacterial heterogeneity (78). These findings raise the possibility of investigating the role of the gut microbiota as an adjunct to immunotherapy in gynecologic malignancies.
Checkpoint inhibitors have gained approval for the treatment of recurrent endometrial cancer that is either mismatch repair protein-deficient or has a high microsatellite stability index (MSI-H), ovarian cancer, and advanced cervical cancer (84). For example, pembrolizumab was used to treat cervical cancer after the KEYNOTE-158 clinical trial (85).
Sequencing technology
The advancement of sequencing technology has shifted our understanding of the human microbiome and diseases from a macro to a micro level. Next-generation sequencing (NGS) technology offers new insights into early diagnosis, progression prediction, and treatment guidance of cancer.
Amplicon sequencing is the most commonly used method. It demands minimal sequence data, making it efficient and economical, although it has limited representativeness (86,87). Other methods, such as shotgun metagenomics and RNA sequencing, provide more accurate microbial classification at the genus and species levels but come with a higher cost (88).
The clinical application of microbiota-targeted therapy relies on precise gene sequencing for the accurate identification and regulation of specific microbial species. Clinical trials are underway to assess the potential of probiotics, fetal, and vaginal microbiota transplantation (VMT) treatments (4). For instance, a trial in Israel (NCT04517487) is recruiting participants to evaluate the feasibility of VMT in women with intractable BV.
Multi-omics analyses, combining microbiome and metabolome studies, contribute to a better understanding of disease pathology and metabolic pathways. They also help in the discovery of new biomarkers, providing further support for clinical diagnostics.
Conclusions
In this work, we systematically present general information, research status, and frontiers in the microbiome of gynecologic malignancies, offering a comprehensive overview. The relationship between cervical cancer and the microbiome is particularly intimate, while research on endometrial and ovarian cancers is still in its infancy. Areas such as vulvar and vaginal cancers require more attention. Existing research predominantly focuses on pathogenic diagnosis and microbial identification, with frontiers mainly exploring the impact of microbiota on treatment efficacy. Additionally, it is crucial to recognize the limitations of a microbe-centric approach, considering the host’s influence on microbial habitats and the impact of host environmental changes on microbial communities. Researchers may consider multidisciplinary integration to expand the scope of work in this field. Despite inevitable limitations, such as exclusion-induced incompleteness and biased term selection, we hope this review inspires researchers to expand both the scope and depth of their investigations.
Acknowledgments
Funding: This research was supported by
Footnote
Reporting Checklist: The authors have completed the PRIBA reporting checklist. Available at https://tcr.amegroups.com/article/view/10.21037/tcr-23-1769/rc
Peer Review File: Available at https://tcr.amegroups.com/article/view/10.21037/tcr-23-1769/prf
Conflicts of Interest: All authors have completed the ICMJE uniform disclosure form (available at https://tcr.amegroups.com/article/view/10.21037/tcr-23-1769/coif). The authors have no conflicts of interest to declare.
Ethical Statement: The authors are accountable for all aspects of the work in ensuring that questions related to the accuracy or integrity of any part of the work are appropriately investigated and resolved.
Open Access Statement: This is an Open Access article distributed in accordance with the Creative Commons Attribution-NonCommercial-NoDerivs 4.0 International License (CC BY-NC-ND 4.0), which permits the non-commercial replication and distribution of the article with the strict proviso that no changes or edits are made and the original work is properly cited (including links to both the formal publication through the relevant DOI and the license). See: https://creativecommons.org/licenses/by-nc-nd/4.0/.
References
- Siegel RL, Miller KD, Wagle NS, et al. Cancer statistics, 2023. CA Cancer J Clin 2023;73:17-48. [Crossref] [PubMed]
- Łaniewski P, Ilhan ZE, Herbst-Kralovetz MM. The microbiome and gynaecological cancer development, prevention and therapy. Nat Rev Urol 2020;17:232-50. [Crossref] [PubMed]
- Marchesi JR, Ravel J. The vocabulary of microbiome research: a proposal. Microbiome 2015;3:31. [Crossref] [PubMed]
- Wahid M, Dar SA, Jawed A, et al. Microbes in gynecologic cancers: Causes or consequences and therapeutic potential. Semin Cancer Biol 2022;86:1179-89. [Crossref] [PubMed]
- Chalif J, Wang H, Spakowicz D, et al. The microbiome and gynecologic cancer: cellular mechanisms and clinical applications. Int J Gynecol Cancer 2023;ijgc-2023-004894. [PubMed]
- Walboomers JM, Jacobs MV, Manos MM, et al. Human papillomavirus is a necessary cause of invasive cervical cancer worldwide. J Pathol 1999;189:12-9. [Crossref] [PubMed]
- Łaniewski P, Barnes D, Goulder A, et al. Linking cervicovaginal immune signatures, HPV and microbiota composition in cervical carcinogenesis in non-Hispanic and Hispanic women. Sci Rep 2018;8:7593. [Crossref] [PubMed]
- Brusselaers N, Shrestha S, van de Wijgert J, et al. Vaginal dysbiosis and the risk of human papillomavirus and cervical cancer: systematic review and meta-analysis. Am J Obstet Gynecol 2019;221:9-18.e8. [Crossref] [PubMed]
- Godoy-Vitorino F, Romaguera J, Zhao C, et al. Cervicovaginal Fungi and Bacteria Associated With Cervical Intraepithelial Neoplasia and High-Risk Human Papillomavirus Infections in a Hispanic Population. Front Microbiol 2018;9:2533. [Crossref] [PubMed]
- Łaniewski P, Cui H, Roe DJ, et al. Features of the cervicovaginal microenvironment drive cancer biomarker signatures in patients across cervical carcinogenesis. Sci Rep 2019;9:7333. [Crossref] [PubMed]
- Mitra A, MacIntyre DA, Lee YS, et al. Cervical intraepithelial neoplasia disease progression is associated with increased vaginal microbiome diversity. Sci Rep 2015;5:16865. [Crossref] [PubMed]
- Baker JM, Chase DM, Herbst-Kralovetz MM. Uterine Microbiota: Residents, Tourists, or Invaders? Front Immunol 2018;9:208. [Crossref] [PubMed]
- Walther-António MR, Chen J, Multinu F, et al. Potential contribution of the uterine microbiome in the development of endometrial cancer. Genome Med 2016;8:122. [Crossref] [PubMed]
- Amabebe E, Anumba DOC. Female Gut and Genital Tract Microbiota-Induced Crosstalk and Differential Effects of Short-Chain Fatty Acids on Immune Sequelae. Front Immunol 2020;11:2184. [Crossref] [PubMed]
- Baker JM, Al-Nakkash L, Herbst-Kralovetz MM. Estrogen-gut microbiome axis: Physiological and clinical implications. Maturitas 2017;103:45-53. [Crossref] [PubMed]
- Donthu N, Kumar S, Mukherjee D, et al. How to conduct a bibliometric analysis: An overview and guidelines. J Bus Res 2021;133:285-96. [Crossref]
- Haque S, Raina R, Afroze N, et al. Microbial dysbiosis and epigenetics modulation in cancer development - A chemopreventive approach. Semin Cancer Biol 2022;86:666-81. [Crossref] [PubMed]
- Kyrgiou M, Moscicki AB. Vaginal microbiome and cervical cancer. Semin Cancer Biol 2022;86:189-98. [Crossref] [PubMed]
- Ilhan ZE, Łaniewski P, Thomas N, et al. Deciphering the complex interplay between microbiota, HPV, inflammation and cancer through cervicovaginal metabolic profiling. EBioMedicine 2019;44:675-90. [Crossref] [PubMed]
- Kaelin EA, Skidmore PT, Łaniewski P, et al. Cervicovaginal DNA Virome Alterations Are Associated with Genital Inflammation and Microbiota Composition. mSystems 2022;7:e0006422. [Crossref] [PubMed]
- Bokulich NA, Łaniewski P, Adamov A, et al. Multi-omics data integration reveals metabolome as the top predictor of the cervicovaginal microenvironment. PLoS Comput Biol 2022;18:e1009876. [Crossref] [PubMed]
- Chase D, Goulder A, Zenhausern F, et al. The vaginal and gastrointestinal microbiomes in gynecologic cancers: a review of applications in etiology, symptoms and treatment. Gynecol Oncol 2015;138:190-200. [Crossref] [PubMed]
- Sims TT, Colbert LE, Zheng J, et al. Gut microbial diversity and genus-level differences identified in cervical cancer patients versus healthy controls. Gynecol Oncol 2019;155:237-44. [Crossref] [PubMed]
- Sims TT, Biegert GWG, Ramogola-Masire D, et al. Tumor microbial diversity and compositional differences among women in Botswana with high-grade cervical dysplasia and cervical cancer. Int J Gynecol Cancer 2020;30:1151-6. [Crossref] [PubMed]
- Karpinets TV, Solley TN, Mikkelson MD, et al. Effect of Antibiotics on Gut and Vaginal Microbiomes Associated with Cervical Cancer Development in Mice. Cancer Prev Res (Phila) 2020;13:997-1006. [Crossref] [PubMed]
- Sims TT, El Alam MB, Karpinets TV, et al. Gut microbiome diversity is an independent predictor of survival in cervical cancer patients receiving chemoradiation. Commun Biol 2021;4:237. [Crossref] [PubMed]
- El Alam MB, Sims TT, Kouzy R, et al. A prospective study of the adaptive changes in the gut microbiome during standard-of-care chemoradiotherapy for gynecologic cancers. PLoS One 2021;16:e0247905. [Crossref] [PubMed]
- Karpinets TV, Wu X, Solley T, et al. Metagenomes of rectal swabs in larger, advanced stage cervical cancers have enhanced mucus degrading functionalities and distinct taxonomic structure. BMC Cancer 2022;22:945. [Crossref] [PubMed]
- Mitra A, MacIntyre DA, Marchesi JR, et al. The vaginal microbiota, human papillomavirus infection and cervical intraepithelial neoplasia: what do we know and where are we going next? Microbiome 2016;4:58. [Crossref] [PubMed]
- Kyrgiou M, Mitra A, Moscicki AB. Does the vaginal microbiota play a role in the development of cervical cancer? Transl Res 2017;179:168-82. [Crossref] [PubMed]
- Walsh DM, Hokenstad AN, Chen J, et al. Postmenopause as a key factor in the composition of the Endometrial Cancer Microbiome (ECbiome). Sci Rep 2019;9:19213. [Crossref] [PubMed]
- Crooks TA, Madison JD, Walsh DM, et al. Porphyromonas somerae Invasion of Endometrial Cancer Cells. Front Microbiol 2021;12:674835. [Crossref] [PubMed]
- Adebamowo SN, Ma B, Zella D, et al. Mycoplasma hominis and Mycoplasma genitalium in the Vaginal Microbiota and Persistent High-Risk Human Papillomavirus Infection. Front Public Health 2017;5:140. [Crossref] [PubMed]
- Adebamowo SN, Dareng EO, Famooto AO, et al. Cohort Profile: African Collaborative Center for Microbiome and Genomics Research's (ACCME's) Human Papillomavirus (HPV) and Cervical Cancer Study. Int J Epidemiol 2017;46:1745-1745j. [Crossref] [PubMed]
- Dareng EO, Ma B, Adebamowo SN, et al. Vaginal microbiota diversity and paucity of Lactobacillus species are associated with persistent hrHPV infection in HIV negative but not in HIV positive women. Sci Rep 2020;10:19095. [Crossref] [PubMed]
- Chorna N, Romaguera J, Godoy-Vitorino F. Cervicovaginal Microbiome and Urine Metabolome Paired Analysis Reveals Niche Partitioning of the Microbiota in Patients with Human Papilloma Virus Infections. Metabolites 2020;10:36. [Crossref] [PubMed]
- Chorna N, Godoy-Vitorino F. A Protocol for the Multi-Omic Integration of Cervical Microbiota and Urine Metabolomics to Understand Human Papillomavirus (HPV)-Driven Dysbiosis. Biomedicines 2020;8:81. [Crossref] [PubMed]
- Li C, Zhang Z, Yang Y, et al. Changes in the cervicovaginal microbiota composition of HPV16-infected patients after clinical treatment. Cancer Med 2022;11:5037-49. [Crossref] [PubMed]
- Li C, Gu Y, He Q, et al. Integrated Analysis of Microbiome and Transcriptome Data Reveals the Interplay Between Commensal Bacteria and Fibrin Degradation in Endometrial Cancer. Front Cell Infect Microbiol 2021;11:748558. [Crossref] [PubMed]
- Huang X, Li C, Li F, et al. Cervicovaginal microbiota composition correlates with the acquisition of high-risk human papillomavirus types. Int J Cancer 2018;143:621-34. [Crossref] [PubMed]
- Kang GU, Jung DR, Lee YH, et al. Dynamics of Fecal Microbiota with and without Invasive Cervical Cancer and Its Application in Early Diagnosis. Cancers (Basel) 2020;12:3800. [Crossref] [PubMed]
- Kang GU, Jung DR, Lee YH, et al. Potential Association between Vaginal Microbiota and Cervical Carcinogenesis in Korean Women: A Cohort Study. Microorganisms 2021;9:294. [Crossref] [PubMed]
- Choi Y, Han HS, Chong GO, et al. Updates on Group B Streptococcus Infection in the Field of Obstetrics and Gynecology. Microorganisms 2022;10:2398. [Crossref] [PubMed]
- The Integrative Human Microbiome Project. Nature 2019;569:641-8. [Crossref] [PubMed]
- Norenhag J, Du J, Olovsson M, et al. The vaginal microbiota, human papillomavirus and cervical dysplasia: a systematic review and network meta-analysis. BJOG 2020;127:171-80. [Crossref] [PubMed]
- Mitra A, MacIntyre DA, Paraskevaidi M, et al. The vaginal microbiota and innate immunity after local excisional treatment for cervical intraepithelial neoplasia. Genome Med 2021;13:176. [Crossref] [PubMed]
- Zevin AS, Xie IY, Birse K, et al. Microbiome Composition and Function Drives Wound-Healing Impairment in the Female Genital Tract. PLoS Pathog 2016;12:e1005889. [Crossref] [PubMed]
- Muzny CA, Łaniewski P, Schwebke JR, et al. Host-vaginal microbiota interactions in the pathogenesis of bacterial vaginosis. Curr Opin Infect Dis 2020;33:59-65. [Crossref] [PubMed]
- Vujic G, Jajac Knez A, Despot Stefanovic V, et al. Efficacy of orally applied probiotic capsules for bacterial vaginosis and other vaginal infections: a double-blind, randomized, placebo-controlled study. Eur J Obstet Gynecol Reprod Biol 2013;168:75-9. [Crossref] [PubMed]
- Motevaseli E, Shirzad M, Akrami SM, et al. Normal and tumour cervical cells respond differently to vaginal lactobacilli, independent of pH and lactate. J Med Microbiol 2013;62:1065-72. [Crossref] [PubMed]
- Winters AD, Romero R, Gervasi MT, et al. Does the endometrial cavity have a molecular microbial signature? Sci Rep 2019;9:9905. [Crossref] [PubMed]
- Tao X, Franasiak J M, Zhan Y, et al. Characterizing the endometrial microbiome by analyzing the ultra-low bacteria from embryo transfer catheter tips in IVF cycles: Next generation sequencing (NGS) analysis of the 16S ribosomal gene. Hum Microbiome J 2017;3:15-21. [Crossref]
- Alizadehmohajer N, Shojaeifar S, Nedaeinia R, et al. Association between the microbiota and women’s cancers–Cause or consequences? Biomed Pharmacother 2020;127:110203. [Crossref] [PubMed]
- Martin DH, Marrazzo JM. The Vaginal Microbiome: Current Understanding and Future Directions. J Infect Dis 2016;214:S36-41. [Crossref] [PubMed]
- Moreno I, Garcia-Grau I, Perez-Villaroya D, et al. Endometrial microbiota composition is associated with reproductive outcome in infertile patients. Microbiome 2022;10:1. [Crossref] [PubMed]
- Zhou B, Sun C, Huang J, et al. The biodiversity Composition of Microbiome in Ovarian Carcinoma Patients. Sci Rep 2019;9:1691. [Crossref] [PubMed]
- Nené NR, Reisel D, Leimbach A, et al. Association between the cervicovaginal microbiome, BRCA1 mutation status, and risk of ovarian cancer: a case-control study. Lancet Oncol 2019;20:1171-82. [Crossref] [PubMed]
- Banerjee S, Tian T, Wei Z, et al. The ovarian cancer oncobiome. Oncotarget 2017;8:36225-45. [Crossref] [PubMed]
- Shanmughapriya S, Senthilkumar G, Vinodhini K, et al. Viral and bacterial aetiologies of epithelial ovarian cancer. Eur J Clin Microbiol Infect Dis 2012;31:2311-7. [Crossref] [PubMed]
- Asangba AE, Chen J, Goergen KM, et al. Diagnostic and prognostic potential of the microbiome in ovarian cancer treatment response. Sci Rep 2023;13:730. [Crossref] [PubMed]
- Garrett WS. Cancer and the microbiota. Science 2015;348:80-6. [Crossref] [PubMed]
- Li J, Ji Y, Chen N, et al. Colitis-associated carcinogenesis: crosstalk between tumors, immune cells and gut microbiota. Cell Biosci 2023;13:194. [Crossref] [PubMed]
- Di Tucci C, De Vito I, Muzii L. Immune-Onco-Microbiome: A New Revolution for Gynecological Cancers. Biomedicines 2023;11:782. [Crossref] [PubMed]
- Šarenac T, Mikov M. Cervical Cancer, Different Treatments and Importance of Bile Acids as Therapeutic Agents in This Disease. Front Pharmacol 2019;10:484. [Crossref] [PubMed]
- Wang Z, Qin X, Hu D, et al. Akkermansia supplementation reverses the tumor-promoting effect of the fecal microbiota transplantation in ovarian cancer. Cell Rep 2022;41:111890. [Crossref] [PubMed]
- Mitra A, Grossman Biegert GW, Delgado AY, et al. Microbial Diversity and Composition Is Associated with Patient-Reported Toxicity during Chemoradiation Therapy for Cervical Cancer. Int J Radiat Oncol Biol Phys 2020;107:163-71. [Crossref] [PubMed]
- Poonacha KNT, Villa TG, Notario V. The Interplay among Radiation Therapy, Antibiotics and the Microbiota: Impact on Cancer Treatment Outcomes. Antibiotics (Basel) 2022;11:331. [Crossref] [PubMed]
- Chang CW, Lee HC, Li LH, et al. Fecal Microbiota Transplantation Prevents Intestinal Injury, Upregulation of Toll-Like Receptors, and 5-Fluorouracil/Oxaliplatin-Induced Toxicity in Colorectal Cancer. Int J Mol Sci 2020;21:386. [Crossref] [PubMed]
- Chambers LM, Esakov Rhoades EL, Bharti R, et al. Disruption of the Gut Microbiota Confers Cisplatin Resistance in Epithelial Ovarian Cancer. Cancer Res 2022;82:4654-69. [Crossref] [PubMed]
- Li Q. Bacterial infection and microbiota in carcinogenesis and tumor development. Front Cell Infect Microbiol 2023;13:1294082. [Crossref] [PubMed]
- Sobstyl M, Brecht P, Sobstyl A, et al. The Role of Microbiota in the Immunopathogenesis of Endometrial Cancer. Int J Mol Sci 2022;23:5756. [Crossref] [PubMed]
- Flores R, Shi J, Fuhrman B, et al. Fecal microbial determinants of fecal and systemic estrogens and estrogen metabolites: a cross-sectional study. J Transl Med 2012;10:253. [Crossref] [PubMed]
- Aldunate M, Srbinovski D, Hearps AC, et al. Antimicrobial and immune modulatory effects of lactic acid and short chain fatty acids produced by vaginal microbiota associated with eubiosis and bacterial vaginosis. Front Physiol 2015;6:164. [Crossref] [PubMed]
- Gholiof M, Adamson-De Luca E, Wessels JM. The female reproductive tract microbiotas, inflammation, and gynecological conditions. Front Reprod Health 2022;4:963752. [Crossref] [PubMed]
- Wira CR, Fahey JV, Rodriguez-Garcia M, et al. Regulation of mucosal immunity in the female reproductive tract: the role of sex hormones in immune protection against sexually transmitted pathogens. Am J Reprod Immunol 2014;72:236-58. [Crossref] [PubMed]
- Moncla BJ, Chappell CA, Mahal LK, et al. Impact of bacterial vaginosis, as assessed by nugent criteria and hormonal status on glycosidases and lectin binding in cervicovaginal lavage samples. PLoS One 2015;10:e0127091. [Crossref] [PubMed]
- Pardoll DM. The blockade of immune checkpoints in cancer immunotherapy. Nat Rev Cancer 2012;12:252-64. [Crossref] [PubMed]
- Routy B, Le Chatelier E, Derosa L, et al. Gut microbiome influences efficacy of PD-1-based immunotherapy against epithelial tumors. Science 2018;359:91-7. [Crossref] [PubMed]
- Gopalakrishnan V, Spencer CN, Nezi L, et al. Gut microbiome modulates response to anti-PD-1 immunotherapy in melanoma patients. Science 2018;359:97-103. [Crossref] [PubMed]
- Baruch EN, Youngster I, Ben-Betzalel G, et al. Fecal microbiota transplant promotes response in immunotherapy-refractory melanoma patients. Science 2021;371:602-9. [Crossref] [PubMed]
- Sivan A, Corrales L, Hubert N, et al. Commensal Bifidobacterium promotes antitumor immunity and facilitates anti-PD-L1 efficacy. Science 2015;350:1084-9. [Crossref] [PubMed]
- Vétizou M, Pitt JM, Daillère R, et al. Anticancer immunotherapy by CTLA-4 blockade relies on the gut microbiota. Science 2015;350:1079-84. [Crossref] [PubMed]
- Chaput N, Lepage P, Coutzac C, et al. Baseline gut microbiota predicts clinical response and colitis in metastatic melanoma patients treated with ipilimumab. Ann Oncol 2017;28:1368-79. [Crossref] [PubMed]
- Pakish JB, Jazaeri AA. Immunotherapy in Gynecologic Cancers: Are We There Yet? Curr Treat Options Oncol 2017;18:59. [Crossref] [PubMed]
- Chung HC, Ros W, Delord JP, et al. Efficacy and Safety of Pembrolizumab in Previously Treated Advanced Cervical Cancer: Results From the Phase II KEYNOTE-158 Study. J Clin Oncol 2019;37:1470-8. [Crossref] [PubMed]
- Wensel CR, Pluznick JL, Salzberg SL, et al. Next-generation sequencing: insights to advance clinical investigations of the microbiome. J Clin Invest 2022;132:e154944. [Crossref] [PubMed]
- Rognes T, Flouri T, Nichols B, et al. VSEARCH: a versatile open source tool for metagenomics. PeerJ 2016;4:e2584. [Crossref] [PubMed]
- Jovel J, Patterson J, Wang W, et al. Characterization of the Gut Microbiome Using 16S or Shotgun Metagenomics. Front Microbiol 2016;7:459. [Crossref] [PubMed]