Testosterone and colorectal cancer: a bidirectional Mendelian randomization study
Highlight box
Key findings
• This study revealed a causal effect of bioavailable testosterone (BT) on the risk of colorectal cancer (CRC).
What is known and what is new?
• Many studies suggest that there is no association between testosterone and CRC. Our research also found that total testosterone does not impact CRC.
• We are the first to demonstrate a causal relationship of BT on CRC.
What is the implication, and what should change now?
• Our results enhance the understanding of the real causal relationship between BT and CRC. For individuals with elevated BT levels, regular screening for CRC is necessary. Additionally, for CRC patients, lowering BT levels may help prevent further progression of the disease to some extent.
Introduction
Colorectal cancer (CRC) is the third most common cancer globally and the second leading cause of cancer-related deaths (1). CRC comprises about 10% of all newly diagnosed cancer cases and cancer-related deaths globally each year. It is the second most common cancer in women and the third most in men. Women experience approximately 25% lower incidence and mortality rates compared to men. It is estimated that by 2035, the global incidence of CRC will rise to over 2.5 million new cases (2,3).
Testosterone, an androgen, serves significant physiological functions in both sexes (4). It predominantly binds to sex hormone-binding globulin (SHBG) and human serum albumin (HSA). Bioavailable testosterone (BT) denotes the portion of circulating testosterone not bound to SHBG, mainly comprising free testosterone and HSA-bound testosterone. HSA-bound testosterone, having low affinity, can detach from HSA in capillaries, becoming effectively available for biological activity (5).
Mendelian randomization (MR) analyses showed that testosterone increases the risk of prostate cancer (6), but the relationship between testosterone and CRC remains highly controversial, with numerous clinical studies showing conflicting conclusions. An MR analysis of men in the UK Biobank (UKB) showed no causal relationship between BT and total testosterone (TT) and CRC risk conducted by Chang et al. (7). Inconsistent findings may come from cross-sectional designs in most prior studies, potential confounding factors, or limited sample sizes. Animal experiments also showed varied results. Hence, evidence from large-scale prospective studies is still lacking.
In cross-sectional studies, testosterone levels exhibit age-related changes, increasing during puberty and declining later in life. However, testosterone levels often remain relatively stable between the ages of 20 and 70 years (8). In a subsample of 7,097 men and 5,285 women from the UKB, testosterone levels were reassessed 5 years later. The results demonstrated that individual baseline testosterone levels remain quite stable over time (9). Although some studies showed that estradiol may also influence the occurrence of CRC, the analysis of this trait in UKB was constrained due to the majority having estradiol levels below the limit of detection. Consequently, the genome wide association studies (GWAS) were hindered by limited sample sizes and a bias towards identifying loci linked to female age at menopause (6). Hence, we did not explore the association between estradiol and CRC.
MR is a bioinformatics analytical approach that utilizes GWAS data to explore the causal associations. MR analysis employs single nucleotide polymorphisms (SNPs) as instrumental variables (IVs) to substitute exposure factors, thereby mitigating the impacts of confounding factors. MR investigations typically involve extensive sample sizes, helping to reduce the issue of reverse causation commonly observed in observational epidemiological studies. As a result, MR studies tend to yield findings with enhanced credibility (10). However, the MR result remains controversial. We utilized genetic data from the UKB and Finngen to evaluate the bidirectional MR relationships between TT and BT with the risk of CRC, using a larger sample size and more stringent methods to exclude confounding factors. We present this article in accordance with the STROBE-MR reporting checklist (available at https://tcr.amegroups.com/article/view/10.21037/tcr-24-1481/rc).
Methods
Data sources
GWAS data of TT and BT were obtained from The IEU Open GWAS project: TT (ebi-a-GCST90012114, 425,097 samples), BT (ebi-a-GCST90012104, 382,988 samples). The GWAS data of CRC were obtained from Finngen R11 (8,801 cases, 345,118 controls). The original GWAS data of TT and BT all originated from the UKB. All participants were Europeans. In fact, the MR study by Chang et al. (7) used male testosterone data as exposure and all CRC data from both males and females as outcome. In our study, we used both male and female data, which doubled the sample size for exposure. Besides, the number of cases for CRC outcome increased by more than 100%. The study was conducted in accordance with the Declaration of Helsinki (as revised in 2013).
IVs selection
According to the principles of MR, the IVs utilized for analysis must satisfy three assumptions: (I) IVs exhibit strong associations with the exposure. (II) IVs are not associated with confounding factors. (III) IVs affect the risk of outcomes solely through exposure, not through other pathways (11,12). In the MR analysis, SNPs were selected as IVs to serve as mediators between exposure factors and outcomes only if they met the following criteria: (I) SNPs demonstrated significant associations with exposures at the genome-wide significance level (P<5e–8). (II) There was no linkage disequilibrium among the included IVs, with a linkage disequilibrium correlation coefficient set to r2<0.001 and a clumping window >10,000 kb. (III) The F-statistic was employed to avoid weak instrument bias. The F-statistic >10 was considered to satisfy the requirements of strong association (13). Later, we searched for traits of each SNP in the National Human Genome Research Institute-European Bioinformatics Institute (NHGRI-EBI) Catalog (https://www.ebi.ac.uk/gwas/home). In the forward MR analysis, we excluded SNPs associated with confounding factors [ulcerative colitis, Crohn’s disease, inflammatory bowel disease, cholesterol, low-density lipoprotein, high-density lipoprotein, very low-density lipoprotein, body mass index (BMI), cancer, smoking, alcohol, height, education]. If the traits of an SNP contained the above-mentioned fields, then the SNP was removed. Compared to the MR study by Junke Chang et al. (7), which excluded BMI, alcohol consumption, and years of educational attainment as confounding factors, our study eliminated confounding factors more comprehensively. In the reverse MR analysis, we excluded SNPs associated with confounding factors (testosterone, prostate). Then, we performed harmonization to make the alleles of each SNP consistent between the exposure and outcome. Besides, we employed the MR-pleiotropy residual sum and outlier (MR-PRESSO) method, which automatically identifies outliers in IVW linear regression and eliminates them to obtain corrected MR estimation (14). The distortion test of MR-PRESSO analysis was utilized to identify outliers. The global test of MR-PRESSO analysis was conducted to assess the horizontal pleiotropy, with P>0.05 indicating no horizontal pleiotropy. We excluded relevant SNPs to ensure that the P value of the global test in MR-PRESSO was over 0.05.
MR analysis
The inverse variance weighting (IVW) method was used as the primary analytical approach (15). The IVW method uses a meta-analysis technique to integrate Wald ratio assessments of the causal connection derived from multiple SNPs, yielding a dependable evaluation of the causal relationship (16). Meanwhile, MR-Egger, weighted median, simple mode and weighted mode were used to infer the causal relationship. MR-Egger remains unbiased even in the presence of nonzero mean pleiotropy but may sacrifice statistical power (17). Weighted medians offer robust estimates of effective IVs with a minimum weight of 50% (18). The simple mode represents a model-based evaluation method providing robustness against pleiotropy (19). The weighted mode is effective as long as the majority of IVs satisfy the requirements for MR causal inference (17). We utilized all these methods to comprehensively investigate causality. The two-sample MR analysis was conducted using the “TwoSampleMR” package (version 0.5.9) in R software (version 4.4.0).
Sensitivity analysis
The Cochran’s Q statistic (MR-IVW) and Rucker’s Q statistic (MR-Egger) were employed to assess heterogeneity, with P>0.05 indicating no significant heterogeneity (20). The intercept test of MR-Egger was utilized to identify horizontal pleiotropy, with P>0.05 indicating no presence of horizontal pleiotropy (21). The leave-one-out analysis was used to investigate whether the causal relationships were influenced by individual SNPs.
Statistical analysis
We used MR analysis to assess the causal relationship between BT, TT, and CRC, with a P value <0.05 considered statistically significant. The MR-PRESSO method was applied to remove outliers to enhance the robustness of the results. Heterogeneity and pleiotropy tests were further conducted to assess result stability, with P>0.05 indicating robust findings.
Results
Selection of IVs
The final IVs obtained for each MR analysis are presented in https://cdn.amegroups.cn/static/public/tcr-24-1481-1.xlsx. All these SNPs satisfied F-statistic >10, were unrelated to confounding factors, and were not excluded by MR-PRESSO.
The causal effects of TT and BT on CRC
The bidirectional MR analysis revealed one standard deviation (SD) increase in genetically predicted BT increased the risk of CRC [IVW: odds ratio (OR) =1.834, 95% confidence interval (CI): 1.121−3.001, P=0.02]. There was no causal effect of TT on CRC (Figure 1). Besides, there was no causal relationship of CRC on BT or TT (Figure 2). The OR values of the five methods for the bidirectional causal relationship between BT and CRC were all >1, indicating the robustness of the causal relationship. The Cochran’s Q test, Rucker’s Q test, MR-PRESSO test and MR-Egger intercept test showed our results were not affected by heterogeneity or pleiotropy (Table 1).
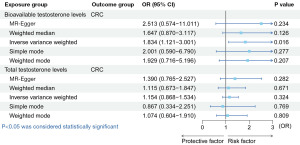
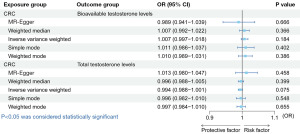
Table 1
Exposure | Outcome | Heterogeneity test | Pleiotropy test (Egger intercept, MR-Egger) | MR-PRESSO (Global test) | |
---|---|---|---|---|---|
Cochran’s Q test, IVW | Rucker’s Q test, MR-Egger | ||||
Bioavailable testosterone levels | CRC | 0.09 | 0.07 | 0.66 | 0.09 |
Total testosterone levels | CRC | 0.16 | 0.15 | 0.49 | 0.17 |
CRC | Bioavailable testosterone levels | 0.37 | 0.34 | 0.47 | 0.39 |
CRC | Total testosterone levels | 0.47 | 0.50 | 0.28 | 0.50 |
The data are presented as P values. MR, Mendelian randomization; IVW, inverse variance weighting; MR-PRESSO, MR-pleiotropy residual sum and outlier; CRC, colorectal cancer.
The scatter plots of causal relationships were shown in Figure S1.
Leave-one-out analysis for the impact of individual SNPs on bidirectional MR between BT and TT with CRC were shown in Figures S2-S5. The results showed that the causal estimates of our MR analysis were not influenced by individual SNPs, suggesting that our MR analysis is stable.
Discussion
To date, our MR analysis is the first to investigate the causal relationship between BT and CRC. The results showed an association between elevated BT levels and increased risk of CRC. Furthermore, the results of bidirectional MR analysis did not support the reverse causal relationship of CRC on BT levels. There was no causal relationship between CRC and TT which is consistent with previous MR research results conducted by Chang et al. (7). Compared to the unidirectional MR studies by Chang et al., our research adopted bidirectional MR to investigate the bidirectional causal relationship between testosterone and CRC. Besides, our study had a larger sample size and removed a more comprehensive set of confounding factors. These results confirm the potential of BT as a biomarker for CRC and provide additional evidence for the connection between CRC and TT as well as BT, thereby enhancing our understanding of the complex interplay involved.
Due to the fact that TT includes BT as well as the portion of testosterone bound to SHBG which does not directly exert biological effects, we infer that this difference leads to the different causal relationships between BT and TT with CRC.
Over time, the relationship between testosterone and CRC has been highly contentious, with many studies pointing to conflicting conclusions. An animal experiment indicated that male sex hormones, particularly testosterone, may induce colonic adenomas (the precancerous lesions of CRC). Male mice injected with the carcinogen azoxymethane for 6 weeks showed higher susceptibility to adenoma development compared to females. Orchidectomy reduced adenoma formation significantly in male mice. Further experiments on male mice subjected to orchidectomy showed that those receiving intramuscular injections of testosterone enanthate exhibited increased susceptibility to adenomatous lesions compared to those who received a placebo alone (22). A retrospective study involving 5,404 postmenopausal women revealed a correlation between higher levels of free testosterone and increased risk of conventional adenomas. The study results suggest the role of free testosterone in the onset of early-stage CRC (23). In vitro, testosterone was demonstrated to promote the growth of colon cancer cells, and this effect can be reduced by anti-androgens (24).
However, there are also studies suggesting the opposite conclusion. It was reported that hypotestosteronemia can lead to CRC and men undergoing androgen deprivation therapy for prostate cancer treatment had an increased risk of CRC (25,26). These studies suggest a protective role of testosterone against CRC.
Another study conducted on samples from the UKB using Cox proportional hazards models showed that testosterone is not associated with CRC (27). This contradicts the causal relationship uncovered in our bidirectional MR analysis, where it was observed that BT increased the risk of CRC. The different results obtained in the two studies may be attributed to biases introduced by unmeasured or residual confounding factors that observational studies cannot address.
The Testosterone Trials in men represent the largest randomized controlled trials (RCTs) of testosterone administration at present. However, data on CRC outcomes remains insufficient. Compared to evidence from RCTs, genetic IV analyses are less susceptible to confounding and reverse causality, providing a more reliable evidence base.
Besides, certain potential mechanisms may contribute to further understanding the interrelationships. Testosterone binds to the androgen receptor NR3C4 which is a DNA-binding transcription factor, thereby regulating gene expression and protein synthesis to promote tissue proliferation (4). This mechanism may be associated with the development of CRC.
Our research suggests the potential of BT as a biomarker for CRC. It indicates the need for regular CRC screening in patients with elevated BT levels, and that reducing BT levels may help lower the risk of CRC.
However, several limitations of this study should be acknowledged. First, because of the absence of gender-stratified GWAS data in CRC, MR studies were not conducted by gender. Second, genetic instruments represent lifelong exposures to the risk factor, making it difficult to determine the effects of short-medium term pharmacological interventions. Therefore, further clinical trials are required to validate our findings. Third, our genetic analysis was limited to samples of European ancestry, which restricts our ability to generalize the results to other populations.
Conclusions
Our research provides evidence of a causal relationship of BT on CRC risk, and CRC does not affect the level of BT. Furthermore, there is no causal relationship between TT and CRC. All these findings offer new insights into the complex associations involved. However, large-scale RCTs are still needed to further confirm this in the future.
Acknowledgments
We express our appreciation to the individuals and researchers whose work contributed to the public database utilized in this study, as well as the authors of all R packages used in this article. Besides, we also extend our gratitude to our mentor because this article would not have been possible without his support.
Footnote
Reporting Checklist: The authors have completed the STROBE-MR reporting checklist. Available at https://tcr.amegroups.com/article/view/10.21037/tcr-24-1481/rc
Peer Review File: Available at https://tcr.amegroups.com/article/view/10.21037/tcr-24-1481/prf
Funding: None.
Conflicts of Interest: All authors have completed the ICMJE uniform disclosure form (available at https://tcr.amegroups.com/article/view/10.21037/tcr-24-1481/coif). The authors have no conflicts of interest to declare.
Ethical Statement: The authors are accountable for all aspects of the work in ensuring that questions related to the accuracy or integrity of any part of the work are appropriately investigated and resolved. The study was conducted in accordance with the Declaration of Helsinki (as revised in 2013).
Open Access Statement: This is an Open Access article distributed in accordance with the Creative Commons Attribution-NonCommercial-NoDerivs 4.0 International License (CC BY-NC-ND 4.0), which permits the non-commercial replication and distribution of the article with the strict proviso that no changes or edits are made and the original work is properly cited (including links to both the formal publication through the relevant DOI and the license). See: https://creativecommons.org/licenses/by-nc-nd/4.0/.
References
- Siegel RL, Miller KD, Fedewa SA, et al. Colorectal cancer statistics, 2017. CA Cancer J Clin 2017;67:177-93. [Crossref] [PubMed]
- Arnold M, Sierra MS, Laversanne M, et al. Global patterns and trends in colorectal cancer incidence and mortality. Gut 2017;66:683-91. [Crossref] [PubMed]
- Bray F, Ferlay J, Soerjomataram I, et al. Global cancer statistics 2018: GLOBOCAN estimates of incidence and mortality worldwide for 36 cancers in 185 countries. CA Cancer J Clin 2018;68:394-424. [Crossref] [PubMed]
- Roshan MH, Tambo A, Pace NP. The role of testosterone in colorectal carcinoma: pathomechanisms and open questions. EPMA J 2016;7:22. [Crossref] [PubMed]
- Goldman AL, Bhasin S, Wu FCW, et al. A Reappraisal of Testosterone's Binding in Circulation: Physiological and Clinical Implications. Endocr Rev 2017;38:302-24. [Crossref] [PubMed]
- Ruth KS, Day FR, Tyrrell J, et al. Using human genetics to understand the disease impacts of testosterone in men and women. Nat Med 2020;26:252-8. [Crossref] [PubMed]
- Chang J, Wu Y, Zhou S, et al. Genetically predicted testosterone and cancers risk in men: a two-sample Mendelian randomization study. J Transl Med 2022;20:573. [Crossref] [PubMed]
- Handelsman DJ, Sikaris K, Ly LP. Estimating age-specific trends in circulating testosterone and sex hormone-binding globulin in males and females across the lifespan. Ann Clin Biochem 2016;53:377-84. [Crossref] [PubMed]
- Leinonen JT, Mars N, Lehtonen LE, et al. Genetic analyses implicate complex links between adult testosterone levels and health and disease. Commun Med (Lond) 2023;3:4. [Crossref] [PubMed]
- Emdin CA, Khera AV, Kathiresan S. Mendelian Randomization. JAMA 2017;318:1925-6. [Crossref] [PubMed]
- Burgess S, Davey Smith G, Davies NM, et al. Guidelines for performing Mendelian randomization investigations: update for summer 2023. Wellcome Open Res 2023;4:186. [Crossref] [PubMed]
- Skrivankova VW, Richmond RC, Woolf BAR, et al. Strengthening the Reporting of Observational Studies in Epidemiology Using Mendelian Randomization: The STROBE-MR Statement. JAMA 2021;326:1614-21. [Crossref] [PubMed]
- Staiger DO, Stock JH. Instrumental variables regression with weak instruments. National Bureau of Economic Research Cambridge, Mass., USA; 1994.
- Verbanck M, Chen CY, Neale B, et al. Detection of widespread horizontal pleiotropy in causal relationships inferred from Mendelian randomization between complex traits and diseases. Nat Genet 2018;50:693-8. [Crossref] [PubMed]
- Wu F, Huang Y, Hu J, et al. Mendelian randomization study of inflammatory bowel disease and bone mineral density. BMC Med 2020;18:312. [Crossref] [PubMed]
- Pierce BL, Burgess S. Efficient design for Mendelian randomization studies: subsample and 2-sample instrumental variable estimators. Am J Epidemiol 2013;178:1177-84. [Crossref] [PubMed]
- Hartwig FP, Davey Smith G, Bowden J. Robust inference in summary data Mendelian randomization via the zero modal pleiotropy assumption. Int J Epidemiol 2017;46:1985-98. [Crossref] [PubMed]
- Bowden J, Davey Smith G, Haycock PC, et al. Consistent Estimation in Mendelian Randomization with Some Invalid Instruments Using a Weighted Median Estimator. Genet Epidemiol 2016;40:304-14. [Crossref] [PubMed]
- Milne RL, Kuchenbaecker KB, Michailidou K, et al. Identification of ten variants associated with risk of estrogen-receptor-negative breast cancer. Nat Genet 2017;49:1767-78. [Crossref] [PubMed]
- Hemani G, Zheng J, Elsworth B, et al. The MR-Base platform supports systematic causal inference across the human phenome. Elife 2018;7:e34408. [Crossref] [PubMed]
- Burgess S, Thompson SG. Interpreting findings from Mendelian randomization using the MR-Egger method. Eur J Epidemiol 2017;32:377-89. [Crossref] [PubMed]
- Amos-Landgraf JM, Heijmans J, Wielenga MC, et al. Sex disparity in colonic adenomagenesis involves promotion by male hormones, not protection by female hormones. Proc Natl Acad Sci U S A 2014;111:16514-9. [Crossref] [PubMed]
- Hang D, He X, Kværner AS, et al. Plasma sex hormones and risk of conventional and serrated precursors of colorectal cancer in postmenopausal women. BMC Med 2021;19:18. [Crossref] [PubMed]
- Tutton PJ, Barkla DH. The influence of androgens, anti-androgens, and castration on cell proliferation in the jejunal and colonic crypt epithelia, and in dimethylhydrazine-induced adenocarcinoma of rat colon. Virchows Arch B Cell Pathol Incl Mol Pathol 1982;38:351-5. [Crossref] [PubMed]
- Gould DC, Petty R. The male menopause: does it exist?: for: some men need investigation and testosterone treatment. West J Med 2000;173:76-8. [Crossref] [PubMed]
- Gillessen S, Templeton A, Marra G, et al. Risk of colorectal cancer in men on long-term androgen deprivation therapy for prostate cancer. J Natl Cancer Inst 2010;102:1760-70. [Crossref] [PubMed]
- Peila R, Arthur RS, Rohan TE. Sex hormones, SHBG and risk of colon and rectal cancer among men and women in the UK Biobank. Cancer Epidemiol 2020;69:101831. [Crossref] [PubMed]