Small peritoneal macrophages are accelerators of peritoneal metastasis of colorectal cancer
Highlight box
Key findings
• Small peritoneal macrophages (SPMs) play a catalytic role in the progression of peritoneal metastasis of colorectal cancer (PM-CRC) and represent potential therapeutic targets.
What is known and what is new?
• Peritoneal macrophages can be classified into large peritoneal macrophages (LPMs) and SPMs, with their characteristics previously delineated.
• The timing of intervention for the depletion of peritoneal macrophages is crucial, highlighting the potential of SPMs as therapeutic targets for PM-CRC.
What is the implication, and what should change now?
• Given their therapeutic potential, SPMs warrant further investigation as targets for patients with PM-CRC. Additional clinical studies are necessary to validate these findings.
Introduction
Colorectal cancer ranks as the third most prevalent malignant neoplasm worldwide and constitutes the second leading cause of cancer-related mortality (1). The peritoneum frequently serves as a site for metastasis and, in certain instances, represents the sole location of recurrence, with an incidence rate reaching up to 25% (2). Approximately 7% of patients with colorectal cancer will develop peritoneal metastasis during primary surgical intervention. Furthermore, during the follow-up period post-radical surgery, between 4% and 19% of patients are likely to develop peritoneal metastasis. Among patients who die from colorectal cancer, 40% to 80% of them die due to the occurrence of peritoneal metastasis (3). Therefore, it is imperative to conduct an in-depth investigation into the progression factors and therapeutic targets of peritoneal metastasis of colorectal cancer (PM-CRC).
PM-CRC represents a form of distant metastasis characterized by the invasion of the primary tumor into the serosa. Subsequently, the aggressive anterior cancer cells detach from the tumor mass and enter the peritoneal cavity (PerC). These cells undergo a series of processes, including matrix degradation, adhesion, and angiogenesis, ultimately leading to their attachment and colonization of the peritoneum, thereby completing the metastatic process (4). Factors such as epithelial-mesenchymal transition and the immune system play significant roles in this progression (4). Moreover, the lymphatic system is intricately linked to peritoneal metastasis. In a rat model of mesenteric lymphatic occlusion, observations of lymphatic contact with veins and mesenteric lymphatic regurgitation of intestinal lymphatic fluid indicate that the accumulation of cancer cells within lymphatic vessels can facilitate peritoneal metastasis (5).
Cancer cells reside within a complex microenvironment known as the tumor microenvironment (TME), which comprises stromal cells, endothelial cells, and immune cells (6). The peritoneum and ascitic fluid create a unique local TME for peritoneal metastatic cancer foci. Within the peritoneum, macrophages represent the predominant immune cell population (7). Macrophages can generally be polarized into the classically activated M1 subtype and the alternatively activated M2 subtype, collectively known as tumor-associated macrophages (TAMs). These TAMs secrete a variety of cytokines that facilitate cancer cell proliferation, metastasis, and tumor tissue angiogenesis (8). Current research indicates that in various solid malignancies, including breast cancer, prostate cancer, and gastric cancer, the localization and density of TAMs are correlated with adverse clinical outcomes (9-11). The role of TAMs in colorectal cancer CRC is multifaceted (12). This complexity likely arises from the heterogeneity of macrophage subtypes present within the abdominal cavity. Consequently, further investigation into the specific functional characteristics of these various peritoneal macrophage subtypes could offer novel therapeutic avenues for addressing PM-CRC.
Peritoneal macrophages, a specialized subset of macrophages, are situated within the abdominal cavity. Investigating the unique characteristics and functions of these cells can substantially enhance our comprehensive understanding of macrophage biology. Within the PerC, there coexist two macrophage subsets, namely large peritoneal macrophages (LPMs) and small peritoneal macrophages (SPMs), which differ from each other in terms of development, phenotype as well as function (13). SPMs are characterized by low expression levels of F4/80 and cluster of differentiation 11b (CD11b) CD11b, high expression levels of major histocompatibility complex II (MHCII), and an origin from the bone marrow. Although typically representing a minor population, SPMs possess the capacity to migrate from circulating monocytes to the peritoneum and infiltrate sites of infection when required. In contrast, LPMs, which are characterized by high expression levels of F4/80 and CD11b and a lack of MHC II expression, originate from the embryonic yolk sac and demonstrate the ability to self-renew and proliferate rapidly in response to inflammatory stimuli (13,14). In healthy mice, LPMs constitute the predominant macrophage population in the abdominal cavity, accounting for approximately 90% of resident macrophages (15).
Therefore, distinct subpopulations of peritoneal macrophages may differentially contribute to the progression of colorectal cancer peritoneal metastasis. In our study, we observed a rapid increase in SPMs concomitant with the advancement of colorectal cancer peritoneal metastasis. This alteration may play a pivotal role in accelerating the progression of the disease by disrupting immune homeostasis and impairing immune surveillance. This study elucidates the distinctive role of SPMs in a murine model of colorectal cancer peritoneal metastasis, demonstrating their accelerating effect on the progression of this condition. The depletion of SPMs significantly extends the survival time of mice afflicted with colorectal cancer peritoneal metastasis, thereby indicating a potential therapeutic target for this malignancy. We present this article in accordance with the ARRIVE reporting checklist (available at https://tcr.amegroups.com/article/view/10.21037/tcr-24-1707/rc).
Methods
Cell cultures
MC38 cells, obtained from the Chinese Academy of Sciences, were cultured at 37 ℃ and 5% CO2 in Dulbecco’s Modified Eagle’s Medium (Hyclone, Logan, USA) supplemented with 10% fetal bovine serum (Gibco, New York, USA).
Animals and experimental design
Eighty Male C57BL/6 mice (ten weeks old, 20 g), sourced from Shanghai Jie Si Jie Laboratory Animal Co., Ltd. (Shanghai, China), were maintained under specific pathogen-free conditions at the Infection Key Laboratory in Shanghai, Chongming, China. C57BL/6 mice were administered an intraperitoneal injection of 1×106 MC38 cells. One week subsequent to tumor challenge, the mice were randomly allocated into a normal control group and an experimental group (12 mice in each group). Euthanasia was performed on mice that exhibited a body weight increase exceeding 20% of their initial weight or demonstrated other signs of distress, such as impaired mobility, reduced food intake, or difficulty walking. Body weights were recorded every second day. Peritoneal cells were harvested by injecting 6 mL of staining medium [Roswell Park Memorial Institute (RPMI) deficient in certain components, supplemented with 5% neonatal calf serum] into the PerC. Experiments were performed under a project license (No. 2022-NSFC-24-310230199410104551) granted by the Shanghai University of Medicine and Health Sciences, in compliance with the national guidelines for the care and use of animals. A protocol was prepared before the study without registration.
Antibodies
The antibodies utilized for fluorescence activated cell sorting (FACS) staining included: anti-human and mouse CD11b-allophycocyanin (APC) (clone M1/70, BioLegend, San Diego, USA), anti-mouse F4/80-phycoerythrin (PE)/Cyanine7 (clone BM8, BioLegend), anti-mouse I-A/I-E (MHC II)-peridinin-chlorophyll-protein complex (PerCP) (clone M51/114.15.2, BioLegend), anti-mouse CD206-PE (clone C068C2, BioLegend), anti-mouse CD45-fluorescein isothiocyanate (FITC) (clone I3/2.3, BioLegend), and anti-mouse IL-10-APC/Cyanine7 (clone JES5-16E3, BioLegend).
FACS staining
Cell suspensions were subjected to centrifugation at 450 g for 10 minutes at 4 ℃. The resulting cell pellets were resuspended in red blood cell lysis solution (Biosharp, Hefei, China) for 2 minutes, followed by termination with phosphate buffered saline (PBS) buffer. Subsequent centrifugation at 450 g for 10 minutes was performed, and the cell pellets were carefully resuspended in a 2.5% BSA solution. A sample of 1×105 cells per tube was prepared for subsequent FACS staining. The cells were incubated with a fluorochrome-conjugated antibody for 30 minutes at 4 ℃ in the dark, and then resuspended in FACS buffer prior to analysis using the Becton, Dickinson and company (BD) FACSCanto special order research products (SORP).
In vivo stimulation
In the in vivo experiment, a dose of 5 µg of lipopolysaccharide (LPS), dissolved in 200 µL of phosphate-buffered saline solution, was administered via intraperitoneal injection. Subsequently, the PerC was harvested, subjected to staining procedures, and analyzed at predetermined time intervals. The PM-CRC mice were randomized into control and the LPS stimulation groups (10 mice in each group) before treatment to ensure uniformity in weight and age within each group.
SPMs isolation
On day 30 after injection of MC38, PM-CRC mice are used to extract peritoneal cells. The isolated cells were resuspended in 40% Percoll (GE Healthcare, Chicago, USA) and carefully layered onto 60% Percoll solutions, followed by centrifugation at 450 g for 25 minutes at 4 ℃. Subsequently, the collected cells were cultured at 37 ℃ with 5% CO2 in the presence of 10% fetal bovine serum (Gibco). Non-adherent cells were discarded after a 2-hour incubation period.
Transwell cell co-culture
Peritoneal macrophages, isolated from the abdominal cavity of tumor-bearing mice, and MC38 cells were co-cultured at a 1:1 ratio. The peritoneal macrophages were seeded in the culture dish located beneath the Transwell chamber. Subsequently, MC38 cells were seeded on the membrane of the upper Transwell chamber, ensuring contact with the culture medium in the lower chamber. The co-culture was maintained with medium changes every 24 hours.
Cell proliferation assay
MC38 cells were co-cultured with SPMs for durations of 24 and 48 hours. Subsequently, a modified 3-(4,5-dimethylthiazol-2-yl)-2,5-diphenyl-tetrazolium bromide (MTT) solution (5 mg/mL, Sigma) was introduced. Then 1 mL of dimethyl sulfoxide (DMSO) was added, followed by gentle shaking for 10 minutes. An aliquot of 200 µL of the resultant solution was transferred to a 96-well plate, and the optical density (OD) was measured at 490 nm using a Varioskan LUX multimode microplate reader (Thermo Fisher, Waltham, USA). The OD values were utilized to determine the number of viable cells.
Macrophage depletion
The PM-CRC mice were randomized into control, the early-stage clearance, the late-stage clearance and the all-stage clearance groups (10 mice in each group) before treatment to ensure uniformity in weight and age within each group, although blinding was not implemented. To achieve in vivo macrophage depletion, clodronate liposomes (CL, Liposoma BV, Amsterdam, Netherlands) or PBS liposomes were administered via intraperitoneal injection to PM-CRC mice at a dosage of 200 µL per mouse.
Statistical analysis
Data were presented as mean ± standard deviation. Comparisons between the values of two groups were conducted using the unpaired, two-tailed Student’s t-test and two-way additive analysis of variance (ANOVA). Survival analysis was performed employing the Log-rank (Mantel-Cox) test. Statistical analyses were executed using Prism 7.0 software (GraphPad). P<0.05 was considered to be statistically significant.
Results
Dramatic changes in the macrophage populations present in the abdomen during PM-CRC
To investigate the role of peritoneal macrophages in PM-CRC, the colorectal cancer cell line MC38 was intraperitoneally injected into C57BL/6 mice to establish a murine PM-CRC model. Each mouse received an injection of 1×106 MC38 cells suspended in 100 µL of PBS (the control group received an injection of 100 µL of PBS), and their body weight (Figure 1A) and survival periods were monitored. The majority of the mice succumbed within about 40 to 60 days post-injection (Figure 1B). Additionally, peritoneal effusions were collected from the model mice on days 10, 20, and 30 post-injection to assess the changes in peritoneal macrophage subpopulations during the progression of PM-CRC. The results indicated a declining trend in both the number and proportion of LPMs in mice during the progression of PM-CRC (Figure 1C-1E). Conversely, the number and proportion of SPMs exhibited an increasing trend (Figure 1C-1E).
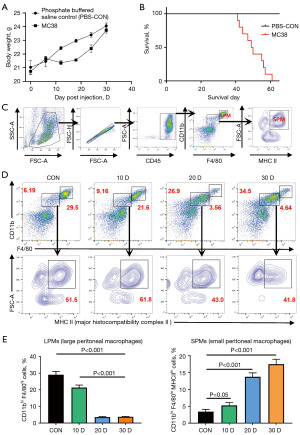
SPMs showed carcinogenesis-promoting characteristics
Utilizing flow cytometry, a notable upregulation of CD206 and the immunosuppressive factor interleukin-10 (IL-10) was detected in SPMs, but not in LPMs, of tumor-bearing mice (Figure 2A,2B). This observation suggests that SPMs undergo polarization towards a protumor phenotype, which is recognized for its role in facilitating tumor progression and metastasis. These findings offer a novel perspective on the involvement of SPMs within the TME. We investigated the role of SPMs in promoting PM-CRC through in vitro experiments. Initially, SPMs were extracted and isolated from the PerC of advanced PM-CRC mice (30 days after injection). The purity of the isolated SPMs was assessed using immunofluorescence, revealing that the expression of the macrophage marker F4/80 exceeded 95% (Figure 2C,2D). Subsequently, the SPMs were co-cultured with MC38 cells for 48 h (Figure 2E). The MTT assay results demonstrated that the OD values for MC38 cells co-cultured with SPMs were significantly higher compared to those of MC38 cells not co-cultured with SPMs at both 24 and 48 hours. This indicates that SPMs have the potential to enhance the proliferation of MC38 cells (Figure 2F).
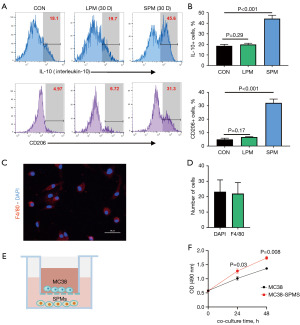
LPS stimulation increasing SPMs accelerates the death of PM-CRC mice
Previous research has demonstrated that intraperitoneal administration of LPS induces a rapid elevation in the levels of SPMs (16). Examination of PerC samples collected from animals on the second and fourth days post low dose LPS (5 µg) stimulation revealed a marked reduction in the number of LPMs, the macrophage subpopulation typically predominant in the PerC (Figure 3A,3B). In contrast, the number of SPMs increased, subsequently becoming the dominant macrophage population (Figure 3A,3B). These findings prompted us to administer LPS on the 5th, 10th and 15th days following the establishment of the PM-CRC model, with the aim of inducing a rapid increase in the proportion of SPMs in the PerC of mice during the early stages of tumor growth (Figure 3C). Upon analyzing the data, it was observed that the survival rate of mice treated with LPS was reduced (Figure 3D). This suggests that alterations in the proportion of SPMs play a significant role in the disease progression of PM-CRC mice. Consequently, these results underscore the critical importance of maintaining a low proportion of SPMs for the survival of these mice.
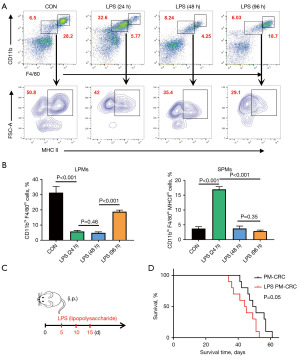
Depletion of SPMs prolongs the survival period of PM-CRC mice
Based on the aforementioned experimental results, clodronate liposomes were subsequently employed for macrophage depletion (17) to sustain a reduced proportion of SPMs within the PM-CRC model. Clodronate liposomes have been shown to effectively deplete macrophage in the murine abdomen (Figure 4A,4B). Consequently, the treatment protocols entailed the administration of clodronate liposomes to the PM-CRC early-stage clearance group (on the 5th, 8th, 11th and 14th days), the late-stage clearance group (on the 18th, 21st, 24th and 27th days), and the all-stage clearance group (on the 5th, 8th, 11th, 14th, 18th, 21st, 24th, and 27th days) with the objective of targeting macrophage elimination in the abdominal cavity at distinct stages (Figure 4C). The results indicated that the survival rate of mice in the late clearance group was significantly higher compared to the PBS liposomes group, whereas the early clearance group did not exhibit a significant effect (Figure 4D). Furthermore, no significant difference was observed in the effect between the full clearance group and the late clearance group (Figure 4D). These findings suggest that the clearance of peritoneal macrophages during the late phase of rapid SPMs growth, as opposed to the early phase dominated by LPMs, can effectively prolong the survival of PM-CRC mice. Consequently, these results indicate that SPMs play a promotive role in the progression of PM-CRC.
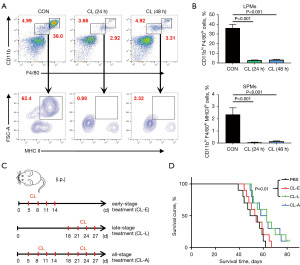
Discussion
In recent years, immunotherapy has demonstrated significant efficacy in the treatment of various cancer types (18,19). However, the presence of immune cells with immunosuppressive properties within the TME has constrained its clinical benefits (20). Notably, the interaction between cancer cells and TAMs facilitates immune evasion by cancer cells, thereby promoting tumor progression (21,22). Macrophages, being heterogeneous cells, are typically classified into two distinct subgroups. Pro-inflammatory M1 macrophages are known to drive the immune response, whereas anti-inflammatory M2 macrophages facilitate tumor progression (23). Considering the ubiquitous presence of macrophages in all adult tissues, resident macrophages likely play a significant role in the tissue microenvironment that supports the malignant progression of disseminated cancer cells.
Specifically, resident macrophages in the PerC can be categorized into two distinct subgroups: LPMs and SPMs (13). These subgroups are integral to maintaining intraperitoneal homeostasis under various conditions, including inflammation and tumorigenesis. LPMs is distinguished by elevated expression of CD11b and F4/80 markers, alongside diminished expression of MHC-II. This population constitutes the predominant macrophage subset within the abdominal cavity of healthy mice, representing approximately 90% of resident macrophages (24). Conversely, SPMs is characterized by reduced expression of CD11b and F4/80 markers and elevated expression of MHC-II, comprising about 10% of resident macrophages in unstimulated mice (25). The differentiation of these cells from circulating monocytes typically occurs at a low level, thereby maintaining homeostasis in healthy animals.
In the context of infection or inflammatory stimuli, the cellular composition within the abdominal cavity undergoes substantial alterations. Notably, there is a marked reduction in LPMs, with SPMs and their precursor inflammatory monocytes becoming the predominant cell populations (15). Etzerodt et al. demonstrated that the depletion of Tim4+ peritoneal macrophages inhibited the progression of ovarian cancer and the metastatic dissemination of the disease (26). These findings suggest that peritoneal macrophages play a critical role in facilitating the malignant progression of cancer cells within the abdominal cavity.
In this study, we elucidated the characteristics and role of SPMs in a murine PM-CRC model. We observed a rapid increase in SPMs during the middle and late stages of PM-CRC progression, accompanied by the onset of ascites production. Notably, the expression of the CD206 and the immunosuppressive cytokine IL-10 in SPMs was significantly upregulated, suggesting that these macrophages polarized towards an protumor phenotype. Based on these findings, we hypothesized that SPMs might facilitate tumor progression. To test this hypothesis, we conducted mechanistic experiments to investigate the functional role of these immune cells in the context of PM-CRC. In vitro experiments demonstrated that SPMs significantly enhances the proliferation activity of MC38 cells. Furthermore, intraperitoneal administration of LPS resulted in a rapid increase in SPMs levels and a marked reduction in LPMs numbers, concomitant with a decrease in mouse survival rates. These findings suggest that alterations in the proportion of SPMs are pivotal in the disease progression of PM-CRC mice, and maintaining a low proportion of SPMs is essential for their survival. Subsequent macrophage depletion experiments utilizing clodronate liposomes demonstrated that administering clodronate liposomes to ablate SPMs on late-stage of PM-CRC mice significantly enhanced mouse survival rates. Conversely, administration of clodronate liposomes at an earlier stage primarily depleted LPMs, resulting in no significant alteration in mouse survival rates. These findings suggest that targeted intervention in mouse peritoneal macrophage subsets to restore a low proportion of SPMs can effectively inhibit the progression of PM-CRC. Consistent with prior research, macrophage depletion via clodronate liposomes has demonstrated efficacy in treating various murine cancer models (17,27). However, the findings of this study underscore the critical importance of the timing of macrophage depletion interventions. Given the varying proportions of macrophage subsets within the TME at different stages of cancer progression, the therapeutic outcomes may differ substantially.
In human pathology, peritoneal metastases are frequently linked to a multitude of intricate factors, including the patient’s pre-existing health conditions, comorbidities, and the heterogeneity of tumor cells, all of which can intensify complications such as obstruction. In our murine model, the direct injection of a tumor model into the PerC may not sufficiently replicate the complexity inherent in human disease, as it differs from secondary metastases observed in colorectal cancer. Consequently, the extent to which these findings can be generalized to human subjects warrants further investigation. And the association between SPMs and patient prognosis requires confirmation through the analysis of clinical samples. Furthermore, the precise mechanism through which the elevated presence of SPMs contributes to the promotion of PM-CRC remains inadequately understood. This phenomenon is likely intricately associated with inflammatory factors present within the TME of the abdominal cavity (15). Consequently, more extensive research is necessary to uncover the relevant molecular pathways and signal transduction mechanisms involved. Incorporating related molecular targets and signaling pathways provides a theoretical foundation for the development of more targeted therapeutic approaches. Our findings indicate that in the PM-CRC mice, SPMs play a distinct role in the malignant progression of tumor cells. These studies have significantly enhanced our understanding of TAMs heterogeneity and the specific contributions of different macrophage subsets to disease progression. The interaction between SPMs and cancer cells may represent novel and important therapeutic targets.
Conclusions
By developing a mouse model of PM-CRC, this study observed a significant increase in SPMs during the progression of PM-CRC, which exhibited properties conducive to tumor growth. The removal of SPMs was found to effectively extend the survival period of mice with PM-CRC, suggesting that SPMs may serve as a promising therapeutic target for this condition.
Acknowledgments
None.
Footnote
Reporting Checklist: The authors have completed the ARRIVE reporting checklist. Available at https://tcr.amegroups.com/article/view/10.21037/tcr-24-1707/rc
Data Sharing Statement: Available at https://tcr.amegroups.com/article/view/10.21037/tcr-24-1707/dss
Peer Review File: Available at https://tcr.amegroups.com/article/view/10.21037/tcr-24-1707/prf
Funding: This work was supported by
Conflicts of Interest: All authors have completed the ICMJE uniform disclosure form (available at https://tcr.amegroups.com/article/view/10.21037/tcr-24-1707/coif). The authors have no conflicts of interest to declare.
Ethical Statement: The authors are accountable for all aspects of the work in ensuring that questions related to the accuracy or integrity of any part of the work are appropriately investigated and resolved. Experiments were performed under a project license (No. 2022-NSFC-24-310230199410104551) granted by the Shanghai University of Medicine and Health Sciences, in compliance with the national guidelines for the care and use of animals.
Open Access Statement: This is an Open Access article distributed in accordance with the Creative Commons Attribution-NonCommercial-NoDerivs 4.0 International License (CC BY-NC-ND 4.0), which permits the non-commercial replication and distribution of the article with the strict proviso that no changes or edits are made and the original work is properly cited (including links to both the formal publication through the relevant DOI and the license). See: https://creativecommons.org/licenses/by-nc-nd/4.0/.
References
- Bastiaenen VP, Klaver CEL, van der Heijden MCS, et al. A mouse model for peritoneal metastases of colorectal origin recapitulates patient heterogeneity. Lab Invest 2020;100:1465-74. [Crossref] [PubMed]
- Ihn MH, Kim DW, Cho S, et al. Curative Resection for Metachronous Pulmonary Metastases from Colorectal Cancer: Analysis of Survival Rates and Prognostic Factors. Cancer Res Treat 2017;49:104-15. [Crossref] [PubMed]
- Lee JH, Ahn BK, Baik SS, et al. Comprehensive Analysis of Somatic Mutations in Colorectal Cancer With Peritoneal Metastasis. In Vivo 2019;33:447-52. [Crossref] [PubMed]
- Tokuda A, Miyake T, Yasukawa D, et al. Cancer-derived Exosomes Activate Immune Surveillance and Suppress Peritoneal Metastasis of Murine Colonic Cancer. Anticancer Res 2021;41:1327-39. [Crossref] [PubMed]
- Qiu C, Li Y, Liang X, et al. A study of peritoneal metastatic xenograft model of colorectal cancer in the treatment of hyperthermic intraperitoneal chemotherapy with Raltitrexed. Biomed Pharmacother 2017;92:149-56. [Crossref] [PubMed]
- Liu W, Zhou H, Lai W, et al. The immunosuppressive landscape in tumor microenvironment. Immunol Res 2024;72:566-82. [Crossref] [PubMed]
- Zhang Y, Ouyang D, Chen YH, et al. Peritoneal resident macrophages in tumor metastasis and immunotherapy. Front Cell Dev Biol 2022;10:948952. [Crossref] [PubMed]
- Dallavalasa S, Beeraka NM, Basavaraju CG, et al. The Role of Tumor Associated Macrophages (TAMs) in Cancer Progression, Chemoresistance, Angiogenesis and Metastasis - Current Status. Curr Med Chem 2021;28:8203-36. [Crossref] [PubMed]
- Shi T, Zhang Y, Wang Y, et al. DKK1 Promotes Tumor Immune Evasion and Impedes Anti-PD-1 Treatment by Inducing Immunosuppressive Macrophages in Gastric Cancer. Cancer Immunol Res 2022;10:1506-24. [Crossref] [PubMed]
- Mehraj U, Dar AH, Wani NA, et al. Tumor microenvironment promotes breast cancer chemoresistance. Cancer Chemother Pharmacol 2021;87:147-58. [Crossref] [PubMed]
- Masetti M, Carriero R, Portale F, et al. Lipid-loaded tumor-associated macrophages sustain tumor growth and invasiveness in prostate cancer. J Exp Med 2022;219:e20210564. [Crossref] [PubMed]
- Wang H, Tian T, Zhang J. Tumor-Associated Macrophages (TAMs) in Colorectal Cancer (CRC): From Mechanism to Therapy and Prognosis. Int J Mol Sci 2021;22:8470. [Crossref] [PubMed]
- Prat M, Coulson K, Blot C, et al. PPARγ activation modulates the balance of peritoneal macrophage populations to suppress ovarian tumor growth and tumor-induced immunosuppression. J Immunother Cancer 2023;11:e007031. [Crossref] [PubMed]
- Kim KH, Park D, Cho SY, et al. Role of histamine-mediated macrophage differentiation in clearance of metastatic bacterial infection. Front Immunol 2023;14:1290191. [Crossref] [PubMed]
- Ardavín C, Alvarez-Ladrón N, Ferriz M, et al. Mouse Tissue-Resident Peritoneal Macrophages in Homeostasis, Repair, Infection, and Tumor Metastasis. Adv Sci (Weinh) 2023;10:e2206617. [Crossref] [PubMed]
- Valdes-Fernandez BN, Ruiz-Jimenez C, Armina-Rodriguez A, et al. Fasciola hepatica GST mu-class suppresses the cytokine storm induced by E. coli-lipopolysaccharide, whereas it modulates the dynamic of peritoneal macrophages in a mouse model and suppresses the classical activation of macrophages. Microbiol Spectr 2024;12:e0347523. [Crossref] [PubMed]
- Opperman KS, Vandyke K, Clark KC, et al. Clodronate-Liposome Mediated Macrophage Depletion Abrogates Multiple Myeloma Tumor Establishment In Vivo. Neoplasia 2019;21:777-87. [Crossref] [PubMed]
- Ling SP, Ming LC, Dhaliwal JS, et al. Role of Immunotherapy in the Treatment of Cancer: A Systematic Review. Cancers (Basel) 2022;14:5205. [Crossref] [PubMed]
- Taefehshokr N, Baradaran B, Baghbanzadeh A, et al. Promising approaches in cancer immunotherapy. Immunobiology 2020;225:151875. [Crossref] [PubMed]
- Nakamura K, Smyth MJ. Myeloid immunosuppression and immune checkpoints in the tumor microenvironment. Cell Mol Immunol 2020;17:1-12. [Crossref] [PubMed]
- Pan Y, Yu Y, Wang X, et al. Tumor-Associated Macrophages in Tumor Immunity. Front Immunol 2020;11:583084. [Crossref] [PubMed]
- Xiang X, Wang J, Lu D, et al. Targeting tumor-associated macrophages to synergize tumor immunotherapy. Signal Transduct Target Ther 2021;6:75. [Crossref] [PubMed]
- Boutilier AJ, Elsawa SF. Macrophage Polarization States in the Tumor Microenvironment. Int J Mol Sci 2021;22:6995. [Crossref] [PubMed]
- Salm L, Shim R, Noskovicova N, et al. Gata6(+) large peritoneal macrophages: an evolutionarily conserved sentinel and effector system for infection and injury. Trends Immunol 2023;44:129-45. [Crossref] [PubMed]
- Li J, Zhao F, Qin Q, et al. The Effect of CD226 on the Balance between Inflammatory Monocytes and Small Peritoneal Macrophages in Mouse Ulcerative Colitis. Immunol Invest 2022;51:1833-42. [Crossref] [PubMed]
- Etzerodt A, Moulin M, Doktor TK, et al. Tissue-resident macrophages in omentum promote metastatic spread of ovarian cancer. J Exp Med 2020;217:e20191869. [Crossref] [PubMed]
- Bader JE, Enos RT, Velázquez KT, et al. Macrophage depletion using clodronate liposomes decreases tumorigenesis and alters gut microbiota in the AOM/DSS mouse model of colon cancer. Am J Physiol Gastrointest Liver Physiol 2018;314:G22-31. [Crossref] [PubMed]