Scoparone suppresses proliferation and cell cycle of hepatocellular carcinoma cells via inhibiting AKT/GSK-3β/cyclin D1 signaling pathway
Highlight box
Key findings
• Scoparone inhibits hepatocellular carcinoma (HCC) cell proliferation, invasion, metastasis and cell cycle.
What is known and what is new?
• The underlying function mechanisms of scoparone in HCC have not been clearly elucidated.
• In this study, scoparone inhibited the proliferation, migration and invasion in HCC via decreasing cell cycle-related proteins and matrix metalloproteinase (MMP)-2, MMP-9, and depressing AKT/GSK-3β/cyclin D1 pathway, which can be partially rescued by AKT activator SC79.
What is the implication, and what should change now?
• Our findings provide insights on new molecular mechanisms for the antitumor effects of scoparone and novel strategies for the treatment of HCC.
Introduction
Hepatocellular carcinoma (HCC) is a highly lethal malignancy worldwide with approximately 865,269 new cases and 757,948 deaths reported in 2022 (1). More than half of the new cases globally occur in China (1). Despite various available treatments, such as surgical resection, radiotherapy, chemotherapy, and traditional Chinese medicine, the overall prognosis for HCC patients remains poor (2). Chemotherapy, a primary treatment method for advanced HCC, is limited in effectiveness and often associated with severe side effects. Therefore, there is an urgent need to identify new targets and molecular pathways for the development of drugs to treat HCC (3-5).
Scoparone, extracted primarily from Artemisia Capillaris Thub, is a simple natural coumarin derivative (6). Initially, it was commonly used to treat liver dysfunction, bile stasis, and jaundice (7-9). Studies have reported that scoparone can modulate the Toll-like receptor 4 (TLR4)/nuclear factor-kappa B (NF-κB) signaling pathway to alleviate the immune response induced by NASH and lipopolysaccharides (LPS) in macrophages, effectively improving hepatic steatosis and inflammation (10,11). Additionally, scoparone exerts hepatoprotective effects by ameliorating the pathological features of acute liver failure via inhibiting of the TLR signaling pathway (12). In non-alcoholic fatty liver disease (NAFLD), scoparone exerts hepatoprotective and choleretic effects by inhibiting obesity-related adipose tissue inflammation, countering cytokine-mediated cytotoxicity, improving insulin resistance, and reducing oxidative stress, thereby inhibiting the progression of NAFLD (7).
Moreover, scoparone was found to exert antitumor effects on several cancers (13-16). A previous study had shown that scoparone inhibits cell proliferation, increases cells apoptosis and decreases metastasis-related protein matrix metalloproteinase (MMP)-9 to inhibit metastasis and invasion of pancreatic cancer cells (13). Furthermore, scoparone activates the ROS/JNK/SP1/ACSL4 axis and triggers ferroptosis in non-small cell lung cancer (NSCLC) cells (14). Additionally, scoparone inhibits the growth of colorectal cancer (CRC) (15) and prostate cancer cells (16) via inducing mechanistic target of rapamycin (mTOR)-related autophagy and directly targeting STAT3 transcriptional activity to exert antitumor, respectively. However, the functions of scoparone in HCC are not fully elucidated.
In this study, scoparone inhibited the proliferation, migration and invasion via decreasing cell cycle-related proteins and MMP-2, MMP-9, and depressing AKT/GSK-3β/cyclin D1 pathway, which can be partially rescued by AKT activator SC79. We present this article in accordance with the MDAR reporting checklist (available at https://tcr.amegroups.com/article/view/10.21037/tcr-24-1771/rc).
Methods
Cell culture
The human hepatoma cell lines MHCC-97L, HCCC-9810 and human normal liver cell line L-02 were procured from Zhong Qiao Xin Zhou Biotechnology Co., Ltd. (Shanghai, China). The L-02 and HCCC-9810 cells were cultured in RPMI-1640 medium (Gibco, Waltham, MA, USA), while the MHCC-97L cells were grown in DMEM medium (Gibco) supplemented with 10% fetal bovine serum (FBS; Gibco). All cells were maintained in a 5% CO2 incubator at 37 ℃.
Reagents and antibodies
The following antibodies were used in Western blot: anti-CDK2 [1:1,000, #18048, Cell Signaling Technology (CST), Danfoss, MA, USA], anti-CDK4 (1:1,000, #12790, CST), anti-cyclin D1 (1:1,000, #2922, CST), anti-AKT (1:1,000, #9272, CST), anti-p-AKT (Ser473) (1:1,000, #9271, CST), anti-MMP-2 (1:1,000, #4022, CST), anti-MMP-9 (1:1,000, #3852, CST), anti-p-GSK-3β (Ser9) (1:1,000, #9336, CST), anti-GSK-3β (1:1,000, 22104-1-AP, Proteintech, Wuhan, China), and anti-β-actin (1:1,000, 66009-1-Ig, Proteintech). Scoparone (catalogue number PHL89787) and dimethyl sulfoxide (DMSO; catalogue number S-002-M) were purchased from Sigma (Livonia, MI, USA).
Cell viability assay
The L-02, MHCC-97L, and HCCC-9810 cells were cultured in 96-well plates with a density of 5,000 cells per well. Following a 12-hour incubation period, the medium was replaced with varying concentrations of scoparone (0, 50, 100, 150, 200, 250, 300, 350 µg/mL) for two distinct durations, 24 and 48 hours. Subsequently, cell viability was assessed utilizing the cell counting kit-8 (CCK-8) assay kit (Shanghai Dongren Chemical Technology Co., Ltd., Shanghai, China) in accordance with the provided instructions. The optical density (OD) value was then measured at a wavelength of 450 nm and the half-maximal inhibitory concentration (IC50) value was subsequently calculated.
Colony formation assay
Cells were seeded at 400 cells per well in a six-well plate and incubated for 24 hours. Subsequently, they were treated with different concentrations of scoparone. The resulting cell colonies were stained with 0.01% crystal violet and then counted. These experiments were conducted in triplicate.
5-ethynyl-2'-deoxyuridine (EdU) proliferation assay
MHCC-97L and HCCC-9810 cells were incubated with a EdU-labeling solution for 2 hours at 37 ℃ and then fixed with 4% paraformaldehyde for 15 minutes. After permeabilization, the cells were treated with the reaction solution for 30 minutes. The cell nuclei were subsequently stained with 1× Hoechst 33342 for 10 minutes and photographed using a fluorescent microscope. Finally, the cell proliferation rate was calculated.
Wound healing assay
MHCC-97L and HCCC-9810 cells were pretreated with 0 (control), 100, and 200 µmol/L scoparone for 24 hours. A vertical scratch was made in the cell monolayer using a 200-µL sterile pipette tip. The cells were then washed with phosphate-buffered saline (PBS), and serum-free culture medium was added. Images of the wound area were captured at 0 and 24 hours using a microscope.
Transwell assay
MHCC-97L and HCCC-9810 cells were pretreated with 0, 100, and 200 µmol/L scoparone for 24 hours and then resuspended in serum-free medium. A 100-µL aliquot of the cell suspension was added to the upper chamber of the cell culture insert, while 600 µL of medium containing 15% FBS was added to the lower chamber. The setup was incubated in a 5% CO2 incubator at 37 ℃ for 24 hours. The membrane of the upper chamber was fixed with 4% paraformaldehyde for 30 minutes and then stained with 0.2% crystal violet at 25 ℃ for 15 minutes. Four fields of view (200× magnification) were randomly selected and imaged using a microscope.
Cell cycle by flow cytometry
According to the instructions, cell cycle analysis was conducted using the Cell Cycle Staining Kit (MultiSciences Biotech Co., Ltd., Hangzhou, China) and flow cytometry. MHCC-97L and HCCC-9810 cells were pretreated with 0, 50, 100, and 200 µmol/L scoparone for 48 hours. After digestion, the cells were resuspended in cold PBS and fixed with 70% ethanol overnight at −20 ℃. Subsequently, 1 mL DNA staining solution and 10 of permeabilization solution were added to each flow cytometry tube and incubated in the dark for 30 minutes. The cell cycle was then measured using a flow cytometer.
RNA sequencing (RNA-seq)
Total RNA extraction from both MHCC-97L and HCCC-9810 cells, including control and scoparone-treated groups, was performed using Trizol reagent (Sinopharm Chemical Reagent Co., Shanghai, China). The RNA-seq library construction and sequencing were conducted by a specialized company. Differentially expressed genes were identified with an absolute log2[fold change (FC)] ≥1 and a P value <0.05, indicating statistical significance.
Quantitative reverse transcription-polymerase chain reaction (qRT-PCR)
Total RNA was extracted using Trizol reagent (Sinopharm Chemical Reagent Co.), and the RNA was reverse transcribed into complementary DNA (cDNA) using a qRT-PCR kit (Novizan Biotechnology Co., Nanjing, China). Real-time PCR was then performed in a 20 µL reaction mixture containing diluted first-strand cDNA and a primer mix (Shanghai Sangon, Shanghai, China). The PCR primer sequences are listed in Table 1.
Table 1
Name | Forward | Reverse |
---|---|---|
CDK2 | TGAAGATGGACGGAGCTTGTTATCG | CTGGCTTGGTCACATCCTGGAAG |
CDK3 | CCAGCTTTCGTATCTTTCGT | TTCCTGGTCCACTTAGGGAAG |
CDK4 | TGCCAGCCGAAACGATCAAGG | TCCACCACTTGTCACCAGAATGTTC |
CCND1 | GCCCTCGGTGTCCTACTTCAAATG | TCCTCCTCGCACTTCTGTTCCTC |
CCNE1 | GTGTCCTGGATGTTGACTGCCTTG | TCTCTATGTCGCACCACTGATACCC |
CDC25A | TGTGCCGGTATGTGAGAGAG | TGCGGAACTTCTTCAGGTCT |
CDKN1A | GCCCGTGAGCGATGGAACTTC | CCTGCCTCCTCCCAACTCATCC |
β-actin | CATGTACGTTGCTATCCAGGC | AGAGACCCACAGGAGAGGTG |
PCR, polymerase chain reaction.
Western blot
MHCC-97L and HCCC-9810 cells were pretreated with 0, 50, 100, and 200 µmol/L scoparone for 48 hours. Cells were lysed using radioimmunoprecipitation assay (RIPA) lysis buffer (Beyotime Biotech, Shanghai, China), and proteins were separated and transferred to polyvinylidene fluoride (PVDF) membranes (GE Healthcare, Chicago, IL, USA). The membranes were blocked with 5% skimmed milk for 2 hours, then incubated with primary antibodies overnight at 4 ℃. This was followed by incubation with secondary antibodies at room temperature for 2 hours. The membranes were developed using electrochemiluminescence (ECL) reagent (GE Healthcare).
Statistical analysis
Data were presented as the mean ± standard deviation (SD). Statistical analyses were conducted using a t-test for comparisons between two groups or one-way analysis of variance (ANOVA) tests for comparisons among multiple groups, utilizing GraphPad Prism 9.0 software (GraphPad Software, La Jolla, CA, USA). A P value <0.05 was considered statistically significant. All experiments were repeated at least three times.
Results
Scoparone suppresses HCC cell proliferation in vitro
To determine the effective concentrations and cytotoxic effects of scoparone on HCC cells and normal human liver cells in vitro, MHCC-97L, HCCC-9810, and L-02 cells were treated with 0, 50, 100, 150, 200, 250, 300, and 350 µg/mL scoparone for 24 and 48 hours. Cell viability was then measured using the CCK-8 assay. The viability of L-02, MHCC-97L, and HCCC-9810 cells was significantly reduced by scoparone treatment in a dose- and time-dependent manner, with scoparone being significantly less toxic to L-02 cells than to MHCC-97L and HCCC-9810 cells (Figure 1A). The IC50 values of scoparone after 48 hours of treatment were 395.06 µg/mL for L-02 cells, 196.33 µg/mL for MHCC-97L cells, and 205.06 µg/mL for HCCC-9810 cells (Figure 1B). Measurement of cell viability showed that the proliferation of MHCC-97L and HCCC-9810 cells was significantly suppressed at scoparone concentrations higher than 200 µg/mL, while mildly inhibited the proliferation of L-02 cells. Consequently, we selected the concentration of 200 µg/mL for 48 hours for subsequent experiments.
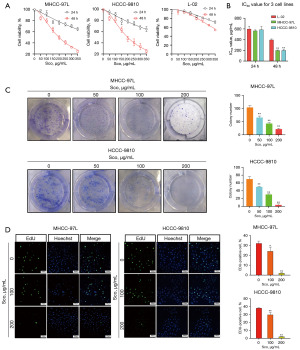
To illustrate long time inhibition of scoparone for cells, colony formation assays were performed. The proliferation ability of MHCC-97L and HCCC-9810 cells was reduced in a dose-dependent manner, compared to the control group after scoparone treatment (Figure 1C). Furthermore, the EdU assay results revealed that scoparone significantly decreased the proliferation abilities of MHCC-97L and HCCC-9810 cells, compared to the control group (Figure 1D). Overall, these findings demonstrate that scoparone significantly suppresses the proliferative capacity of HCC cells.
Scoparone suppresses HCC cell invasion and migration in vitro
Whether scoparone inhibits the invasion and migration of HCC cells is not clear. To demonstrate the effects of scoparone on invasion and migration, wound healing assays were executed. The results showed that the migration ability of MHCC-97L and HCCC-9810 cells was reduced in a dose-dependent manner, compared to the control group after scoparone treatment (Figure 2A). Additionally, transwell assay results revealed that scoparone significantly decreased the invasion abilities of MHCC-97L and HCCC-9810 cells, compared to the control group (Figure 2B). Furthermore, scoparone treatment significantly reduced the expression levels of MMP-2 and MMP-9, which are crucial for tumor cell invasion and metastasis (Figure 2C). These findings clearly demonstrate that scoparone inhibits the invasion and migration of HCC cells.
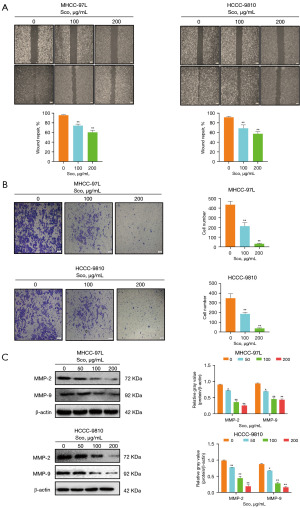
Scoparone induces cell cycle arrest in HCC cells
To investigate the molecular mechanisms by which scoparone inhibits the proliferation of HCC cells, we conducted transcriptome sequencing on two HCC cell lines. Each cell line was divided into control group and treatment group, with the treatment group receiving 200 µg/mL scoparone for 48 hours. Total 373 differentially expressed genes in MHCC-97L cells (124 upregulated and 249 downregulated) and 207 differentially expressed genes in HCCC-9810 cells (24 upregulated and 183 downregulated) were identified by transcriptome sequencing, respectively (Figure 3A). Among them, a total of 33 genes were obtained by overlapping MHCC-97L and HCCC-9810 cells (Figure 3B). KEGG pathway enrichment analysis was performed on these 33 genes. The most enriched pathways among the tumor-related signaling pathways in which scoparone might be involved were cell cycle-related signaling pathways (Figure 3C). We validated the expression of these genes using qRT-PCR and found that, compared to the control group, there was an increased expression of CDKN1A and decreased expression of CDC25A, CCND1, CCNE1, CDK2, CDK3, and CDK4 (Figure 3D). To further clarify the impact of scoparone on the cell cycle of HCC cells, we performed flow cytometry on MHCC-97L and HCCC-9810 cells treated with different concentrations of scoparone for 48 hours. The results indicated an increase in the abundance of G0/G1 phase, with a corresponding decrease in the S phase in the treatment group, but no noticeable change in the G2/M phase (Figure 3E). This confirms that scoparone inhibits cell proliferation via inducing cell cycle arrest in G0/G1 phase. These experimental findings suggest that scoparone inhibits the proliferation of HCC cells by inducing cell cycle arrest.
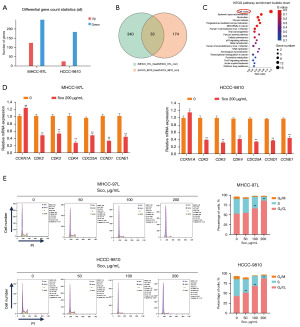
Scoparone suppresses HCC cell proliferation via the AKT/GSK-3β/cyclin D1 signaling pathway
Our previous flow cytometry results confirmed that scoparone induces cell cycle arrest at the G1 phase in HCC cells. The formation of the cyclin D1/CDK4 complex is crucial for cells to progress from the G1 phase to the S phase, and inhibition of this complex can cause cell cycle arrest (17). Therefore, we measured the expression levels of related proteins. After scoparone treatment, the expression levels of G1 phase-related proteins, including CDK2, CDK4, and cyclin D1, were decreased (Figure 4A), consistent with our previous flow cytometry findings. Previous studies have confirmed that in human nasopharyngeal carcinoma cells, inhibiting the AKT/GSK-3β/cyclin D1 signaling pathway promotes cyclin D1 degradation, causing cell cycle arrest at the G1 phase (17,18). Additionally, in our investigation of cell cycle-related signaling pathways, we identified cyclin D1 as a critical target in G1 phase. GSK-3β, an upstream target of cyclin D1, significantly influences cyclin D1 expression by regulating its messenger RNA (mRNA) transcription and protein degradation, playing crucial roles in tumor cell cycle progression. Furthermore, as a substrate of AKT, GSK-3β is important in tumor proliferation. AKT inhibits GSK-3β activity by phosphorylating its inhibitory site Ser9, thereby promoting cyclin D1 expression (19). Consequently, the AKT/GSK-3β/cyclin D1 signaling pathway was analyzed using Western blot. Scoparone treatment did not decrease the total expression of AKT and GSK-3β, but it inhibited the expression of p-AKT (Ser473) and p-GSK-3β (Ser9) (Figure 4B). This finding suggests that scoparone inhibits the proliferation of HCC cells via the AKT/GSK-3β/cyclin D1 signaling pathway.
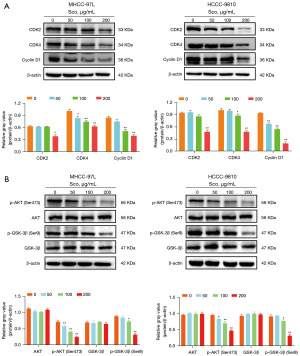
To further validate our findings, rescue experiments were conducted using the AKT pathway activator SC79. As shown in Figure 5A, scoparone significantly decreased cell viability in both cell lines, while SC79 mildly increased cell viability. Notably, co-treatment of HCC cells with SC79 and scoparone partially reversed scoparone’s inhibitory effect on cell proliferation (Figure 5A). Additionally, DNA replication capability was restored in HCC cells treated with both SC79 and scoparone compared to those treated with scoparone alone (Figure 5B). These results indicate that activation of the AKT pathway partially reverses scoparone’s inhibitory effect on HCC cell proliferation, confirming that AKT pathway is the crucial targets by which scoparone exerts its anti-tumor effects.
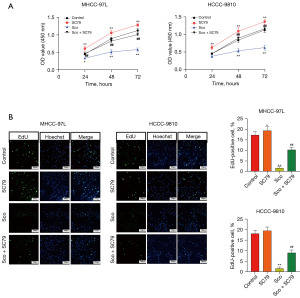
Discussion
Scoparone has been proven to exert antitumor effects in pancreatic cancer, small cell lung cancer, prostate cancer, and CRC (20-23). We investigated the inhibitory effects and molecular mechanisms of scoparone on HCC cell proliferation and cell cycle. Scoparone inhibited proliferation, invasion and metastasis of HCC cells in a dose- and time-dependent manner. MMPs release proteases that degrade the basement membrane, facilitating the entry of tumor cells into the bloodstream and then subsequent metastasis to distant organs, ultimately leading to secondary malignancies. The MMP family, particularly MMP-2 and MMP-9, is closely associated with cancer cell infiltration, metastasis, and poor prognosis (24). Mechanistically, this study found that scoparone inhibits the expression of MMP-2 and MMP-9, thereby suppressing the invasion and migration of HCC cells. This provides a new therapeutic direction and strategy for inhibiting the invasion and metastasis of liver cancer.
There are many challenges in the treatment of HCC, with drug resistance being a significant aspect. Previous studies have shown that abnormal activation of signaling pathways such as PI3K/AKT/mTOR, BNIP3/AMPK/ENO2, and EGFR are closely related to drug resistance in liver cancer (20,21,25). Targeting the mutations and dysregulation of PI3K/AKT signaling pathway in HCC, inhibitors of this signaling pathway have been used in treatment of HCC in several models. PI3K/AKT pathway is overactivated in about 50% of HCC cells, promoting growth, metabolism and survival of HCC cells (22,23). GSK-3β, a substrate of AKT, is associated with the accumulation and nuclear translocation of β-catenin, which in turn promotes the expression of cyclin D1 to facilitate tumor cell growth (26). Moreover, GSK-3β is critically involved in the development and progression of HCC (27) and upregulation of GSK-3β in HCC can predict poor prognosis (28). Cyclin D1, a target of GSK-3β, acts as a regulator of cell cycle progression, promoting cells to pass through the G1/S checkpoint (29). The degradation of cyclin D1 is regulated by GSK-3β, whose activation can lead to a decrease in the protein levels of cyclin D1. AKT phosphorylates the inhibitory phosphorylation site Ser9 in GSK-3β, leading to a decrease in GSK-3β activity and an increase in cyclin D1 expression (19). The AKT/GSK-3β/cyclin D1 signaling pathway plays a crucial role in promoting tumor growth. Interestingly, our study found that scoparone affects the expression of cell cycle-related proteins by inhibiting the AKT/GSK-3β/cyclin D1 signaling pathway, thereby inhibiting cell proliferation, which consists the results that targeting the AKT/GSK-3β/cyclin D1 signaling pathway inhibits HCC proliferation by inducing mitochondrial apoptosis in liver cancer cells (30,31). However, the AKT pathway activator SC79 partially rescued the suppression of cell proliferation confirming that scoparone inhibited HCC cell proliferation and cell cycle progression by suppressing the AKT/GSK-3β/cyclin D1 signaling pathway.
In summary, scoparone exhibits significant hepatoprotective effects and improves liver cirrhosis. Our research, along with studies by several other scholars (32), has demonstrated that scoparone possesses notable antitumor properties and inhibits tumor cell proliferation and cell cycle progression via suppressing AKT/GSK-3β/cyclin D1 signaling pathway. Additionally, scoparone inhibits tumor cell invasion and metastasis by downregulating the expression of MMP-2 and MMP-9. These findings provide insights on new molecular mechanisms for the antitumor effects of scoparone and novel strategies for the treatment of HCC.
Conclusions
This study demonstrates that scoparone, a natural compound, significantly inhibits the proliferation, invasion, and migration of HCC cells in vitro, reducing HCC cell viability in a dose- and time-dependent manner while exhibiting lower toxicity to normal liver cells. Further investigations reveal that scoparone’s inhibition of cell proliferation is achieved by inducing G0/G1 phase cell cycle arrest in HCC cells, a mechanism associated with the AKT/GSK-3β/cyclin D1 signaling pathway. Rescue experiments utilizing the AKT pathway activator SC79 further substantiate that scoparone’s anti-tumor effects are mediated through the inhibition of the AKT pathway, strongly supporting its potential as a therapeutic agent for HCC.
Acknowledgments
None.
Footnote
Reporting Checklist: The authors have completed the MDAR reporting checklist. Available at https://tcr.amegroups.com/article/view/10.21037/tcr-24-1771/rc
Data Sharing Statement: Available at https://tcr.amegroups.com/article/view/10.21037/tcr-24-1771/dss
Peer Review File: Available at https://tcr.amegroups.com/article/view/10.21037/tcr-24-1771/prf
Funding: This work was supported by
Conflicts of Interest: All authors have completed the ICMJE uniform disclosure form (available at https://tcr.amegroups.com/article/view/10.21037/tcr-24-1771/coif). The authors have no conflicts of interest to declare.
Ethical Statement: The authors are accountable for all aspects of the work in ensuring that questions related to the accuracy or integrity of any part of the work are appropriately investigated and resolved.
Open Access Statement: This is an Open Access article distributed in accordance with the Creative Commons Attribution-NonCommercial-NoDerivs 4.0 International License (CC BY-NC-ND 4.0), which permits the non-commercial replication and distribution of the article with the strict proviso that no changes or edits are made and the original work is properly cited (including links to both the formal publication through the relevant DOI and the license). See: https://creativecommons.org/licenses/by-nc-nd/4.0/.
References
- Siegel RL, Miller KD, Fuchs HE, et al. Cancer statistics, 2022. CA Cancer J Clin 2022;72:7-33. [Crossref] [PubMed]
- Wang W, Wei C. Advances in the early diagnosis of hepatocellular carcinoma. Genes Dis 2020;7:308-19. [Crossref] [PubMed]
- Grem JL. 5-Fluorouracil: forty-plus and still ticking. A review of its preclinical and clinical development. Invest New Drugs 2000;18:299-313. [Crossref] [PubMed]
- Kalyan A, Nimeiri H, Kulik L. Systemic therapy of hepatocellular carcinoma: current and promising. Clin Liver Dis 2015;19:421-32. [Crossref] [PubMed]
- Shen YC, Lin ZZ, Hsu CH, et al. Clinical trials in hepatocellular carcinoma: an update. Liver Cancer 2013;2:345-64. [Crossref] [PubMed]
- Yang D, Yang J, Shi D, et al. Scoparone potentiates transactivation of the bile salt export pump gene and this effect is enhanced by cytochrome P450 metabolism but abolished by a PKC inhibitor. Br J Pharmacol 2011;164:1547-57. [Crossref] [PubMed]
- Hui Y, Wang X, Yu Z, et al. Scoparone as a therapeutic drug in liver diseases: Pharmacology, pharmacokinetics and molecular mechanisms of action. Pharmacol Res 2020;160:105170. [Crossref] [PubMed]
- Huang X, Gao Y, Cao H, et al. Effects of scoparone on non-alcoholic fatty liver disease revealed by RNA sequencing. Front Endocrinol (Lausanne) 2022;13:1004284. [Crossref] [PubMed]
- Wei M, Li T, Cao H, et al. The effects of scoparone on alcohol and high-fat diet-induced liver injury revealed by RNA sequencing. Biomed Pharmacother 2022;155:113770. [Crossref] [PubMed]
- Liu B, Deng X, Jiang Q, et al. Scoparone alleviates inflammation, apoptosis and fibrosis of non-alcoholic steatohepatitis by suppressing the TLR4/NF-κB signaling pathway in mice. Int Immunopharmacol 2019;75:105797. [Crossref] [PubMed]
- Liu B, Deng X, Jiang Q, et al. Scoparone improves hepatic inflammation and autophagy in mice with nonalcoholic steatohepatitis by regulating the ROS/P38/Nrf2 axis and PI3K/AKT/mTOR pathway in macrophages. Biomed Pharmacother 2020;125:109895. [Crossref] [PubMed]
- Kang JW, Kim DW, Choi JS, et al. Scoparone attenuates D-galactosamine/lipopolysaccharide-induced fulminant hepatic failure through inhibition of toll-like receptor 4 signaling in mice. Food Chem Toxicol 2013;57:132-9. [Crossref] [PubMed]
- Li N, Yang F, Liu DY, et al. Scoparone inhibits pancreatic cancer through PI3K/Akt signaling pathway. World J Gastrointest Oncol 2021;13:1164-83. [Crossref] [PubMed]
- Shen H, Wei Y, Yang Q, et al. Scoparone induces both apoptosis and ferroptosis via multiple mechanisms in non-small-cell lung cancer cells. Toxicol In Vitro 2023;91:105627. [Crossref] [PubMed]
- Huang S, Lin L, Ma Y, et al. Scoparone induces autophagic cell death via the PAK1/AKT axis in colorectal cancer. Eur J Pharmacol 2023;959:176091. [Crossref] [PubMed]
- Kim JK, Kim JY, Kim HJ, et al. Scoparone exerts anti-tumor activity against DU145 prostate cancer cells via inhibition of STAT3 activity. PLoS One 2013;8:e80391. [Crossref] [PubMed]
- Ong CS, Zhou J, Ong CN, et al. Luteolin induces G1 arrest in human nasopharyngeal carcinoma cells via the Akt-GSK-3β-Cyclin D1 pathway. Cancer Lett 2010;298:167-75. [Crossref] [PubMed]
- Romdhoni AC, Rajanagara AS, Albab CF, et al. The Role of Cyclin d1 in Radiotherapy Resistance of Advance Stage Nasopharyngeal Carcinoma: A Systematic Review. Asian Pac J Cancer Prev 2024;25:2211-8. [Crossref] [PubMed]
- Takahashi-Yanaga F, Sasaguri T. GSK-3beta regulates cyclin D1 expression: a new target for chemotherapy. Cell Signal 2008;20:581-9. [Crossref] [PubMed]
- Shimura T, Kakuda S, Ochiai Y, et al. Targeting the AKT/GSK3β/cyclin D1/Cdk4 survival signaling pathway for eradication of tumor radioresistance acquired by fractionated radiotherapy. Int J Radiat Oncol Biol Phys 2011;80:540-8. [Crossref] [PubMed]
- Huang A, Yang XR, Chung WY, et al. Targeted therapy for hepatocellular carcinoma. Signal Transduct Target Ther 2020;5:146. [Crossref] [PubMed]
- Chen C, Wang G. Mechanisms of hepatocellular carcinoma and challenges and opportunities for molecular targeted therapy. World J Hepatol 2015;7:1964-70. [Crossref] [PubMed]
- Whittaker S, Marais R, Zhu AX. The role of signaling pathways in the development and treatment of hepatocellular carcinoma. Oncogene 2010;29:4989-5005. [Crossref] [PubMed]
- Okazaki I, Inagaki Y. Novel strategies for hepatocellular carcinoma based on MMPs science. Anticancer Agents Med Chem 2012;12:753-63. [Crossref] [PubMed]
- Wang S, Cheng H, Li M, et al. BNIP3-mediated mitophagy boosts the competitive growth of Lenvatinib-resistant cells via energy metabolism reprogramming in HCC. Cell Death Dis 2024;15:484. [Crossref] [PubMed]
- Wang H, Liu Y, Wang D, et al. The Upstream Pathway of mTOR-Mediated Autophagy in Liver Diseases. Cells 2019;8:1597. [Crossref] [PubMed]
- Cervello M, Augello G, Cusimano A, et al. Pivotal roles of glycogen synthase-3 in hepatocellular carcinoma. Adv Biol Regul 2017;65:59-76. [Crossref] [PubMed]
- Fang G, Zhang P, Liu J, et al. Inhibition of GSK-3β activity suppresses HCC malignant phenotype by inhibiting glycolysis via activating AMPK/mTOR signaling. Cancer Lett 2019;463:11-26. [Crossref] [PubMed]
- Xi J, Sun Y, Zhang M, et al. GLS1 promotes proliferation in hepatocellular carcinoma cells via AKT/GSK3β/CyclinD1 pathway. Exp Cell Res 2019;381:1-9. [Crossref] [PubMed]
- Gu Y, Chen B, Guo D, et al. Up-Regulation of RACGAP1 Promotes Progressions of Hepatocellular Carcinoma Regulated by GABPA via PI3K/AKT Pathway. Oxid Med Cell Longev 2022;2022:3034150. [Crossref] [PubMed]
- Bai C, Zhao J, Su J, et al. Curcumin induces mitochondrial apoptosis in human hepatoma cells through BCLAF1-mediated modulation of PI3K/AKT/GSK-3β signaling. Life Sci 2022;306:120804. [Crossref] [PubMed]
- Ye M, Liu C, Liu J, et al. Scoparone inhibits the development of hepatocellular carcinoma by modulating the p38 MAPK/Akt/NF-κB signaling in nonalcoholic fatty liver disease mice. Environ Toxicol 2024;39:551-61. [Crossref] [PubMed]