Adenanthin inhibits pancreatic cancer proliferation by regulation of H2O2/ROS
Highlight box
Key findings
• Adenanthin can make the content of hydrogen peroxide (H2O2) exceed the endurance limit of pancreatic cancer cells by regulating the content of H2O2, thus leading to DNA damage, cycle arrest and apoptosis of pancreatic cancer cells, and ultimately leading to the death of pancreatic cancer cells.
What is known and what is new?
• Previous studies have found that adenanthin can inhibit the activity of peroxiredoxin (Prx) I/II, leading to the accumulation of intracellular reactive oxygen species (ROS) and ultimately killing liver cancer cells. However, there have been no reports on the inhibitory effect of adenanthin on pancreatic cancer cells.
• In this study, adenanthin can inhibit the proliferation and induce apoptosis of pancreatic cancer cells both in vivo and in vitro.
What is the implication, and what should change now?
• Adenanthin is expected to become a new drug against pancreatic cancer.
Introduction
Pancreatic cancer is a highly malignant disease that is characterized by rapid progression and poor prognosis (1,2). To date, the therapeutic efficacy of drugs against pancreatic cancer has been unsatisfactory, and the development of innovative anti-pancreatic cancer drugs has encountered significant obstacles. Therefore, it is of great significance to conduct in-depth research on the mechanisms of the occurrence and development of pancreatic cancer, to discover new proteins that have significant impact on pancreatic cancer, and to provide new targets for the development of innovative anti-pancreatic cancer drugs.
Adenanthin is a diterpenoid compound extracted from the herb Rabdosia adenantha that has anti-inflammatory and anti-tumor activities (3). Previous study (4) found that adenanthin can induce intracellular reactive oxygen species (ROS) accumulation by inhibiting the activity of peroxiredoxin (Prx) I/II, ultimately leading to the killing of liver cancer cells. Multiple studies have also confirmed the specific targeting of Prx by adenanthin and its inhibitory effect on its activity (4,5), and have used it as a natural inhibitor of Prx for related mechanistic studies (6-8). However, so far, there have been no reports on the inhibitory effect of adenanthin on pancreatic cancer cells. It has only been reported that Prx I is highly expressed in pancreatic cancer tissues and is significantly associated with the prognosis of pancreatic cancer; increased expression of Prx I is associated with poorer prognosis (9). The Prx family is considered to be the most important enzyme for decomposing hydrogen peroxide (H2O2) in the body. Current views suggest that Prx can regulate ROS by controlling the level of H2O2, thereby reducing oxidative stress, decreasing tumor cell apoptosis, promoting proliferation, and on the other hand, can directly activate tumor growth signals by regulating ROS, thereby promoting tumor proliferation and metastasis (10-12).
Adenanthin, as a natural inhibitor of Prx, exerts significant effects on the proliferation and apoptosis of pancreatic cancer through the regulation of H2O2/ROS but its specific mechanisms has not been reported. Therefore, this study focuses on adenanthin to investigate its regulatory effects on the proliferation of pancreatic cancer cells and its potential mechanisms, with the aim of adenanthin becoming a new drug for anti-pancreatic cancer. We present this article in accordance with the MDAR and ARRIVE reporting checklists (available at https://tcr.amegroups.com/article/view/10.21037/tcr-24-874/rc).
Methods
Experimental cells and tissue specimens
The human pancreatic cancer cell line Aspc-1 was purchased from the Cell Bank of the Shanghai Institute of Cell Biology, Chinese Academy of Sciences. Cancer adjacent tissue and cancer tissue specimens from six pancreatic cancer patients were obtained from surgeries at Suzhou Kowloon Hospital. The study was conducted in accordance with the Declaration of Helsinki (as revised in 2013). The study was approved by the Ethics Committee of Suzhou Kowloon Hospital (approval number: Kowloon Ethics Review KY-2022-001), and informed consent was obtained from all the patients.
Major reagents and instruments
Adenanthin was purchased from Shanghai Biotech Pharmaceutical Technology Co., Ltd. (Shanghai, China); Cell Counting Kit-8 (CCK-8) and Hoechast 33258 reagents were purchased from Shanghai Biyun Tian Biotechnology Co., Ltd. (Shanghai, China); TRIzol was purchased from Invitrogen (USA); cell cycle assay kit and apoptosis assay kit were purchased from Jiangsu Kaiji Biotechnology Co., Ltd. (Nanjing, China); enzyme linked immunosorbent assay (ELISA) kits were purchased from Hangzhou Union-Biotechnology Co., Ltd. (Hangzhou, China); all antibodies were purchased from Cell Signaling Technology (USA); polymerase chain reaction (PCR) primers were synthesized by Shanghai Sangon Biotech Co., Ltd. (Shanghai, China).
The Western blot electrophoresis system was purchased from Bio-Rad (USA); the multifunctional enzyme labeling instrument was purchased from Thermo Fisher (USA).
Experimental methods
CCK-8 assay for cell proliferation
Aspc-1 cells were seeded in a 96-well plate at a density of 2,000 cells/well and treated with different concentrations of adenanthin (5, 1, 0.5, 0.1, 0.05, 0.01, 0 µM) for 1, 2, 3, and 5 days (with medium change every two days). Then, 20 µL of CCK-8 solution was added to each well, followed by 1 hour of incubation. Absorbance at 450 nm was measured using a microplate reader to indirectly assess cell viability.
Colony formation assay
Cells were seeded in a 6-well plate at a density of 1,000 cells/well and treated with different concentrations of drugs (0, 0.1, 0.5, 1 µM) the next day. After 14 days of incubation, colonies were fixed, stained with 0.5% crystal violet (Sigma Aldrich, USA), and counted under a microscope. Colony inhibition rate was calculated.
Annexin-V assay for apoptosis detection
Aspc-1 cells in logarithmic growth phase were treated with different concentrations of adenanthin (1, 0.5, 0 µM) for 48 hours. Annexin-V and propidium iodide (PI) staining were performed, followed by flow cytometry analysis.
Hochest 33258 staining
Aspc-1 cells were treated with different concentrations of adenanthin (1, 0.5, 0 µM) for 48 hours, fixed, stained with Hochest 33258, and observed under a fluorescence microscope.
Cell cycle analysis
Aspc-1 cells were treated with different concentrations of adenanthin (1, 0.5, 0 µM) for 48 hours, fixed, stained, and analyzed using flow cytometry.
ELISA for H2O2 and ROS detection
Aspc-1 cells were treated with different concentrations of adenanthin (1, 0.5, 0 µM) for 24 hours, and the levels of H2O2 and ROS in the cells were measured according to the ELISA kit instructions.
Tumor xenograft mouse model
The nude mice (~20 g, 4 weeks, male) were purchased from Beijing Vital River Laboratory Animal Technology Co. Ltd. (Beijing, China). All mice were maintained in a Specific-Pathogen-Free facility (Grade = Nasal-1000) and subcutaneously injected with Aspc-1 cells. Mice with tumor volumes ranging from 100 to 300 mm3 were selected for subsequent grouping and drug experiments. A protocol was prepared before the study without registration. Mice were divided into three groups, with 10 mice in each group: control group receiving saline, low-dose adenanthin group (10 mg/kg/day), and high-dose adenanthin group (30 mg/kg/day). Treatment was administered via intraperitoneal injection for 20 days, with tumor volume and mouse weight measured every two days. After 21 days, mice were euthanized, tumors were collected, and protein expression was analyzed by Western blot. All animal experiments involved in this study were approved by the Animal Experimentation Ethics Committee at Genepharma Company (No. IACUC-2022539), in compliance with institutional guidelines for the care and use of animals.
Western blot
Aspc-1 cells in logarithmic growth phase were harvested and seeded into a 96-well plate, then treated with different concentrations of adenanthin (1, 0.5, 0.1, 0 µM) for 48 hours. After incubation, cellular proteins were collected. The bicinchoninic acid (BCA) protein assay kit was utilized to quantify the protein content within the cells. Proteins were separated by Sodium Dodecyl Sulfate (SDS)-polyacrylamide gel electrophoresis and subsequently transferred onto polyvinylidene fluoride (PVDF) membranes. The membranes were incubated overnight at 4 °C with primary antibodies, followed by incubation with secondary antibodies at room temperature for 1 hour. Protein bands were detected using an enhanced chemiluminescence (ECL) system.
Statistical analysis
Data analysis was performed using GraphPad Prism 7 software. All experiments were independently repeated three times, and results were expressed as mean ± standard deviation (SD). Homogeneity of variance was tested, and if the data met the assumptions of normal distribution and homogeneity of variance, one-way analysis of variance (ANOVA) was employed, followed by least significant difference-t (LSD-t) test for pairwise comparisons. If variance homogeneity was not met, non-parametric methods were used. The significance level was set at α=0.05, denoted by * for P<0.05, ** for P<0.01, *** for P<0.001, and **** for P<0.0001.
Results
Expression of Prx I in pancreatic cancer tissues is significantly higher than adjacent tissues
Tissues from six pancreatic cancer patients, including cancer adjacent tissues and cancer tissues, were collected for Western blot analysis to detect the expression of Prx I. The results showed a significant increase in the expression of Prx I in pancreatic cancer tissues compared to adjacent tissues (Figure 1). This suggests that the elevated expression of Prx I may be associated with the prognosis of pancreatic cancer, consistent with previous reports, indicating the potential involvement of Prx I in the proliferation of pancreatic cancer.
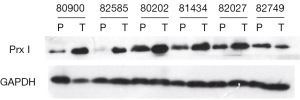
Inhibition of Aspc-1 cell proliferation by adenanthin
We subsequently used the reported small molecule inhibitor of Prx I, adenanthin, as a probe to test its activity on pancreatic cancer cells (Aspc-1). Aspc-1 cells were seeded at a density of 2,000 cells/well in a 96-well plate and treated with different concentrations of adenanthin (20, 10, 5, 1, 0.5, 0.1, 0.05, 0.01, 0 µM) for 1, 2, 3 days, and 1 µM adenanthin was used to treat cells for 1, 2, 3, 5 days, followed by CCK-8 assays to measure cell viability. The results showed that adenanthin could significantly inhibit cell proliferation in a time- and dose-dependent manner (Figure 2A).
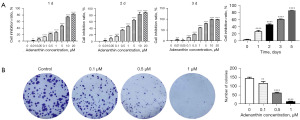
To further validate the antitumor effect of adenanthin, we performed a colony formation assay. Based on the above experimental results, we selected concentrations of 0.1, 0.5, and 1 µM adenanthin to treat Aspc-1 cells. Cells were seeded at a specific concentration in a 6-well plate and allowed to grow overnight, followed by treatment with different drugs the next day and continued incubation in the culture chamber for 14 days, after which fixation and staining were performed. The results revealed that adenanthin significantly inhibited the proliferation of pancreatic cancer cells, significantly reduced the growth of pancreatic cancer cells, and the colony formation rate of cells treated with 1 µM adenanthin was as low as 1%. These results indicate that adenanthin can significantly inhibit the proliferation of pancreatic cancer cells (Figure 2B).
Adenanthin induces apoptosis in pancreatic cancer cells
The above results suggest that adenanthin can significantly inhibit the proliferation and growth of pancreatic cancer cells. To investigate whether this inhibitory effect is associated with apoptosis, we used flow cytometry to detect apoptosis in cells after treatment with different concentrations of the drug for 48 hours. The results showed that treatment with adenanthin for 48 hours significantly induced apoptosis in Aspc-1 cells, with early apoptotic cell rates of 6.36%, 17.24%, and 26.43% for the dimethyl sulfoxide (DMSO)/0.5 µM/1 µM treatment groups, respectively (Figure 3A). The overall apoptosis statistics are shown in Figure 3B.
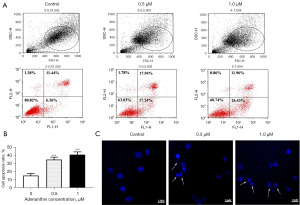
In the Hoechst 33258 staining experiment, after 48 hours of treatment with adenanthin, Aspc-1 cells exhibited nuclear condensation, chromatin aggregation, and the presence of apoptotic bodies. Moreover, with increasing concentrations, the number of cells decreased, the number of apoptotic bodies increased, a large number of cells exhibited cytoplasmic shrinkage, chromatin condensation, and fragmented nuclei, displaying typical apoptotic morphology (Figure 3C).
Adenanthin blocks the cell cycle in pancreatic cancer cells
Adenanthin can inhibit the proliferation and growth of cells by inducing apoptosis. To investigate whether adenanthin has an impact on the cell cycle, cells were incubated with different concentrations of adenanthin (1, 0.5, 0 µM) for 48 hours, followed by flow cytometry to assess the distribution of the cell cycle in pancreatic cancer cells after treatment with different concentrations of adenanthin. The results revealed that adenanthin significantly blocked Aspc-1 cells at the S phase and G2/M phase. The ratios of G1/S/G2M phases for the DMSO group were 60.21%/16.1%/17.38%, for the 0.5 µM compound treated for 48 hours were 2.49%/14.72%/74.92%, and for the 1 µM compound treated for 48 hours were 1.86%/57.12%/32.67% (Figure 4). These results indicate that adenanthin can induce cell death and inhibit the growth of tumor cells by blocking the cell cycle.
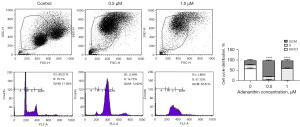
Adenanthin increases H2O2 and ROS levels in pancreatic cancer cells
H2O2 is a common intermediate product of cellular oxygen metabolism in aerobic organisms. Current research suggests that tumor cells are more sensitive to H2O2 compared to normal cells, and elevated levels of H2O2 can lead to cell cycle arrest and apoptosis in tumor cells. Prx is the most important protein for degrading H2O2, and its role in tumors is also crucial. As a natural inhibitor of Prx, adenanthin may have a significant impact on the proliferation and apoptosis of pancreatic cancer by regulating H2O2. We measured the H2O2 concentration in Aspc-1 cells using an H2O2 detection kit and detected ROS levels in Aspc-1 cells using a ROS detection kit. The results showed that adenanthin treatment significantly increased the levels of H2O2 and ROS in pancreatic cancer cells (Figure 5).
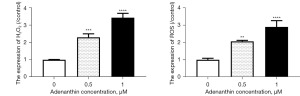
Adenanthin affects the expression of apoptosis and cell cycle-related proteins
The aforementioned studies suggest that adenanthin can effectively inhibit the growth and proliferation of pancreatic cancer cells and can influence the proliferation and apoptosis of pancreatic cancer by regulating the levels of H2O2 and ROS. To further explore the underlying mechanisms of adenanthin’s inhibitory effects on pancreatic cancer cells, we examined the expression of apoptosis and cell cycle-related proteins. The results showed that after 48 hours of adenanthin treatment, the expression of apoptosis-related proteins caspase 9 and Bax increased, while the anti-apoptotic protein Bcl-2 decreased in the cells. Cyclin B1, P21, and cdc 25A are all cell cycle-related proteins. When cells experience G2/M phase arrest, the expression of cyclin B1 and cdc 25A decreases, while P21 expression increases. Western blot analysis revealed an increase in P21 expression and a decrease in the expression of cyclin B1 and cdc 25A (Figure 6). These results indicate that adenanthin’s inhibition of Prx I can lead to the regulation of H2O2 levels, exceeding the tolerance limit of pancreatic cancer cells, thereby causing DNA damage and promoting cell cycle arrest and apoptosis, ultimately leading to the death of pancreatic cancer cells.
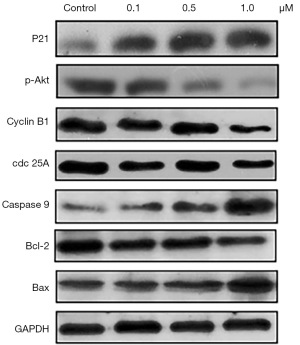
In vivo validation of adenanthin’s inhibitory effect on pancreatic cancer
To explore the inhibitory effect of adenanthin on pancreatic cancer in vivo, we established a subcutaneous xenograft model in nude mice. One group was given physiological saline as a control, another group was administered a low dose of adenanthin (10 mg/kg/day), and a third group was given a high dose of adenanthin (30 mg/kg/day). After euthanasia, the tumors were excised to measure their weight, and Western blot analysis was conducted to examine the expression of relevant proteins. The results showed that consistent with the in vitro studies, high-dose adenanthin treatment effectively controlled tumor growth. The expression of apoptosis-related proteins caspase 9 and Bax increased, while the anti-apoptotic protein Bcl-2 decreased. The expression of cell cycle proteins cyclin B1 and cdc 25A decreased (Figure 7).
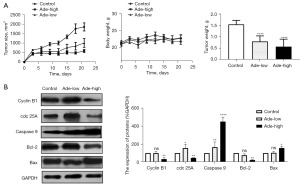
Discussion
This study primarily investigated the role and regulatory mechanisms of adenanthin in the proliferation of pancreatic cancer cells. The findings revealed that adenanthin could significantly induce apoptosis and G2/M phase arrest in pancreatic cancer cells. To further explore its molecular mechanisms, we studied the expression of apoptosis proteins Bax/Bcl-2, caspase 9, and G2/M phase-related proteins cyclin B1, P21, and cdc 25A. We found that adenanthin-treated pancreatic cancer cells had increased expression of apoptosis proteins Bax/Bcl-2 and caspase 9, decreased expression of cell cycle-related proteins cyclin B1 and cdc 25A, and increased expression of P21. ELISA experiments further confirmed that adenanthin could significantly increase the levels of H2O2 and ROS, indicating that adenanthin can increase H2O2 levels to reach the tolerance limit of pancreatic cancer cells, thereby triggering DNA damage responses (DDR) and mitochondrial damage, causing cell cycle arrest in pancreatic cancer cells and inducing apoptosis, ultimately contributing to the treatment of pancreatic cancer. In vivo experiments in mice further confirmed the inhibitory effect of adenanthin on pancreatic cancer. Therefore, adenanthin may become a new drug for the treatment of pancreatic cancer.
Multiple studies have confirmed that adenanthin can specifically target Prx I/II and inhibit its activity, thereby exerting antitumor effects (4,5). Prx is a class of peroxidase belonging to the superfamily of antioxidant proteins. Prx I is primarily distributed in the cytoplasm and nucleus and is involved in cell signaling, particularly in the regulation of tumorigenesis. It has been confirmed that Prx I is overexpressed in various tumors, including pancreatic cancer, breast cancer, colorectal cancer, etc., and its high expression is associated with the progression of pancreatic cancer (13). Our study found that the expression of Prx I in pancreatic cancer tissues was significantly higher than that in adjacent tissues, suggesting that it can be used as an auxiliary indicator for exploring pancreatic cancer prognosis. However, its regulatory role in tumors has not been fully elucidated. Reports have found that Prx I can promote the metastasis of pancreatic cancer through the regulation of the P38 pathway (14), but there is little reporting on its effect on pancreatic cancer proliferation. Adenanthin, as a natural inhibitor of Prx I, exerts an inhibitory effect on the proliferation of pancreatic cancer cells by inhibiting the activity of Prx I.
Study (15) has found that adenanthin can influence the development of obesity by regulating ROS and H2O2. Additionally, adenanthin can selectively kill hepatocellular carcinoma cells by inhibiting the activity of Prx I/II and causing ROS accumulation in cells (4). H2O2 is a common intermediate product of cellular oxygen metabolism in aerobic organisms (16). Tumor cells are more sensitive to H2O2 than normal cells and are more likely to die due to excessive or insufficient H2O2 levels. Low concentrations of H2O2 act as signaling molecules to regulate cell division and growth, but when the amount of H2O2 exceeds a certain critical value, the cell cycle can be arrested or apoptosis triggered (17). It is currently believed that excessive H2O2 causes DNA damage, thereby triggering complex DDR, ultimately leading to cell cycle arrest or apoptosis. DDR causes ataxia telangiectasia mutated (ATM) and Chk2 phosphorylation to increase, leading to the upregulation of P53 and P21, thereby causing cell cycle arrest (18-20); on the other hand, excessive H2O2 causes mitochondrial damage, thereby initiating apoptosis, and H2O2 can also activate Caspase 3, thereby initiating apoptosis (21-24). We have reason to believe that the antitumor effect of adenanthin is through the regulation of H2O2 levels, thereby regulating ROS and increasing oxidative stress to promote tumor cell apoptosis and inhibit their proliferation. Our study found that adenanthin treatment significantly increased the levels of H2O2, ROS, and P21 expression in pancreatic cancer cells, and the changes in apoptosis and cell cycle proteins further confirmed this.
However, there are a few limitations of this study: Our study mainly preliminarily studied the inhibitory effect of adenanthin on pancreatic cancer cells through cell and mouse experiments. The research on the specific mechanism is still insufficient. Besides H2O2/ROS pathway, it is not clear whether there are other pathways. It is also unclear whether adenanthin inhibits the proliferation of pancreatic cancer through Prx I and clarify the role of Prx I. In the future, we will knock-out Prx I and study the exact mechanism by which H2O2/ROS regulates pancreatic cancer cell death.
Conclusions
Prx I is highly expressed in pancreatic cancer, and adenanthin, a small molecule inhibitor of Prx I, can exceed the tolerance limit of pancreatic cancer cells by regulating the levels of H2O2, thereby causing DNA damage and prompting pancreatic cancer cells to undergo cycle arrest and apoptosis, ultimately leading to the death of pancreatic cancer cells.
Acknowledgments
None.
Footnote
Reporting Checklist: The authors have completed the MDAR and ARRIVE reporting checklists. Available at https://tcr.amegroups.com/article/view/10.21037/tcr-24-874/rc
Data Sharing Statement: Available at https://tcr.amegroups.com/article/view/10.21037/tcr-24-874/dss
Peer Review File: Available at https://tcr.amegroups.com/article/view/10.21037/tcr-24-874/prf
Funding: This work was supported by
Conflicts of Interest: All authors have completed the ICMJE uniform disclosure form (available at https://tcr.amegroups.com/article/view/10.21037/tcr-24-874/coif). The authors have no conflicts of interest to declare.
Ethical Statement: The authors are accountable for all aspects of the work in ensuring that questions related to the accuracy or integrity of any part of the work are appropriately investigated and resolved. The study was conducted in accordance with the Declaration of Helsinki (as revised in 2013). The study was approved by the Ethics Committee of Suzhou Kowloon Hospital (approval number: Kowloon Ethics Review KY-2022-001), and informed consent was obtained from all the patients. Animal experiments were approved by Animal Experimentation Ethics Committee at Genepharma Company (No. IACUC-2022539), in compliance with institutional guidelines for the care and use of animals.
Open Access Statement: This is an Open Access article distributed in accordance with the Creative Commons Attribution-NonCommercial-NoDerivs 4.0 International License (CC BY-NC-ND 4.0), which permits the non-commercial replication and distribution of the article with the strict proviso that no changes or edits are made and the original work is properly cited (including links to both the formal publication through the relevant DOI and the license). See: https://creativecommons.org/licenses/by-nc-nd/4.0/.
References
- Siegel RL, Miller KD, Wagle NS, et al. Cancer statistics, 2023. CA Cancer J Clin 2023;73:17-48. [Crossref] [PubMed]
- Li ZC, Li ZX, Zhang Y, et al. Interpretation of the 2020 Global Cancer Statistics Report 2020. Journal of Multidisciplinary Cancer Management 2021;7:1-13.
- Jiang B, Yang H, Li ML, et al. Diterpenoids from Isodon adenantha. J Nat Prod 2002;65:1111-6. [Crossref] [PubMed]
- Hou JK, Huang Y, He W, et al. Adenanthin targets peroxiredoxin I/II to kill hepatocellular carcinoma cells. Cell Death Dis 2014;5:e1400. [Crossref] [PubMed]
- Liu CX, Yin QQ, Zhou HC, et al. Adenanthin targets peroxiredoxin I and II to induce differentiation of leukemic cells. Nat Chem Biol 2012;8:486-93. [Crossref] [PubMed]
- Dos Santos MC, Tairum CA, Cabrera VIM, et al. Adenanthin Is an Efficient Inhibitor of Peroxiredoxins from Pathogens, Inhibits Bacterial Growth, and Potentiates Antibiotic Activities. Chem Res Toxicol 2023;36:570-82. [Crossref] [PubMed]
- O'Leary PC, Terrile M, Bajor M, et al. Peroxiredoxin-1 protects estrogen receptor α from oxidative stress-induced suppression and is a protein biomarker of favorable prognosis in breast cancer. Breast Cancer Res 2014;16:R79. [Crossref] [PubMed]
- Yin QQ, Liu CX, Wu YL, et al. Preventive and therapeutic effects of adenanthin on experimental autoimmune encephalomyelitis by inhibiting NF-κB signaling. J Immunol 2013;191:2115-25. [Crossref] [PubMed]
- Cai CY, Zhai LL, Wu Y, et al. Expression and clinical value of peroxiredoxin-1 in patients with pancreatic cancer. Eur J Surg Oncol 2015;41:228-35. [Crossref] [PubMed]
- Tochhawng L, Deng S, Pervaiz S, et al. Redox regulation of cancer cell migration and invasion. Mitochondrion 2013;13:246-53. [Crossref] [PubMed]
- Tobar N, Villar V, Santibanez JF. ROS-NFkappaB mediates TGF-beta1-induced expression of urokinase-type plasminogen activator, matrix metalloproteinase-9 and cell invasion. Mol Cell Biochem 2010;340:195-202. [Crossref] [PubMed]
- Ho JN, Lee SB, Lee SS, et al. Phospholipase A2 activity of peroxiredoxin 6 promotes invasion and metastasis of lung cancer cells. Mol Cancer Ther 2010;9:825-32. [Crossref] [PubMed]
- Hampton MB, Vick KA, Skoko JJ, et al. Peroxiredoxin Involvement in the Initiation and Progression of Human Cancer. Antioxid Redox Signal 2018;28:591-608. [Crossref] [PubMed]
- Taniuchi K, Furihata M, Hanazaki K, et al. Peroxiredoxin 1 promotes pancreatic cancer cell invasion by modulating p38 MAPK activity. Pancreas 2015;44:331-40. [Crossref] [PubMed]
- Hu J, Li X, Tian W, et al. Adenanthin, a Natural ent-Kaurane Diterpenoid Isolated from the Herb Isodon adenantha Inhibits Adipogenesis and the Development of Obesity by Regulation of ROS. Molecules 2019;24:158. [Crossref] [PubMed]
- Auten RL, Davis JM. Oxygen toxicity and reactive oxygen species: the devil is in the details. Pediatr Res 2009;66:121-7. [Crossref] [PubMed]
- Halliwell B. The antioxidant paradox: less paradoxical now? Br J Clin Pharmacol 2013;75:637-44. [Crossref] [PubMed]
- Liu SL, Lin X, Shi DY, et al. Reactive oxygen species stimulated human hepatoma cell proliferation via cross-talk between PI3-K/PKB and JNK signaling pathways. Arch Biochem Biophys 2002;406:173-82. [Crossref] [PubMed]
- Chua PJ, Yip GW, Bay BH. Cell cycle arrest induced by hydrogen peroxide is associated with modulation of oxidative stress related genes in breast cancer cells. Exp Biol Med (Maywood) 2009;234:1086-94. [Crossref] [PubMed]
- Park IJ, Hwang JT, Kim YM, et al. Differential modulation of AMPK signaling pathways by low or high levels of exogenous reactive oxygen species in colon cancer cells. Ann N Y Acad Sci 2006;1091:102-9. [Crossref] [PubMed]
- Chen JP, Xu DG, Yu XY, et al. Discrepancy between the effects of morronside on apoptosis in human embryonic lung fibroblast cells and lung cancer A549 cells. Oncol Lett 2014;7:927-32. [Crossref] [PubMed]
- Su H, Liu DD, Zhao M, et al. Dual-Enzyme Characteristics of Polyvinylpyrrolidone-Capped Iridium Nanoparticles and Their Cellular Protective Effect against H2O2-Induced Oxidative Damage. ACS Appl Mater Interfaces 2015;7:8233-42. [Crossref] [PubMed]
- Chirino YI, Sánchez-Pérez Y, Osornio-Vargas AR, et al. PM(10) impairs the antioxidant defense system and exacerbates oxidative stress driven cell death. Toxicol Lett 2010;193:209-16. [Crossref] [PubMed]
- Hanschmann EM, Godoy JR, Berndt C, et al. Thioredoxins, glutaredoxins, and peroxiredoxins--molecular mechanisms and health significance: from cofactors to antioxidants to redox signaling. Antioxid Redox Signal 2013;19:1539-605. [Crossref] [PubMed]