Analysis and exploration of regulatory mechanisms and potential prognostic biomarkers in squamous cell carcinoma of the lung by expression profiling
Highlight box
Key findings
• This study constructed two competing endogenous RNA networks centered on the long non-coding RNAs MAGI2-AS3 and LINC01089.
What is known, and what is new?
• Despite advances in diagnosis and treatment, patients with squamous cell lung cancer are often diagnosed at a late stage, and have a poor prognosis due to tumor metastasis and recurrence. There is an unmet need for identifying optimal prognostic and predictive biomarkers for this disease.
• MAGI2-AS3 and MBNL2 are differentially expressed in squamous cell carcinoma of the lung and could serve as potential prognostic markers for this disease. The high expression of MBNL2 can inhibit cancer cell proliferation and migration yet promote cancer cell apoptosis.
What is the implication, and what should change now?
• Our findings revealed the prognostic role of two novel biomarkers that can potentially be further applied on treatment of patients with squamous cell carcinoma of the lung.
Introduction
Lung cancer is one of the most common malignant tumors worldwide, and has a high incidence and mortality rate (1). In the United States, lung cancer causes approximately 350 deaths per day (2). Histologically, lung cancer can be divided into two major types: small cell lung cancer (SCLC) and non-small cell lung cancer (NSCLC) (3).
NSCLC accounts for approximately 85% of all lung cancer cases (4), and can be divided into three major subtypes: lung adenocarcinoma (LUAD), lung squamous cell carcinoma (LUSC), and large cell lung cancer (5). LUSC accounts for approximately 25–30% of all lung cancer cases (6) and 30% of NSCLC cases. It is mostly diagnosed in middle-aged and elderly men, and is associated with a poorer prognosis due to high rates of recurrence and metastases (7). LUSC causes approximately 400,000 deaths annually worldwide (5). LUSC originates from the epithelial cells lining the airways, specifically through transformation of squamous cells in the bronchial tubes. It typically arises in the central parts of the lungs, in the larger bronchi, and that is the reason why it is often referred to as a central type of lung cancer (8). Risk factors for the development and progression of LUSC include exposures to tobacco products (smoking), occupational exposures, living amidst polluted air, and exposures to ionizing radiation (9,10). Despite extensive research in this area, the pathogenesis of LUSC is still not completely elucidated.
Squamous cell lung cancer patients are mostly diagnosed at a late stage (11), and have a poor prognosis due to increased risk for tumor metastases and recurrence (12,13), resulting in 5-year survival rate of only 15% (11). There have been significant advances in the last few decades with advent of targeted therapy, however, these therapies are mostly applicable for non-squamous NSCLC. For LUSC, immunotherapy (programmed cell death-1/programmed cell death-ligand 1 inhibitors) +/− chemotherapy has become the best treatment method for those with advanced-stage NSCLC whose tumors cannot be surgically removed (14,15). However, there are many limitations with immunotherapy, including its lack of efficacy in a significant proportion of patients, rare but potentially serious immune related adverse events, and prohibitive high-cost (16). Additionally, some patients with underlying comorbidities such as active autoimmune disease are unable to receive the agents for immunotherapy. Thus, there is an urgent need for new biomarkers in LUSC that can help predict response to treatment and survival, provide avenues for targeted therapies, and add to our understanding of this disease.
Competing endogenous RNA network (ceRNA network) is a widely existing regulatory network. Under traditional conditions, microRNA (miRNA) exerts its function of regulating gene expression by binding to miRNA response elements (MREs) of messenger RNA (mRNA), and further guides the activation of target genes by Argonaute proteins for degradation. However, recent studies have suggested that other long non-coding RNAs (lncRNAs) with the same MRE can competitively absorb miRNAs, thereby affecting their regulatory effects on target genes. This type of lncRNA is called ceRNA, and the extensive interactions between genes mediated by miRNA are called the ceRNA network (17,18). LncRNAs are found to be an important regulatory factor responsible for various biological processes such as cell growth, cell migration, cell invasion, and metabolic rewiring (19). Abnormally expressed lncRNAs are considered potential alternative biomarkers or therapeutic targets for NSCLC (20). Although more and more lncRNAs have been annotated, the specific role and molecular mechanism of lncRNAs in non-small cell lung cancer are still poorly understood (21).
This article is based on public databases to screen for and identify differential expression in lung cancer. Then, through pathway enrichment analysis, the pathways that these genes may be involved in were identified, and their targeting relationships were predicted. Finally, their targeting relationship was further validated through wet experiments. The expression of the identified differentially expressed genes (DEGs) and their impact on tumor cell proliferation in lung cancer cell lines were validated. By combining dry and wet experiments, the results of the article are made more reliable. We present this article in accordance with the MDAR reporting checklist (available at https://tcr.amegroups.com/article/view/10.21037/tcr-2024-2443/rc).
Methods
Cell culture
The human LUSC line NCI-H520 (H520) was purchased from the American TypeCulture Collection (Manassas, VA, USA), and maintained in low-passage cultures as recommended. Briefly, the H520 cells were cultured in Roswell Park Memorial Institute 1640 medium (Sigma, St. Louis, MO, USA) supplemented with 10% fetal bovine serum (FBS) and 1% penicillin-streptomycin at 37 ℃ in a humidified atmosphere of 5% carbon dioxide (CO2).
Public data analysis
The Gene Expression Profiling Interactive Analysis (GEPIA2, http://gepia2.cancer-pku.cn/) web server was first released in 2017, and is used to conduct gene expression analyses of tumor and normal samples from The Cancer Genome Atlas (TCGA, https://www.cancer.gov/ccg/research/genome-sequencing/tcga) and Genotype-Tissue Expression (GTEx, https://www.genome.gov/Funded-Programs-Projects/Genotype-Tissue-Expression-Project) databases. This study mainly analyzed the gene expression of LUSC and paraneoplastic tissues in TCGA and GTEx. The study was conducted in accordance with the Declaration of Helsinki (as revised in 2013).
Gene Set Enrichment Analysis (GSEA) is a widely used technique in transcriptome data analysis that uses a predefined gene set database to sort the list of genes from microarray studies to identify significant and coordinated changes in gene expression data. In this study, the log2 (fold-change) values of differentially expressed mRNAs and sample grouping information were imported, and the pathways were analyzed using the GSEA function in the R “clusterProfiler” package (version 2.1.4). The pathways with P <0.05 were selected for presentation. The “gseaplot2” function in R “enrichplot” package (version 1.24.4) was used for pathway visualization.
The miRNAs targeting the mRNAs were predicted using the miRTarBase (https://mirtarbase.cuhk.edu.cn/~miRTarBase/miRTarBase_2019/php/index.php), while articles on miRNA function research were collected and selected manually. The targeting relationships were identified in the miRTarBase. The targeting relationships presented in the miRTarBase were experimentally validated by reported paper, western blots, microarrays, and next-generation sequencing experiments. After obtaining the genes in the database, we selected the DEGs to construct the miRNA-mRNA networks. The regulatory networks were visualized using Cytoscape (version 3.6.1).
Cell transfection
The recombinant lentivirus was produced by Shanghai Genechem Company (Shanghai, China). The short-hairpin RNA (shRNA) sequence (CCGGGC-target sequence-CTCGAG-reverse complementary target sequence-GCTTTTTG) was subcloned into the lentiviral silencing vector, and scramble shRNA (GCCTAACTGTGTCAGAAGGAA) was used as the negative control. The designed shRNA sequence of KIAA0101 was packaged with lentivirus and subsequent transfection experiments were performed after the sequencing results were found consistent. The cells (2×105) were placed in a 12-well plate, and suitable eukaryotic resistance was chosen to screen the cells (blasticidin full lethal concentration: 8 µg/mL; maintenance concentration: 4 µg/mL). After stabilization, the cells were maintained using Dulbecco’s Modified Eagle Medium (DMEM) supplemented with 10% FBS and 1% penicillin-streptomycin and 4 µg/mL blasticidin complete medium. The overexpression plasmids were subsequently transfected and co-transfected with shRNA following the above steps. The experiments were conducted on two groups: the control group (the scramble shRNA group) and the shKIAA0101 group.
The MBNL2 overexpression lentiviral vector was constructed and verified by Hanbio Technology Co., Ltd. (Hanbio, Shanghai, China). For the lentiviral transfection, Opti-MEM (Invitrogen, Waltham, MA, USA) was used as the medium with the appropriate amounts of viral vectors and 5 mg/mL polybrene (Hanbio). Next, puromycin was used to select stably transfected H520 cells.
Anti-MBNL2 small interfering RNA (siRNA) was purchased from GenePharma (Shanghai, China) and siRNA negative control (si-NC) was used as a control agent. The mimics and inhibitors of miR-374a-5p were prepared and compared at Sanbang Biotechnology (Beijing, China) using nitrocellulose (NC) mimics and NC inhibitor as the control. All transfections were performed using INTERFERin® siRNA and miRNA transfection reagents (Polyplus, New York, NY, USA).
Dual luciferase assay
The sequences of the MAGI2-AS3 and MBNL2 binding sites (the three prime untranslated regions) and the predicted miR-374a-5p binding sites were amplified by polymerase chain reaction (PCR). The wild-type MAGI2-AS3 reporter gene (MAGI2-AS3-wt) and wild-type MBNL2 reporter gene (MBNL2-wt) were constructed using the GeneArt™ Site-Directed Mutagenesis System (Thermo Fisher Scientific, Waltham, MA, USA). The constructed MAGI2-AS3-wt, MBNL2-wt, miR-negative control (miR-NC), or miR-374a-5p mimics were transfected into the H520 cells using GP-miRGLO plasmid and incubated in an incubator for 36 h. Then the Dual Lumi™ Dual Luciferase Reporter Assay Kit (Beyotime Biotechnology, Nantong, China) was used to measure luciferase activity and the experiments were performed in triplicate.
Quantitative-PCR
Trizol reagent (Invitrogen, Waltham, MA, USA) was used to isolate the total RNA from the tissues and cells. The RNA was reversed to complementary DNA using HiScript®II QRT SuperMix (Vazyme, Nanjing, China). Quantitative real-time PCR (qRT-PCR) was conducted using QuantStudio 5 Real-Time PCR System (Applied Biosystems, Waltham, MA, USA) and the dye ChamQ Universal SYBR qPCR Master Mix (Vazyme, Nanjing, China). Gene expression was calculated using the 2−ΔΔCt method, and glyceraldehyde-3-phosphate dehydrogenase (GAPDH) served as the internal control. Primer sequences were as follows: lncRNA MAGI2-AS3 (F): 5'-CACCTTGCTTGACAACTTGA-3' and lncRNA MAGI2-AS3 (R), miR-374a-5p (F): 5'-GCGCGCTTATAATACAACCTGA-3' and miR-374a-5p (R): 5'-GTGCAGGGTCCGAGGT-3'; MBNL2 (F): 5'-AGCGGCGCAACATCAAGCTA-3' and MBNL2 (R): 5'-CTGCAACTGAGCATTGGCAAG-3'; U6 (F): 5'-CTCGCTTCGGCAGCACA-3' and U6 (R): 5'-ACGCTTCACGAATTTGCGT-3'; GAPDH (F), 5'-ACCACAGTCCATGCCATCAC-3' and GAPDH (R): 5'-TCCACCACCCTGTTGCTGTA-3'.
Western blot analysis
The stable cell strains were removed from the incubator, the culture medium was discarded, and the cells were washed twice with precooled phosphate-buffered saline (PBS). Then the PBS was discarded, the cells were lysed on ice with immunoprecipitation (IP) lysis solution, and 5× loading buffer was then added. The protein samples were placed in boiling water for 10 min, cooled on ice, and then resolved by sodium dodecyl-sulfate polyacrylamide gel electrophoresis wells. Next, 80 V was applied for 30 min until the bromophenol blue reached the dividing line between the concentrated and separated gum. The voltage was then adjusted to 120 V, and the electrophoresis time was determined based on the molecular weight of the detected proteins. Generally, the electrophoresis was stopped when the bromophenol blue reached the bottom of the gel. After electrophoresis, proteins were electrotransferred to NC membranes under a 300-mA constant current; the transfer time depended on the molecular weight. Immediately after the transfer, the protein membranes were blocked in 5% skim milk in Tris-buffered saline with Tween (TBST) at room temperature for 2 h. After washing the membrane with TBST, the membranes were incubated with primary antibodies overnight at 4 ℃ with slow shaking. After three 10-min washes with TBST, the membranes were incubated with the appropriate secondary antibody at room temperature for 2 h. Then the membranes were washed with TBST solution three times for 10 min each. The Tanon 4600 Chemiluminescence/Fluorescence Imaging System (Tanon, Shanghai, China) was used to develop the film.
Caspase 3/7 activity assays
The Promega Caspase-Glo 3/7 Assay Kit (Promega, Fitchburg, WI, USA) was used to detect caspase 3/7 activity in the cells. In brief, the cells infected with sh-control or sh-MBNL2 lentivirus were placed into 96-well plates and incubated for 5 days. The cells were collected, and 100 µL Caspase-Glo reaction solution was added to the cell suspension (1×104 cells/well). After shaking at 300–500 rpm for 30 min, the cell suspension was incubated at room temperature for 2 h. Finally, the signal intensity was measured using a microplate reader.
Cell Counting Kit-8 (CCK-8) assay
In the exponential growth phase, the cells were digested by pancreatin and the number of the cells was counted. Next, 3 mL of cell suspension was prepared at a density of 1.5×104 cells/mL. Using four 96-well plates, three wells in each 96-well plate were inoculated with 100 µL cell suspension per well, and the cells were pre-incubated in an incubator (at 37 ℃ with 5% CO2). Using one of the 96-well plates, 10 µL of CCK-8 solution (Sigma, St. Louis, MO, USA) was added to each well, cells were incubated in an incubator at 37 ℃ for 4 h, and the absorbance value was measured at 450 nm using a microplate reader. The same procedure was conducted on the remaining plates after culturing for 1, 2, 3, 4, and 5 days.
Transwell assays for migration
Transwell assays (Corning, Somerville, MA, USA) were used to assess the migration and invasion ability of the H520 cells. The cell suspension was prepared at a density of 2.5×105 cells/mL using serum-free medium. Next, 200 µL of serum-free cell suspension was added to the upper chamber, and 600 µL of DMEM containing 10% FBS was added to the lower chamber. After 48 h of incubation in the incubator, the chamber was removed. Then the cells were washed with PBS, fixed on the lower side of the inserted membrane with methanol for 30 min, and crystallized by violet staining. The inserted membrane was washed in PBS for a few seconds to remove any excess dye. The upper side of the membrane was wiped with a cotton swab to remove the gel. The inserted membrane was dried, after which photographs were taken and the migratory cells were counted with a microscope (Olympus, Kyoto, Japan). In the cell invasion test, the upper chamber was pre-coated with Matrigel (BD Biosciences, Franklin Lakes, NJ, USA), but the other steps were the same as those detailed for the migration test above.
Statistical analyses
All of analyses in this study were performed using GraphPad Prism (version 9.0). The Wilcoxon rank-sum test and the unpaired t-test were used to determine the differences between two groups of numerical variables, respectively. The Spearman test was used for the correlation analysis. P<0.05 was considered statistically significant.
Results
Differential gene expression analysis and pathway analysis for LUSC
By comparing the cancer tissues and paracancerous tissues, we identified 5,963 DEGs, which were distributed on each chromosome (Figure 1A). Among them, 4,039 genes were downregulated, and 1,924 genes were upregulated. Of the 5,963 DEG, there were 4,649 gene-encoding proteins, 730 lncRNAs, and 584 other genes (Figure 1B). To explore how these genes affect body functions through pathways, we performed GSEA and found a total of four differentially enriched pathways. The results showed that the p53 pathway (Figure 2A), cell cycle pathway (Figure 2B), and mismatch repair (MMR) pathway (Figure 2C) were activated, whereas the mitogen activated protein kinase (MAPK) pathway (Figure 2D) was inhibited.
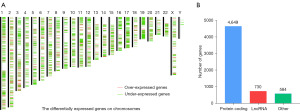
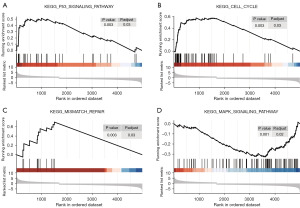
ceRNA network construction
As we found the presence of the lncRNAs and protein-coding genes in DEGs, we constructed ceRNA networks. We predicted the miRNA of the lncRNAs and coding genes using the miRTarBase, and then constructed the ceRNA networks based on their targeting relationships and gene expression. The two networks were centered on the lncRNAs MAGI2-AS3 (Figure 3A) and long intergenic non-protein coding RNA 1089 (LINC01089) (Figure 3B). MAGI2-AS3 targets three miRNAs (i.e., miR-374a/b-5p, and miR-15b-5p), and the miRNAs can bind to five mRNAs (i.e., MBNL2, ATP5L, FAM103A1, MDH1, STXBP1), whereas LINC01089 targets two miRNAs (i.e., miR-3187-3p and miR-27a-3p), and the miRNAs can bind to six mRNAs (ZFP36L2, APBB2, PDLIM3, MYADM, PHF5A, and SLC26A9).
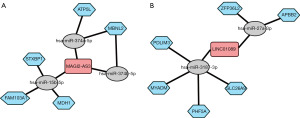
Prognostic analyses
Subsequently, we performed prognostic analysis of the genes found by ceRNA. The results showed that the overall survival rates of the APBB2 (Figure 4A), ZFP36L2 (Figure 4B), MYADM (Figure 4C), PDLIM3 (Figure 4D), SLC26A9 (Figure 4E), and STXBP1 (Figure 4F) of the low-expression group were better than those of the high-expression group. The results showed that the overall survival rates of the ATP5L (Figure 5A), FAM103A1 (Figure 5B), MAGI2-AS3 (Figure 5C), MBNL2 (Figure 5D), MDH1 (Figure 5E), LINC01089 (Figure 5F), and PHF5A (Figure 5G) of the high-expression group were better than those of the low-expression group. These samples were grouped based on the median expression value of the population.
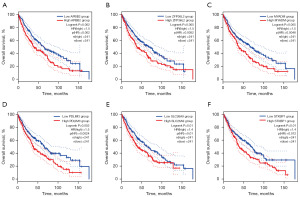
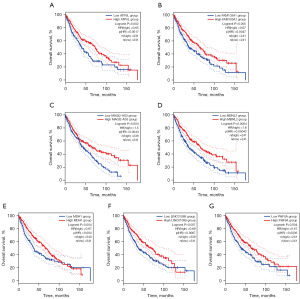
Prognosis signature construction
To further verify the relationships among the ceRNA network genes, we performed a correlation analysis of the expression profiles of the included genes, and found that one lncRNA-mRNA pair (MAGI-AS3-MBNL2) was strongly correlated. The results showed that the transcriptome levels of the two genes were highly correlated, and had a correlation coefficient of R=0.51 (Figure 6A). Subsequently, we used these two genes to form a signature and their median values (the sum of the expression values of two genes in 482 patients) were used to group the population. The results showed that the overall survival rate of the high-expression signature group was higher than that of the low-expression group (Figure 6B).
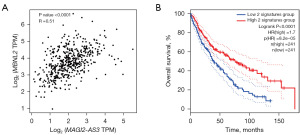
In vitro experiments
To verify the targeting relationship between MAGI2-AS3 or MBNL2 and miR-374a-5p, we performed dual luciferase assays. The results showed that the fluorescence activity was significantly decreased in both the MBNL2-WT (Figure 7A) and MAGI2-AS3-WT groups (Figure 7B), which indicated a targeting relationship among MAGI2-ASA3, MBNL2, and miR-374a-5p. The inhibition of MAGI2-AS3 expression suppressed the upregulation of miR-374a-5p expression, and suppressed MBNL2 expression, confirming the regulatory relationship among MAGI2-AS3, miR-374a-5p, MBNL2 (Figure 7C). Promoting MBNL2 expression by Lenti- MBNL2 inhibited cancer cell expansion (Figure 7D), and inhibiting MBNL2 expression promoted cancer cell growth (Figure 7E). In addition, promoting MBNL2 expression inhibited the migration ability of cancer cells (Figure 7F), while inhibiting MBNL2 expression enhanced migration ability of cancer cells (Figure 7G). Promoting MBNL2 expression enhanced caspase 3/7 activity (Figure 7H), while inhibiting MBNL2 expression decreased the caspase 3/7 activity (Figure 7I).
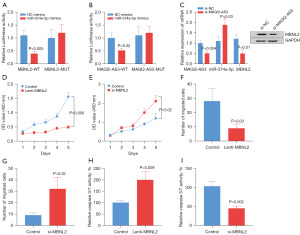
Discussion
By analyzing LUSC tissues and paraneoplastic tissues, 5,963 DEGs were identified. These DEGs were examined by a GSEA, and four differential enrichment pathways were found. Among these pathways, the p53 pathway, cell cycle pathway, and MMR pathway were activated, while the MAPK pathway was inhibited. By constructing two ceRNA networks centered on MAGI2-AS3 and LINC01089, respectively, we found the correlated mRNAs, and by analyzing the prognosis of these genes, we found a targeting relationship among MAGI2-ASA3, MBNL2, and miR-374a/b-5p.
A study suggests that the p53 pathway, cell cycle pathway, and MMR pathway are correlated with the development of NSCLC. The tumor protein gene p53 (TP53) is located on chromosome 17p13.1, and is used to encode the tumor suppressor protein p53 (22). It is the most common mutant gene in humans, and more than 50% of human cancers show a change in p53 signaling (23). Functionally, p53 binds directly to chromatin and plays an important role in regulating the cell cycle, apoptosis, and autophagy, and repairing damaged DNA (24). However, when mutations occur, the loss of these functions can lead to abnormal cell proliferation and promote cancer development (25). TP53 mutations are mainly missense mutations, and are more prevalent in LUSC than LUAD (5). Mutated p53 protein forms aggregates, and cytoplasmic p53 aggregates are associated with poor prognosis in human ovarian cancer (26). Analysis of patient derived tumor organoids with cytoplasmic p53 aggregates established from patients with LUSC showed that eliminating cytoplasmic p53 aggregates inhibited cell proliferation, thereby prolonging patient prognosis. In addition, experiments in LUSC cell lines have shown that cytoplasmic p53 aggregates lead to cisplatin resistance (26).
The MMR pathway is a highly evolutionarily conserved mechanism responsible for correcting base mismatches (e.g., C or G as opposed to T or A) and insertion/deletion loops (appearing in repetitive sequences such as AAAAAAA… or CACACACA…). The nucleotide mismatches are resolved by the MMR pathway, and patients with mismatch repair deficient/microsatellite instability-high status (dMMR/MSI-H) show high response rates to treatment with immune checkpoint inhibitors (27-29). However, rare dMMR and MSI-H are observed in NSCLC (27,30). A study by scholars have shown that DNA mismatch repair gene polymorphism is associated with hematological and gastrointestinal toxicity, and may be considered a predictive factor for pre-treatment assessment in lung cancer patients (31).
Cell cycle progression plays a crucial role in cell proliferation, and its alteration has been recognized as one of the hallmarks of cancer (32). Oshi et al. (33) studied the cell cycle of 4,626 breast cancer tumors, and found an association between G2M checkpoint activity in cell-cycle control and cancer aggressiveness, metastasis, and treatment response. The authors also found that breast cancer tumors with genetically active G2M cells were more aggressive and more likely to metastasize, which is the leading cause of eventual death of breast cancer patients. Chen et al. (34) found that protein phosphatase 1 regulatory subunit 12B (PPP1R12B) plays a regulatory role in esophageal cancer by inhibiting the cell cycle pathway, and the overexpression of ADAMTS9-AS2 regulates tumor formation in esophageal cancer cells in vivo through the miR-196b-5p/PPP1R12B/cell-cycle signaling pathway axis. These findings suggests that ADAMTS9-AS2 downregulates PPP1R12B by adsorbing miR-196b-5p, thereby regulating the cell cycle signaling pathway and inhibiting the malignant progression of esophageal cancer. Moreover, scholars have reported an activator ZK53, which has therapeutic effects on lung squamous cell carcinoma in vivo. ZK53 treatment leads to a decrease in electron transport chain in a caseinolytic protease P (ClpP) dependent manner, resulting in reduced oxidative phosphorylation and adenosine triphosphate (ATP) production in lung squamous cell carcinoma cells, ultimately triggering cell cycle arrest and leading to cell death (35).
LncRNAs are non-coding transcripts longer than 200 nucleotides (36). They can be divided into genes (exons, introns, and overlapping genes) and intergenic lncRNAs (37). Numerous studies have shown that lncRNAs act as important regulators of a variety of biological processes including cell proliferation, differentiation, migration, invasion, and apoptosis. LncRNA dysregulation has been observed in various tumor tissues (38,39). Li et al. (40) found that LINC01089, which is a highly conserved lncRNA, inhibits cell proliferation and leads to breast cancer metastasis, and that LINC01089 expression is significantly downregulated in NSCLC tissues and cell lines compared to adjacent normal tissues and cells. In vitro cell proliferation and migration analyses (40) of LINC01089 in NSCLC and mouse tumors revealed that a very small amount of LINC01089 promotes NSCLC cell proliferation and migration, thus promoting tumor formation.
Members of the MAPK signaling pathway, including p38, ERK1/2, and JNK, regulate all key stages of cell growth, including proliferation, differentiation, and apoptosis. It is reported that the disorder of MAPK pathway occurs in several types of tumors, including human epithelial cancer, prostate cancer, renal cell carcinoma, hepatocellular carcinoma, glioma and breast cancer (18). Recently, scholars have confirmed that Licochalcone A can induce cell cycle arrest and apoptosis in LUSC by inhibiting the MAPK signaling pathway and FBXO5 expression (41).
Studies (42,43) have shown that MAGI2-AS3 acts as a tumor suppressor in bladder cancer, breast cancer and hepatocellular carcinoma. Previous studies (44,45) have also shown that MAGI2-AS3 is downregulated in NSCLC samples, including LUAD and LUSC. He et al. (46) identified the downregulation of MAGI2-AS3 in LUSC samples based on TCGA data from GEPIA and validated this result in LUSC samples by qPCR, which showed that MAGI2-AS3 is involved in LUSC. In addition, the authors showed that MAGI2-AS3 inhibits cell proliferation, cell migration and invasion, induces cell apoptosis in vitro, which suggests that the overexpression of MAGI2-AS3 attenuates tumor cell genesis and metastasis in vivo. These findings are consistent with the results of the current study. Other researchers have focused on stem cell use in tumor therapy and revealed that bone marrow mesenchymal stem cell-derived extracellular vesicles inhibit colorectal cancer progression by transporting MAGI2-AS3 (47).
MiRNAs are single-stranded non-coding small RNA molecules that range from 21 to 25 nucleotides, and play a key role in multiple physiological and pathological processes in NSCLC. Aberrant miRNA expression in tissues, blood, and blood exosomes can be used as potential diagnostic, therapeutic, and prognostic biomarkers for NSCLC patients (46,48). Zhang et al. (49) found that miR-3187-3p is significantly upregulated in NSCLC, and that higher miR-3187-3p expression is correlated with lower survival in NSCLC. MiR-3187-3p overexpression in normal lung epithelial cells is followed by a significant increase in cell proliferation, migration and invasion, which also suggests that miR-3187-3p acts as an oncogene in NSCLC. Additionally, miR-3187-3p overexpression promotes the proliferation, migration and invasion of A549 and SK-MES-1, and reverses the role of LINC01089, which suggests that LINC01089 and miR-3187-3p constitute a regulatory axis in NSCLC (49).
LncRNAs interact with miRNAs to regulate the level of target genes, and usually act as ceRNAs in the cytoplasm of cells. Studies have shown that MAGI2-AS3 is mostly distributed in the cytoplasm of LUSC cells (43,50); therefore, it is speculated that MAGI2-AS3 may also function in this mode. MiR-374a/b-5p has been proven to promote tumor development in both breast cancer and hepatocellular carcinoma (43,50). He et al. (46) showed that MAGI2-AS3 can negatively regulate miR-374a/b-5p levels, while MAGI2-AS3 shows no changes in the context of miR-374a/b-5p overexpression, which suggests a binding between MAGI2-AS3 and miR-374a/b-5p.
The authors (46) selected CADM2 as a target gene for miR-374a/b-5p, and verified that miR-374a/b-5p negatively regulates CADM2 mRNA and protein levels by binding to CAMD2 mRNA (46). Furthermore, subsequent rescue assays revealed that the effect of miR-374a/b-5p amplification on cell proliferation, apoptosis resistance, migration, and invasion is blocked by the transfection of pcDNA3.1/CADM2. This suggests that lncRNA MAGI2-AS3 inhibits multiple cellular processes in LUSC cells by regulating the miR-374a/b-5p/CADM2 axis.
RNA-binding proteins (RBPs) have been found to be dysregulated in a variety of cancers and are associated with tumorigenesis and progression (51-53). Li et al. (54) used univariate Cox regression analysis, least absolute shrinkage and selection operator regression analysis, Kaplan-Meier test, and multivariate Cox regression analysis to screen for the central RBPs associated with prognosis. The results revealed that nine genes, including MBNL2, RSRC1 and TRMU, are highly expressed, and these genes are associated with a good prognosis in patients with LUSC, which is consistent with the findings of this study.
This study only included one cell line for experimentation, so there are certain limitations with the experimental results. In addition, we were unable to explore the relationship between MAGI2-AS3 and MBNL2 and regulatory pathways in this study. In the future, more cell lines will be included and further validation will be performed with animal models.
Conclusions
This study analyzed LUSC tissue and adjacent tissue and identified 5,963 DEGs. By using GSEA enrichment analysis, four differentially enriched pathways were identified. Taking the four pathways as examples, it was found that the p53 pathway, cell cycle pathway, and MMR pathway were activated, while the MAPK pathway was inhibited. By constructing two ceRNA networks centered around MAGI2-AS3 and LINC01089, the mRNAs associated with them were identified. Prognostic analysis of these genes revealed a mechanistic relationship among MAGI2-ASA3, MBNL2, and miR-374a/b-5p. Previous reports predicted the prognosis of patients through a single gene. In this article, a combination of lncRNA and mRNA with potential regulatory relationships is used to predict the prognosis of patients, which can improve the accuracy of prediction. In addition, research on these genes in LUSC is still scarce and limited at the molecular level. The discovery of the relationship between lncRNA-mRNA and patient prognosis in this article can provide some guidance for future patient prognostic classification.
Acknowledgments
None.
Footnote
Reporting Checklist: The authors have completed the MDAR reporting checklist. Available at https://tcr.amegroups.com/article/view/10.21037/tcr-2024-2443/rc
Data Sharing Statement: Available at https://tcr.amegroups.com/article/view/10.21037/tcr-2024-2443/dss
Peer Review File: Available at https://tcr.amegroups.com/article/view/10.21037/tcr-2024-2443/prf
Funding: None.
Conflicts of Interest: All authors have completed the ICMJE uniform disclosure form (available at https://tcr.amegroups.com/article/view/10.21037/tcr-2024-2443/coif). A.R. reported stock by IQVIA Holdings Inc. Nobuhiko Seki obtained research grants from Eli Lilly, Chugai Pharmaceutical, Taiho Pharmaceutical, Pfizer, Ono Pharmaceutical, Nippon Kayaku, Takeda Pharmaceutical, Eisai, Shionogi, Daiichi Sankyo, and Boehringer Ingelheim and received speaking honoraria from Eli Lilly, AstraZeneca, MSD, Chugai Pharmaceutical, Taiho Pharmaceutical, Pfizer, Ono Pharmaceutical, Nippon Kayaku, Takeda Pharmaceutical, Daiichi Sankyo, Boehringer Ingelheim, Novartis, Kyowa Kirin, and Bristol Myers Squibb. The other authors have no conflicts of interest to declare.
Ethical Statement: The authors are accountable for all aspects of the work in ensuring that questions related to the accuracy or integrity of any part of the work are appropriately investigated and resolved. The study was conducted in accordance with the Declaration of Helsinki (as revised in 2013).
Open Access Statement: This is an Open Access article distributed in accordance with the Creative Commons Attribution-NonCommercial-NoDerivs 4.0 International License (CC BY-NC-ND 4.0), which permits the non-commercial replication and distribution of the article with the strict proviso that no changes or edits are made and the original work is properly cited (including links to both the formal publication through the relevant DOI and the license). See: https://creativecommons.org/licenses/by-nc-nd/4.0/.
References
- Bray F, Laversanne M, Sung H, et al. Global cancer statistics 2022: GLOBOCAN estimates of incidence and mortality worldwide for 36 cancers in 185 countries. CA Cancer J Clin 2024;74:229-63. [Crossref] [PubMed]
- Siegel RL, Giaquinto AN, Jemal A. Cancer statistics, 2024. CA Cancer J Clin 2024;74:12-49. [Crossref] [PubMed]
- Giaccone G, He Y. Current knowledge of small cell lung cancer transformation from non-small cell lung cancer. Semin Cancer Biol 2023;94:1-10. [Crossref] [PubMed]
- Gao M, Kong W, Huang Z, et al. Identification of Key Genes Related to Lung Squamous Cell Carcinoma Using Bioinformatics Analysis. Int J Mol Sci 2020;21:2994. [Crossref] [PubMed]
- Xu F, Lin H, He P, et al. A TP53-associated gene signature for prediction of prognosis and therapeutic responses in lung squamous cell carcinoma. Oncoimmunology 2020;9:1731943. [Crossref] [PubMed]
- Travis WD, Brambilla E, Nicholson AG, et al. The 2015 World Health Organization Classification of Lung Tumors: Impact of Genetic, Clinical and Radiologic Advances Since the 2004 Classification. J Thorac Oncol 2015;10:1243-60. [Crossref] [PubMed]
- Qian L, Lin L, Du Y, et al. MicroRNA-588 suppresses tumor cell migration and invasion by targeting GRN in lung squamous cell carcinoma. Mol Med Rep 2016;14:3021-8. [Crossref] [PubMed]
- Barbone F, Bovenzi M, Cavallieri F, et al. Cigarette smoking and histologic type of lung cancer in men. Chest 1997;112:1474-9. [Crossref] [PubMed]
- Rivera GA, Wakelee H. Lung Cancer in Never Smokers. Adv Exp Med Biol 2016;893:43-57. [Crossref] [PubMed]
- Osmani L, Askin F, Gabrielson E, et al. Current WHO guidelines and the critical role of immunohistochemical markers in the subclassification of non-small cell lung carcinoma (NSCLC): Moving from targeted therapy to immunotherapy. Semin Cancer Biol 2018;52:103-9. [Crossref] [PubMed]
- Molina JR, Yang P, Cassivi SD, et al. Non-small cell lung cancer: epidemiology, risk factors, treatment, and survivorship. Mayo Clin Proc 2008;83:584-94. [Crossref] [PubMed]
- Wei Y, Zhang X. Transcriptome analysis of distinct long non-coding RNA transcriptional fingerprints in lung adenocarcinoma and squamous cell carcinoma. Tumour Biol 2016; Epub ahead of print. [Crossref]
- Miyoshi A, Kanao S, Naoi H, et al. Investigation of the clinical features of lower uterine segment carcinoma: association with advanced stage disease and indication of poorer prognosis. Arch Gynecol Obstet 2018;297:193-8. [Crossref] [PubMed]
- Cheung CHY, Juan HF. Quantitative proteomics in lung cancer. J Biomed Sci 2017;24:37. [Crossref] [PubMed]
- Zhang Y, Yang Q, Wang S. MicroRNAs: a new key in lung cancer. Cancer Chemother Pharmacol 2014;74:1105-11. [Crossref] [PubMed]
- Matsubara T, Uchi H, Haratake N, et al. Acute Generalized Exanthematous Pustulosis Caused by the Combination of Pembrolizumab Plus Chemotherapy in a Patient With Squamous-Cell Carcinoma. Clin Lung Cancer 2020;21:e54-6. [Crossref] [PubMed]
- Liu TT, Li R, Huo C, et al. Identification of CDK2-Related Immune Forecast Model and ceRNA in Lung Adenocarcinoma, a Pan-Cancer Analysis. Front Cell Dev Biol 2021;9:682002. [Crossref] [PubMed]
- Wu S, Xu J, Zhang M, et al. Analysis of Genetic Variants and the ceRNA Network of miR-9 in Non-Small Cell Lung Cancer. DNA Cell Biol 2022;41:142-50. [Crossref] [PubMed]
- Fatica A, Bozzoni I. Long non-coding RNAs: new players in cell differentiation and development. Nat Rev Genet 2014;15:7-21. [Crossref] [PubMed]
- Chen Y, Li C, Pan Y, et al. The Emerging Role and Promise of Long Noncoding RNAs in Lung Cancer Treatment. Cell Physiol Biochem 2016;38:2194-206. [Crossref] [PubMed]
- Hua Q, Jin M, Mi B, et al. LINC01123, a c-Myc-activated long non-coding RNA, promotes proliferation and aerobic glycolysis of non-small cell lung cancer through miR-199a-5p/c-Myc axis. J Hematol Oncol 2019;12:91. [Crossref] [PubMed]
- Jovanović KK, Escure G, Demonchy J, et al. Deregulation and Targeting of TP53 Pathway in Multiple Myeloma. Front Oncol 2018;8:665. [Crossref] [PubMed]
- Ingaramo MC, Sánchez JA, Dekanty A. Regulation and function of p53: A perspective from Drosophila studies. Mech Dev 2018;154:82-90. [Crossref] [PubMed]
- Mogi A, Kuwano H. TP53 mutations in nonsmall cell lung cancer. J Biomed Biotechnol 2011;2011:583929. [Crossref] [PubMed]
- Huszno J, Grzybowska E. TP53 mutations and SNPs as prognostic and predictive factors in patients with breast cancer. Oncol Lett 2018;16:34-40. [Crossref] [PubMed]
- Nishitsuji K, Mito R, Ikezaki M, et al. Impacts of cytoplasmic p53 aggregates on the prognosis and the transcriptome in lung squamous cell carcinoma. Cancer Sci 2024;115:2947-60. [Crossref] [PubMed]
- Akhoundova D, Francica P, Rottenberg S, et al. DNA Damage Response and Mismatch Repair Gene Defects in Advanced and Metastatic Prostate Cancer. Adv Anat Pathol 2024;31:61-9. [Crossref] [PubMed]
- Incorvaia L, Bazan Russo TD, Gristina V, et al. The intersection of homologous recombination (HR) and mismatch repair (MMR) pathways in DNA repair-defective tumors. NPJ Precis Oncol 2024;8:190. [Crossref] [PubMed]
- Riedinger CJ, Esnakula A, Haight PJ, et al. Characterization of mismatch-repair/microsatellite instability-discordant endometrial cancers. Cancer 2024;130:385-99. [Crossref] [PubMed]
- Li N, Wan Z, Lu D, et al. Long-term benefit of immunotherapy in a patient with squamous lung cancer exhibiting mismatch repair deficient/high microsatellite instability/high tumor mutational burden: A case report and literature review. Front Immunol 2022;13:1088683. [Crossref] [PubMed]
- Singh S, Singh N, Sharma S. Genetic polymorphisms in the mismatch repair pathway (MMR) genes contribute to hematological and gastrointestinal toxicity in North Indian lung cancer patients treated with platinum-based chemotherapy. J Biochem Mol Toxicol 2022;36:e23183. [Crossref] [PubMed]
- Hanahan D, Weinberg RA. The hallmarks of cancer. Cell 2000;100:57-70. [Crossref] [PubMed]
- Oshi M, Takahashi H, Tokumaru Y, et al. G2M Cell Cycle Pathway Score as a Prognostic Biomarker of Metastasis in Estrogen Receptor (ER)-Positive Breast Cancer. Int J Mol Sci 2020;21:2921. [Crossref] [PubMed]
- Chen Z, Tang W, Ye W, et al. ADAMTS9-AS2 regulates PPP1R12B by adsorbing miR-196b-5p and affects cell cycle-related signaling pathways inhibiting the malignant process of esophageal cancer. Cell Cycle 2022;21:1710-25. [Crossref] [PubMed]
- Zhou LL, Zhang T, Xue Y, et al. Selective activator of human ClpP triggers cell cycle arrest to inhibit lung squamous cell carcinoma. Nat Commun 2023;14:7069. [Crossref] [PubMed]
- Xing Z, Park PK, Lin C, et al. LncRNA BCAR4 wires up signaling transduction in breast cancer. RNA Biol 2015;12:681-9. [Crossref] [PubMed]
- Xu J, Bai J, Zhang X, et al. A comprehensive overview of lncRNA annotation resources. Brief Bioinform 2017;18:236-49. [Crossref] [PubMed]
- Thin KZ, Liu X, Feng X, et al. LncRNA-DANCR: A valuable cancer related long non-coding RNA for human cancers. Pathol Res Pract 2018;214:801-5. [Crossref] [PubMed]
- Luo D, Deng B, Weng M, et al. A prognostic 4-lncRNA expression signature for lung squamous cell carcinoma. Artif Cells Nanomed Biotechnol 2018;46:1207-14. [Crossref] [PubMed]
- Li X, Lv F, Li F, et al. LINC01089 Inhibits Tumorigenesis and Epithelial-Mesenchymal Transition of Non-small Cell Lung Cancer via the miR-27a/SFRP1/Wnt/β-catenin Axis. Front Oncol 2020;10:532581. [Crossref] [PubMed]
- Fan X, Guan G, Wang J, et al. Licochalcone A induces cell cycle arrest and apoptosis via suppressing MAPK signaling pathway and the expression of FBXO5 in lung squamous cell cancer. Oncol Rep 2023;50:214. [Crossref] [PubMed]
- Wang F, Zu Y, Zhu S, et al. Long noncoding RNA MAGI2-AS3 regulates CCDC19 expression by sponging miR-15b-5p and suppresses bladder cancer progression. Biochem Biophys Res Commun 2018;507:231-5. [Crossref] [PubMed]
- Yin Z, Ma T, Yan J, et al. LncRNA MAGI2-AS3 inhibits hepatocellular carcinoma cell proliferation and migration by targeting the miR-374b-5p/SMG1 signaling pathway. J Cell Physiol 2019;234:18825-36. [Crossref] [PubMed]
- Luo CL, Xu ZG, Chen H, et al. LncRNAs and EGFRvIII sequestered in TEPs enable blood-based NSCLC diagnosis. Cancer Manag Res 2018;10:1449-59. [Crossref] [PubMed]
- Huang J, Li Y, Lu Z, et al. Analysis of functional hub genes identifies CDC45 as an oncogene in non-small cell lung cancer - a short report. Cell Oncol (Dordr) 2019;42:571-8. [Crossref] [PubMed]
- He J, Zhou X, Li L, et al. Long Noncoding MAGI2-AS3 Suppresses Several Cellular Processes of Lung Squamous Cell Carcinoma Cells by Regulating miR-374a/b-5p/CADM2 Axis. Cancer Manag Res 2020;12:289-302. [Crossref] [PubMed]
- Ma T, Wang M, Wang S, et al. BMSC derived EVs inhibit colorectal Cancer progression by transporting MAGI2-AS3 or something similar. Cell Signal 2024;121:111235. [Crossref] [PubMed]
- Świtlik WZ, Szemraj J. Circulating miRNAs as non-invasive biomarkers for non-small cell lung cancer diagnosis, prognosis and prediction of treatment response. Postepy Hig Med Dosw (Online) 2017;71:649-62. [Crossref] [PubMed]
- Zhang D, Cai X, Cai S, et al. Long Intergenic Non-Protein Coding RNA 01089 Weakens Tumor Proliferation, Migration, and Invasion by Sponging miR-3187-3p in Non-Small Cell Lung Cancer. Cancer Manag Res 2020;12:12151-62. [Crossref] [PubMed]
- Son D, Kim Y, Lim S, et al. miR-374a-5p promotes tumor progression by targeting ARRB1 in triple negative breast cancer. Cancer Lett 2019;454:224-33. [Crossref] [PubMed]
- Chen H, Liu J, Wang H, et al. Inhibition of RNA-Binding Protein Musashi-1 Suppresses Malignant Properties and Reverses Paclitaxel Resistance in Ovarian Carcinoma. J Cancer 2019;10:1580-92. [Crossref] [PubMed]
- Cooke A, Schwarzl T, Huppertz I, et al. The RNA-Binding Protein YBX3 Controls Amino Acid Levels by Regulating SLC mRNA Abundance. Cell Rep 2019;27:3097-3106.e5. [Crossref] [PubMed]
- Zhang J, Zheng Z, Wu M, et al. The natural compound neobractatin inhibits tumor metastasis by upregulating the RNA-binding-protein MBNL2. Cell Death Dis 2019;10:554. [Crossref] [PubMed]
- Li W, Li X, Gao LN, et al. Integrated Analysis of the Functions and Prognostic Values of RNA Binding Proteins in Lung Squamous Cell Carcinoma. Front Genet 2020;11:185. [Crossref] [PubMed]
(English Language Editor: L. Huleatt)