Based on network pharmacology and experimental validation, berberine can inhibit the progression of gastric cancer by modulating oxidative stress
Highlight box
Key findings
• Berberine can effectively inhibit the progression of gastric cancer (GC) through multiple targets and pathways, particularly by modulating oxidative stress and suppressing the activation of relevant signaling pathways such as hypoxia-inducible factor-1 (HIF-1), nuclear factor erythroid 2-related factor 2 (Nrf2)/heme oxygenase-1 (HO-1), and epithelial-mesenchymal transition (EMT) signaling pathways to exert its anti-GC pharmacological effects.
What is known and what is new?
• Oxidative stress levels are elevated in GC patients, promoting tumor progression. Berberine has been utilized in the treatment of bacterial infections, diabetes, cardiovascular diseases, and cancer. It exhibits potent anti-GC activity without significant adverse effects on the human body.
• Berberine can regulate the oxidative stress levels in GC cells, and its mechanism is associated with the downregulation of the activation of the Nrf2/HO-1 and HIF-1α oxidative stress-related signaling pathways in GC cells. Furthermore, it further inhibits the activation of the EMT signaling pathway to suppress tumor metastasis.
What is the implication, and what should change now?
• This study offers a new perspective for adjuvant therapy in GC, potentially reducing the side effects of chemotherapy, alleviating patient suffering and economic burden, and extending patient survival. It also provides a theoretical basis for the use of berberine as an adjuvant therapy for GC. Further in-depth research on the mechanisms of berberine’s anti-GC effects and clinical trials is needed to actively develop its clinical application.
Introduction
Gastric cancer (GC) is one of the most common malignant tumors globally, ranking as the third leading cause of cancer-related deaths and the fifth most diagnosed malignant tumor worldwide (1). GC often presents insidiously, with atypical early symptoms, leading to late-stage diagnosis in most patients. Treatment modalities for GC include chemotherapy, radiotherapy, surgery, immunotherapy, and targeted therapy (2). However, the median survival period is less than 1 year (3), with a very low 5-year survival rate of only 20% to 25% (4). Therefore, a thorough investigation into the mechanisms of GC development and progression, as well as the development of new and effective drugs and treatment strategies, is an urgent issue.
The oxidative stress levels in GC patients are elevated, promoting the progression of the disease (5). The primary characteristic of oxidative stress is the excessive generation of reactive oxygen species (ROS), which, when accumulated persistently, can lead to genetic toxicity, induce DNA damage, ultimately triggering genomic mutations and tumor formation (6-10). Additionally, due to its cytotoxic nature, ROS can induce apoptosis in tumor cells (11). Studies have revealed that hypoxia in GC cells can activate hypoxia-inducible factor-1 alpha (HIF-1α), which, in turn, reduces the expression of E-cadherin, an epithelial-mesenchymal transition (EMT)-related protein, thereby promoting tumor cell migration (12). The Kelch-like epichlorohydrin (ECH)-associated protein 1 (Keap1)-nuclear factor erythroid 2-related factor 2 (Nrf2) signaling pathway is the most studied cellular defense mechanism against oxidative stress (13). Activation of the Nrf2/HO-1 signaling pathway is commonly observed in malignant tumor cells with invasive and poor outcomes (14). Furthermore, Nrf2 can induce angiogenesis and promote cancer growth by stimulating the expression of HIF-1α-dependent vascular endothelial growth factor (VEGF) in cancer cells (14-16).
Berberine (BBR), an isoquinoline alkaloid derived from goldenseal, significantly influences blood glucose levels, insulin resistance, lipid profiles, inflammatory markers, colorectal adenomas, and Helicobacter pylori infections (17). Numerous studies suggest that BBR exhibits low toxicity in humans. Research indicates that BBR has minimal toxicity towards healthy cells (18). While it may lead to mild adverse effects such as constipation and nausea, these reactions are generally not severe, with the most prevalent issue of constipation resolving upon cessation of BBR (19,20). Numerous studies have reported the anticancer effects of BBR. For instance, BBR can inhibit the proliferation, invasion, and migration of GC by suppressing the AMPK/HNF4α/WNT5A signaling pathway (21). It also restricts the growth of human GC cells by inactivating the p38/JNK pathway, inducing mitochondria-mediated apoptosis, activating caspases, and inhibiting NF-κB (22). BBR induces autophagy by inhibiting the MAPK and Akt/mTOR/p70S6K pathways, thereby suppressing the growth of human GC cells both in vitro and in vivo (23). However, the regulatory role of BBR in GC-related oxidative stress has yet to be reported.
Network pharmacology is a discipline that integrates drug, target, and disease target genes into a multi-layered interactive biological network system through systems biology and bioinformatics (24). It is widely applied in traditional Chinese medicine (TCM) research for screening active ingredients, discovering targets, evaluating toxicity, and conducting mechanistic studies (25).
This study utilized network pharmacology methods to analyze and predict the biological processes and molecular mechanisms through which BBR may exert its effects in treating GC. Subsequent in vitro cell experiments were conducted to validate these findings, aiming to elucidate the mechanisms of BBR’s anti-GC properties. This research provides theoretical support for the development of novel therapeutic agents for GC and offers potential directions for expanding the clinical applications of BBR. Simultaneously, this study presents a novel perspective on the adjuvant treatment of GC, with the potential to enhance therapeutic efficacy, mitigate the side effects of chemotherapy, targeted therapy, or immunotherapy, alleviate patient suffering and financial burdens, improve quality of life, and even extend survival. We present this article in accordance with the MDAR reporting checklist (available at https://tcr.amegroups.com/article/view/10.21037/tcr-24-732/rc).
Methods
Databases and software
Traditional Chinese Medicine Systems Pharmacology (TCMSP) Database (https://tcmspw.com/tcmsp.php), Comparative Toxicogenomics Database (CTD) Database (http://ctdbase.org/), UniProt Database (https://www.Uniprot.org/), GeneCards Database (https://www.genecards.org/), Online Mendelian Inheritance in Man (OMIM) Database (http://www.omim.org/), PharmGkb Database (http://www.pharmgkb.org/), Therapeutic Target Database (TTD) Database (http://db.idrblab.net/ttd), DrugBank Database (https://go.drugbank.com/), Venny Platform (http://bioinfogp.cnb.csic.es/tools/venny/), STRING Database (https://string-db.org/), DAVID Database (https://david.ncifcrf.gov/home.jsp), PERL, R4.2.2, and Cytoscape 3.9.1 Softwares were used in this analysis. This study was conducted in accordance with the Declaration of Helsinki (as revised in 2013).
Cell lines
Human gastric adenocarcinoma cells AGS (1101HUM-PUMC000480) and Human GC cells MKN-45 (1101HUM-PUMC000229) cells were obtained from the Beijing Xiehe Cell Resource Center.
Drugs and reagents
BBR (No.A0151, CAS Accession No. 633-65-8, purity ≥98%) was purchased from Chengdu Mansite Biotechnology Co., Ltd. (China). It was dissolved in Dental Management Service Organization (DMSO) (D8371-50ml, Beijing Solarbio Science & Technology Co., Ltd., China) at a concentration of 107 mM, filtered through a 0.22 µm filter, and stored at 4 ℃ for later use. The Cell Counting Kit-8 (CCK-8) assay kit (GK10001-5) was obtained from Glpbio (CA, USA); the ROS fluorescent assay kit (E-BC-K138-F-96T) was purchased from Elabscience Biotechnology Co., Ltd. (China); the malondialdehyde (MDA) content assay kit (BC0025) was procured from Beijing Soleabio Technology Co., Ltd. The total superoxide dismutase (SOD) activity assay kit (S0101S) was obtained from Shanghai Biotree Biotechnology Co., Ltd. (China); 1640 medium (PM150110), Ham’s F-12 medium (PM150810), fetal bovine serum (164210-50), 1640 complete medium (PM150110B), and Ham’s F-12 complete medium (PM150810B) were all sourced from Wuhan Puno Sai Life Technology Co., Ltd. (China); trypsin (25200056) was acquired from Gibco (NY, USA); the BCA protein concentration determination kit (GK10009) was bought from Glpbio; protein-free rapid blocking solution (G2052-500ML) was purchased from Wuhan Saviour Biotechnology Co., Ltd. (China); primary antibodies for Nrf2 (380773), HO-1 (380753), HIF-1α (340462), and β-Actin (R23450), as well as the secondary antibody HRP-conjugated goat anti-rabbit monoclonal antibody (511203), were all obtained from Chengdu Zenbio Biotechnology Co., Ltd. (China); primary antibodies for Snail (Affinity Biosciences Cat# AF6032, RRID: AB_2834965) and Vimentin (Affinity Biosciences Cat# AF7013, RRID: AB_2835318) were sourced from Affinity Biosciences (OH, USA).
Acquisition and collection of BBR target genes
BBR target genes were obtained from the TCMSP and CTD databases, merged, and then validated using the UniProt database for gene name correction.
Retrieval of GC-related target genes and identification of BBR-GC intersection targets
Using “gastric cancer” as a keyword, GC-related target genes were searched in GeneCards, OMIM, PharmGkb, TTD, and DrugBank databases, and the intersection was visualized using Venn diagrams to identify potential targets of BBR for treating GC.
Construction of the “berberine-gastric cancer-target” network
ActivePerl software was employed to identify the target genes associated with BBR and GC, resulting in the creation of the net.network file, net.type file, net.geneLists file, and netolLists file. Subsequently, Cytoscape software was utilized to create the “berberine-gastric cancer-target” network diagram.
Protein-protein interaction (PPI) network construction
The intersecting targets of BBR and GC were entered into the online STRING database, with the species designated as “Homo sapiens”. The minimum interaction threshold was set to the highest confidence level (0.98), while free targets were concealed, resulting in the generation of a PPI network.
Topological analysis and core target selection
Cytoscape software was employed for the visualization of the PPI network, and the CytoNCA plugin was utilized for topological analysis of the PPI network. The analysis focused on three parameters: degree centrality (DC), betweenness centrality (BC), and closeness centrality (CC) to examine the topological characteristics of each node within the interaction network, thereby facilitating the identification of core targets. Finally, a PPI network of the core targets and a corresponding bar chart were constructed.
Gene Ontology (GO) and Kyoto Encyclopedia of Genes and Genomes (KEGG) pathway enrichment analysis
The GO functional enrichment analysis examines the enrichment levels of proteins and genes from three perspectives: biological processes (BP), cellular components (CC), and molecular functions (MF). The KEGG serves as a valuable database resource that enables the exploration of biological systems—such as cells, organisms, and ecosystems—at a molecular level, particularly through the insights gleaned from extensive molecular datasets generated by genome sequencing and other high-throughput experimental techniques. Employing R software modules such as “colorspace”, “stringi”, “ggplot2”, “DOSE”, “clusterProfiler”, and “enrichplot” to conduct GO function and KEGG pathway enrichment analysis of key target genes. The enrichment results were analyzed with a significance threshold of P>0.05 and visualized using bubble plots. The “pathview” module was used to generate pathway diagrams.
CCK-8 cell viability assay
CCK-8, a reagent designed for the straightforward and accurate assessment of cell proliferation and toxicity analysis. AGS and MKN45 GC cells in logarithmic growth phase were seeded at a concentration of 1×104/mL in a 96-well plate with 200 µL per well. After cell adherence, different concentrations of BBR (2.5, 5, 10, 20, 40 µM) were added to the experimental group, while the control group received DMSO. The plate was then incubated in a 37 ℃, 5% CO2 cell culture incubator for 24 and 48 h. After changing the culture medium, 10 µL of CCK-8 solution was added and incubated for 4 h. The absorbance (A) at 450 nm was measured using a microplate reader to assess cell viability.
Cell scratch assay
AGS and MKN45 GC cells in logarithmic growth phase were seeded at a concentration of 1×105/mL in a 24-well plate with 500 µL per well. After reaching 60–70% confluence, a linear scratch was made using a 10 µL pipette tip. The cells were washed with PBS to remove debris. The experimental group was treated with culture medium containing 40 µM BBR, while the control group received an equivalent amount of DMSO. The migration of cells at the scratch site was observed under an inverted microscope at 200× magnification. After 24 h of further incubation, cell migration was assessed by counting the cells that migrated into the scratch area.
Detection of ROS levels
AGS and MKN45 GC cells in logarithmic growth phase were seeded at a concentration of 1×105/mL in a 24-well plate. After reaching 60–70% confluence, the culture medium was replaced with medium containing 40 µM BBR for the experimental group, while the control group received an equivalent amount of DMSO. After 24 h of incubation, ROS levels were detected using the reactive oxygen species fluorescent probe (DCFH-DA) according to the manufacturer’s instructions. Fluorescence images were captured using a fluorescence microscope, with three images taken per well for statistical analysis.
Detection of MDA and SOD
AGS and MKN45 GC cells in the logarithmic growth phase were seeded in a 24-well plate at a density of 1×105/mL. The cells were incubated overnight in a culture chamber. The experimental group was treated with culture medium containing 40 µM BBR, while the control group was supplemented with an equivalent amount of DMSO, followed by an additional 24 hours of incubation. Subsequently, MDA and SOD levels were measured using respective detection kits in accordance with the manufacturer’s instructions. The experiment was performed in triplicate for statistical analysis.
Western blot analysis was performed to evaluate the expression levels of proteins associated with the oxidative stress signaling pathways HIF-1α, Nrf2/HO-1, and EMT
AGS and MKN45 GC cells in logarithmic growth phase were seeded at a concentration of 1×106/mL in a 6-well plate with 2 mL per well. After overnight incubation, the culture medium was replaced with medium containing 40 µM BBR for the experimental group, while the control group received an equivalent amount of DMSO. Following 24 h of incubation, cells were collected, and total protein was extracted. Protein concentrations were determined using a BCA assay. Western blot analysis was performed to assess the expression levels of the target proteins, with β-actin used as an internal reference.
Statistical analysis
Data are presented as mean ± standard deviation. Statistical analysis was performed using GraphPad Prism 9.5.0 software, with a t-test used for comparisons between two groups. Statistical significance was set at P<0.05 (*, P<0.05; **, P<0.01; ***, P<0.001), and non-significant differences were denoted as “ns” (P>0.05).
Results
Potential targets of BBR
By screening through the TCMSP and CTD databases, we identified BBR targets and subsequently standardized the information using the Uniprot database. This process facilitated the retrieval of protein names and the confirmation of the species as Homo sapiens, resulting in a total of 281 BBR target IDs.
GC-related targets
We gathered cancer-related targets from the GeneCards, OMIM, GKB, TTD, and DrugBank databases. After merging and removing duplicate genes, we identified 8,953 potential targets for GC, and we utilized R software to create a Venn diagram (Figure 1A).
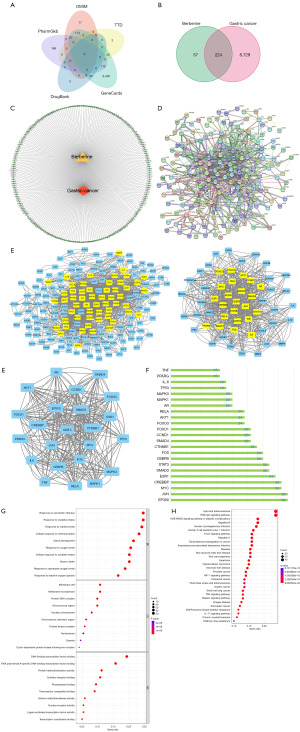
BBR-GC common targets
By taking the intersection of BBR and GC targets, a total of 224 potential targets for BBR in treating GC were identified. The Venn diagram depicting the common targets of BBR and GC is shown in Figure 1B.
“berberine-gastric cancer-targets” network
The intersecting target genes of BBR and GC were imported into Cytoscape software, resulting in the “BBR-Gastric Cancer-Target” network diagram. In this representation, yellow nodes signify BBR, red nodes represent GC, and green nodes denote the targets (Figure 1C).
PPI network of common targets of BBR and GC
The STRING database was employed to retrieve the previously identified intersecting targets of BBR and GC. “Homo sapiens” was selected as the species, with a confidence score set at 0.98. Independent genes were excluded in order to construct the PPI network (Figure 1D).
Topological analysis and core target selection
The PPI network was subjected to visualization and topological analysis using Cytoscape software to select core targets. The PPI network and bar graph of core targets are constructed (Figure 1E,1F). The top 23 ranked targets selected under specific conditions include EP300, c-Jun N-terminal kinase (JUN), MYC, CREBBP, ESR1, SMAD3, STAT3, CEBPB, FOS, CTNNB1, SMAD4, CCND1, FOXO1, FOXO3, AKT1, RELA, AR, MAPK1, MAPK3, TP53, IL-6, PPARG, and TNF.
GO functional enrichment analysis
GO functional enrichment analysis was conducted on the BBR-treated GC targets, resulting in 3,001 biological processes, 122 CCs, and 213 MFs. The top 10 GO terms were visualized in a bubble chart based on ascending P values (Figure 1G), including responses to external stimuli, oxidative stress responses, responses to nutrient levels, cellular responses to chemical stress, responses to oxygen levels, cellular responses to oxidative stress, neuron loss, gland development, responses to ROS, and responses to hypoxia. It is evident that oxidative stress plays a crucial role in BBR’s anti-GC effects.
KEGG pathway enrichment analysis
KEGG pathway enrichment analysis was performed on the BBR-treated GC targets, resulting in 176 relevant signaling pathways. The top 30 pathways were selected based on P values (Figure 1H). Through bubble chart analysis and considering tumor-related pathways, it was observed that the potential targets of BBR in treating GC primarily involve the phosphatidylinositol-3-kinase (PI3K)-protein kinase B (Akt) signaling pathway, Forkhead Box Protein O (FOXO), HIF-1, GC, and tumor necrosis factor (TNF) signaling pathways.
Inhibition of human GC cell proliferation by BBR
BBR at different concentrations (2.5, 5, 10, 20, 40 µM) could inhibit the activity of AGS and MKN45 cells after 24 hours of treatment (Figure 2A,2B). Notably, when the concentration of BBR was 2.5 µM, there was no statistically significant difference compared to the DMSO control group; however, differences were statistically significant for all other concentrations (P<0.05). The most significant inhibitory effect was observed at a concentration of 40 µM.
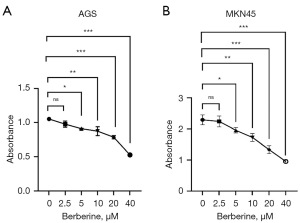
Inhibition of GC cell migration by BBR
AGS (Figure 3A) and MKN45 (Figure 3B) cells were treated with 40 µM BBR for 24 hours to observe the number of cells migrating towards the scratch area. The migration of AGS cells per field in the BBR group (55.67±3.68) was significantly lower than that in the control group (130.00±9.79), with a statistically significant difference (t=10.40, P<0.001). Similarly, the migration of MKN45 cells in the 40 µM BBR group (31.33±2.49) was significantly lower than that in the control group (102.70±6.13), with a statistically significant difference (t=15.25, P<0.001).
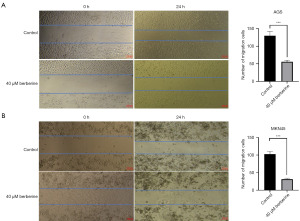
BBR enhances intracellular ROS levels in GC cells
AGS (Figure 4A) and MKN45 (Figure 4B) cells were treated with 40 µM BBR for 24 hours, and the number of ROS-stained cells was observed under a fluorescence microscope. The number of stained AGS cells per field in the BBR group (227.00±1.87) was significantly higher than that in the control group (88.33±4.26), with a statistically significant difference (t=36.49, P<0.001). Similarly, the number of stained MKN45 cells in the BBR group (295.00±6.28) was significantly higher than that in the control group (38.67±2.16), with a statistically significant difference (t=47.24, P<0.001).
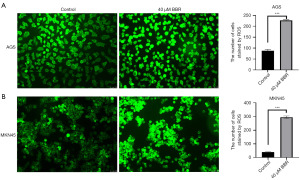
BBR significantly elevated the MDA levels in GC cells while concurrently reducing SOD levels
After 24 hours of BBR treatment in GC cells, the MDA concentrations in AGS cells (Figure 5A) for the 40 µM BBR group and the control group were (0.0310±0.0006) nmol/104 cells and (0.0189±0.0009) nmol/104 cells, respectively, exhibiting a statistically significant difference (t=20.29, P<0.001). In MKN45 cells (Figure 5B), the MDA concentrations for the 40 µM BBR group and the control group were (0.0305±0.0006) nmol/104 cells and (0.0203±0.0005) nmol/104 cells, also demonstrating a significant difference (t=21.74, P<0.001). Furthermore, in AGS cells (Figure 5C), the SOD concentrations for the 40 µM BBR group and the control group were (0.5601±0.0112) and (0.8327±0.0097) U/mg, respectively, with a statistically significant difference observed (t=31.32, P<0.001). In MKN45 cells (Figure 5D), the SOD concentrations for the 40 µM BBR group and the control group were (0.4343±0.0040) and (0.7387±0.0143) U/mg, respectively, revealing a significant difference (t=35.39, P<0.001). These experimental results conclusively indicate that BBR markedly elevates MDA levels while concurrently reducing SOD levels in GC cells. Conversely, SOD levels were markedly reduced (Figure 5C,5D), also showing a statistically significant difference (P<0.001).
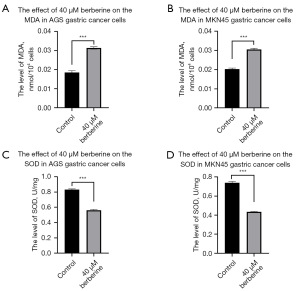
Regulation of Nrf2, HO-1, HIF-1α, Snail, and Vimentin protein expression by BBR
The Western blot analysis revealed that treatment with 40 µM BBR for 24 hours significantly suppressed the expression of HO-1 and Nrf2 (Figure 6A). The expression levels of these proteins in AGS (Figure 6B) and MKN45 (Figure 6C) cells presented statistically significant differences in comparison to the control group (P<0.001). Additionally, under the same intervention, BBR was shown to inhibit the expression of Snail, Vimentin, and HIF-1α proteins in GC cells (Figure 6D), with the expression levels in AGS (Figure 6E) and MKN45 (Figure 6F) cells exhibiting statistically significant differences relative to the control group (P<0.001).
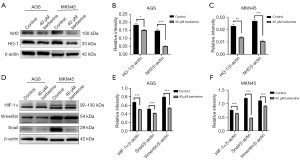
Discussion
Network pharmacology emerges as a novel, interdisciplinary frontier in the era of artificial intelligence and big data, tasked with the systematic integration of extensive, multi-source data from clinical and experimental contexts to construct intricate biological disease networks (26). This discipline deeply investigates the interactions among genes, proteins, drugs, and diseases, while elucidating the mechanisms of action of TCM from a microscopic molecular perspective. By revealing the material basis of TCM’s efficacy and integrating scientific validation, network pharmacology offers a clear direction for research, enhancing both efficiency and accuracy, and providing a theoretical foundation and technological support for rational clinical medication, as well as the development of new drugs and formulations (26). The field of TCM has been pioneering in proposing the core theoretical framework of network pharmacology, particularly the concept of network targets, which plays a pivotal role in its origin and evolution. This framework serves as a unique impetus for interpreting TCM principles through modern technology, thereby facilitating the modernization and internationalization of TCM (26). However, future research in TCM network pharmacology necessitates further standardization regarding data inclusion criteria, updating protocols, and data acquisition processes (26). Additionally, there is a pressing need to develop original algorithms for analyzing key targets and mechanisms. In 2021, the World Federation of Chinese Medicine Societies issued the “Guidelines for Network Pharmacology Evaluation Methods”, representing the first international standards specifically for network pharmacology assessment. These guidelines aim to encourage researchers to adopt a more scientific and standardized approach in their work. Moreover, it is imperative to closely integrate experimental validation with clinical applications, supplemented by big data analysis techniques and computational simulation technologies (26). This integration will refine and standardize the research processes within TCM network pharmacology, striving to render result validation more digitized and specific, thereby enhancing the interpretability of the validation process. This endeavor will elucidate the scientific essence of TCM’s syndrome differentiation and treatment theory, guiding its clinical application and propelling the modernization and internationalization of TCM.
In this study, network pharmacology methods were employed to predict the potential biological processes and molecular mechanisms of BBR in treating GC. The “Drug-Disease-Target” network analysis revealed that key target proteins such as JUN, MYC, CREBBP, ESR1, SMAD3, STAT3 are crucial nodes in the entire network, suggesting their significance as potential targets for BBR in treating GC. Therefore, BBR holds promise as an effective adjunctive therapy for GC.
GO functional enrichment analysis indicated that the biological processes mainly involved in BBR treatment of GC included responses to oxidative stress, oxygen levels, ROS, and hypoxia. This suggests that the regulation of oxidative stress plays a critical role in the anti-GC effects of BBR. Our experiments using relevant assay kits to measure the impact of BBR on intracellular ROS levels in GC cells confirmed that BBR significantly enhanced ROS levels within GC cells, thereby participating in the regulation of oxidative stress in GC and inducing apoptosis in GC cells. SOD is crucial antioxidant enzymes that catalyze the dismutation of superoxide anions into hydrogen peroxide and oxygen (27). Consequently, the expression and activation of SOD significantly influences the cellular oxidative response (28). MDA, a metabolic byproduct of lipid peroxidation, serves as a significant indicator of both the production of lipid peroxides and ROS in the body. Its presence can exacerbate cellular membrane damage, exhibiting cytotoxic properties (28,29). Our assays demonstrated that BBR notably elevated MDA levels in GC cells, suggesting that BBR plays a pivotal role in inducing cytotoxicity against GC. Concurrently, we found that BBR reduced the SOD levels in GC cells, thereby enhancing the toxic effects of ROS and MDA on these malignant cells. Subsequently, Western blot experiments were conducted to examine the Nrf2/HO-1 oxidative stress-related signaling pathway, revealing that BBR treatment led to a decrease in the expression of Nrf2/HO-1 pathway-related proteins in GC cells, suggesting that the inhibition of Nrf2/HO-1 pathway activation may be one of the key mechanisms of BBR’s anti-GC effects. This suggests that BBR undermines the inherent adaptive oxidative stress equilibrium of GC cells, thereby exerting its antitumor effects.
KEGG pathway enrichment analysis demonstrated that the common target genes of BBR and GC were significantly enriched in signaling pathways such as PI3K-Akt, FOXO, and HIF-1. Previous studies have shown that BBR can inhibit tumor cell proliferation, promote tumor cell apoptosis, inhibit angiogenesis and metastasis, with potential mechanisms focusing on activating AMPK to regulate tumor energy and oxygen metabolism, inhibiting cell survival-related pathways such as PI3K/AKT, thereby affecting common upstream and downstream targets such as ROS, mTOR, FOXO3a, and NF-κB (30,31). Through in vitro cell experiments and Western blot analysis, it was confirmed that BBR can inhibit the expression of HIF-1α protein in GC AGS and MKN45 cells, further validating the predictions of network pharmacology. Previous studies have demonstrated that HIF-1α can promote tumor EMT, thus we further examined the expression of EMT-related proteins Snail and Vimentin, revealing that BBR can significantly inhibit the expression of Snail and Vimentin proteins in GC AGS and MKN45 cells, thereby promoting the metastasis of GC cells. Therefore, it is postulated that inhibiting the activation of the HIF-1 signaling pathway and EMT may be one of the important mechanisms by which BBR influences the progression of GC.
However, there are certain limitations in this study such as the use of different databases leading to variations in target collection, as well as differences in screening criteria for data processing, which could result in potential omissions. Therefore, further in-depth research is needed on the mechanisms of BBR in anti-GC effects, in order to provide a theoretical basis for the use of BBR as an adjunctive therapy for GC and to actively develop its clinical applications.
Conclusions
This study utilized network pharmacology to explore the mechanisms of BBR in treating GC, followed by experimental validation, revealing that BBR exerts its therapeutic effects on GC through multiple targets and pathways, particularly by regulating oxidative stress in GC, including promoting ROS and MDA production while reducing SOD promoting to induce apoptosis in GC cells, and inhibiting the Nrf2/HO-1, HIF-1, and EMT signaling pathways to exert its anti-GC pharmacological effects.
Acknowledgments
None.
Footnote
Reporting Checklist: The authors have completed the MDAR reporting checklist. Available at https://tcr.amegroups.com/article/view/10.21037/tcr-24-732/rc
Data Sharing Statement: Available at https://tcr.amegroups.com/article/view/10.21037/tcr-24-732/dss
Pee Review File: Available at https://tcr.amegroups.com/article/view/10.21037/tcr-24-732/prf
Funding: This study was supported by
Conflicts of Interest: All authors have completed the ICMJE uniform disclosure form (available at https://tcr.amegroups.com/article/view/10.21037/tcr-24-732/coif). The authors have no conflicts of interest to declare.
Ethical Statement: The authors are accountable for all aspects of the work in ensuring that questions related to the accuracy or integrity of any part of the work are appropriately investigated and resolved. The study was conducted in accordance with the Declaration of Helsinki (as revised in 2013).
Open Access Statement: This is an Open Access article distributed in accordance with the Creative Commons Attribution-NonCommercial-NoDerivs 4.0 International License (CC BY-NC-ND 4.0), which permits the non-commercial replication and distribution of the article with the strict proviso that no changes or edits are made and the original work is properly cited (including links to both the formal publication through the relevant DOI and the license). See: https://creativecommons.org/licenses/by-nc-nd/4.0/.
References
- Rawla P, Barsouk A. Epidemiology of gastric cancer: global trends, risk factors and prevention. Prz Gastroenterol 2019;14:26-38. [Crossref] [PubMed]
- Joshi SS, Badgwell BD. Current treatment and recent progress in gastric cancer. CA Cancer J Clin 2021;71:264-79. [Crossref] [PubMed]
- Smyth EC, Nilsson M, Grabsch HI, et al. Gastric cancer. Lancet 2020;396:635-48. [Crossref] [PubMed]
- Hironaka S. Anti-angiogenic therapies for gastric cancer. Asia Pac J Clin Oncol 2019;15:208-17. [Crossref] [PubMed]
- Wu Z, Wang L, Wen Z, et al. Integrated analysis identifies oxidative stress genes associated with progression and prognosis in gastric cancer. Sci Rep 2021;11:3292. [Crossref] [PubMed]
- Bhattacharyya A, Chattopadhyay R, Mitra S, et al. Oxidative stress: an essential factor in the pathogenesis of gastrointestinal mucosal diseases. Physiol Rev 2014;94:329-54. [Crossref] [PubMed]
- Wang JY, Liu GZ, Wilmott JS, et al. Skp2-Mediated Stabilization of MTH1 Promotes Survival of Melanoma Cells upon Oxidative Stress. Cancer Res 2017;77:6226-39. [Crossref] [PubMed]
- Zhou F, Shen Q, Claret FX. Novel roles of reactive oxygen species in the pathogenesis of acute myeloid leukemia. J Leukoc Biol 2013;94:423-9. [Crossref] [PubMed]
- Oates JC, Gilkeson GS. The biology of nitric oxide and other reactive intermediates in systemic lupus erythematosus. Clin Immunol 2006;121:243-50. [Crossref] [PubMed]
- Smith J, Tho LM, Xu N, et al. The ATM-Chk2 and ATR-Chk1 pathways in DNA damage signaling and cancer. Adv Cancer Res 2010;108:73-112. [Crossref] [PubMed]
- Li J, Wu DD, Zhang JX, et al. Mitochondrial pathway mediated by reactive oxygen species involvement in α-hederin-induced apoptosis in hepatocellular carcinoma cells. World J Gastroenterol 2018;24:1901-10. [Crossref] [PubMed]
- Ou XW, Wang RX, Kang MF, et al. Hypoxia promotes migration and invasion of gastric cancer cells by activating HIF-1α and inhibiting NDRG2 associated signaling pathway. Eur Rev Med Pharmacol Sci 2018;22:8237-47. [PubMed]
- Imai S, Koizumi S, Sugiura M, et al. Gastric carcinoma: monoclonal epithelial malignant cells expressing Epstein-Barr virus latent infection protein. Proc Natl Acad Sci U S A 1994;91:9131-5. [Crossref] [PubMed]
- Jaramillo MC, Zhang DD. The emerging role of the Nrf2-Keap1 signaling pathway in cancer. Genes Dev 2013;27:2179-91. [Crossref] [PubMed]
- Ma Q. Role of nrf2 in oxidative stress and toxicity. Annu Rev Pharmacol Toxicol 2013;53:401-26. [Crossref] [PubMed]
- Li R, Jia Z, Zhu H. Regulation of Nrf2 Signaling. React Oxyg Species (Apex) 2019;8:312-22. [PubMed]
- Li Z, Wang Y, Xu Q, et al. Berberine and health outcomes: An umbrella review. Phytother Res 2023;37:2051-66. [Crossref] [PubMed]
- Och A, Podgórski R, Nowak R. Biological Activity of Berberine-A Summary Update. Toxins (Basel) 2020;12:713. [Crossref] [PubMed]
- Fang S, Guo S, Du S, et al. Efficacy and safety of berberine in preventing recurrence of colorectal adenomas: A systematic review and meta-analysis. J Ethnopharmacol 2022;282:114617. [Crossref] [PubMed]
- Mirzaee F, Razmjouei P, Shahrahmani H, et al. The effect and safety of Berberine on polycystic ovary syndrome: a systematic review. J Obstet Gynaecol 2021;41:684-9. [Crossref] [PubMed]
- Hu Q, Li L, Zou X, et al. Berberine Attenuated Proliferation, Invasion and Migration by Targeting the AMPK/HNF4α/WNT5A Pathway in Gastric Carcinoma. Front Pharmacol 2018;9:1150. [Crossref] [PubMed]
- Wang Y, Zhou M, Shang D. Berberine inhibits human gastric cancer cell growth via deactivation of p38/JNK pathway, induction of mitochondrial-mediated apoptosis, caspase activation and NF-κB inhibition. J BUON 2020;25:314-8. [PubMed]
- Zhang Q, Wang X, Cao S, et al. Berberine represses human gastric cancer cell growth in vitro and in vivo by inducing cytostatic autophagy via inhibition of MAPK/mTOR/p70S6K and Akt signaling pathways. Biomed Pharmacother 2020;128:110245. [Crossref] [PubMed]
- Yuan Z, Pan Y, Leng T, et al. Progress and Prospects of Research Ideas and Methods in the Network Pharmacology of Traditional Chinese Medicine. J Pharm Pharm Sci 2022;25:218-26. [Crossref] [PubMed]
- Luo TT, Lu Y, Yan SK, et al. Network Pharmacology in Research of Chinese Medicine Formula: Methodology, Application and Prospective. Chin J Integr Med 2020;26:72-80. [Crossref] [PubMed]
- Wang ZY, Wang X, Zhang DY, et al. Network Pharmacology of Traditional Chinese Medicine: Development in a New Era Guided by the Guidelines. China Journal of Chinese Materia Medica 2022;47:7-17. [PubMed]
- Miller AF. Superoxide dismutases: ancient enzymes and new insights. FEBS Lett 2012;586:585-95. [Crossref] [PubMed]
- Huang B, Ding C, Zou Q, et al. Cyclophosphamide Regulates N6-Methyladenosine and m6A RNA Enzyme Levels in Human Granulosa Cells and in Ovaries of a Premature Ovarian Aging Mouse Model. Front Endocrinol (Lausanne) 2019;10:415. [Crossref] [PubMed]
- Yin Y, Han W, Cao Y. Association between activities of SOD, MDA and Na+-K+-ATPase in peripheral blood of patients with acute myocardial infarction and the complication of varying degrees of arrhythmia. Hellenic Journal of Cardiology 2019;60:366-71. [Crossref] [PubMed]
- Hanahan D, Weinberg RA. Hallmarks of cancer: the next generation. Cell 2011;144:646-74. [Crossref] [PubMed]
- Huang J, Feng W, Li S, et al. Berberine Exerts Anti-cancer Activity by Modulating Adenosine Monophosphate- Activated Protein Kinase (AMPK) and the Phosphatidylinositol 3-Kinase/ Protein Kinase B (PI3K/AKT) Signaling Pathways. Curr Pharm Des 2021;27:565-74. [Crossref] [PubMed]