Estrogen receptor β inhibits breast cancer migration and promotes its apoptosis through NF-κB/IL-8 signaling
Highlight box
Key findings
• Estrogen receptor (ERβ) suppresses the NF-κB/IL-8 signaling axis by inhibiting the phosphorylation of IκBα and P65, consequently restricting breast cancer cell mobility and migration while promoting apoptosis.
What is known and what is new?
• ERβ is a transcription factor that plays crucial roles in breast cancer. However, the complex regulation of ERβ variants processing leads to its functional opposite in the specific cancer and controversial conclusions in different researches.
• In the present study, we found that ERβ level was decreased significantly but the expression of IL-8 was increased in breast cancer tissue. The underly mechanism is probably achieved by inhibiting the activation of the NF-κB pathway and impairing the production of IL-8 via p65 and IκBα phosphorylation.
What is the implication, and what should change now?
• This study demonstrates that ERβ inhibits the NF-κB/IL-8 signaling axis by suppressing IκBα and P65 phosphorylation, consequently restricting breast cancer cell mobility and migration while promoting apoptosis. Furthermore, we provide mechanistic evidence supporting ERβ’s role in suppressing breast cancer progression. These findings suggest the potential of ERβ as a novel therapeutic target for breast cancer, particularly triple-negative breast cancer, warranting further investigation.
Introduction
Breast cancer is among the most common cancers in the world. Current reports show that its incidence has surpassed lung cancer and ranks first among female malignant tumors. Current breast cancer treatment options include surgery, radiotherapy and chemotherapy, endocrine therapy, and biologically targeted therapy (1). Among them, patients receiving endocrine therapy are mainly estrogen receptor α (ERα) (+) patients; whereas for ERα (−) patients or patients who relapse after endocrine therapy, there is still a lack of effective treatment methods. Furthermore, estrogen acts through two isoforms of this nuclear receptor, ERα and estrogen receptor β (ERβ) in breast cancer. Studies have reported that ERβ is highly expressed in normal breast and 20–30% of invasive breast cancer tissues (2), and the increase in ERβ levels in normal breasts is associated with a reduced risk of breast cancer (3). Moreover, ERβ exerts an anti-proliferation effect on breast cancer cells (BCCs) in the presence of ERα and promotes proliferation when ERα is not expressed (4). Nevertheless, recently Alexandrova et al. (5), confirmed in three triple-negative breast cancer (TNBC) cell lines (HCC1806, MDA-MB-468, and Hs578T) that exogenous expression of ERβ1 reduces the cell proliferation rate and inhibits the clonal potential of TNBC cells. However, the specific mechanism of ERβ involved in the occurrence and development of breast cancer is still unknown.
Interleukin-8 (IL-8) belongs to the CXC chemokine superfamily, which has the functions of chemotaxis, stem cell mobilization, and angiogenesis. Alraouji et al. showed that IL-8 is highly expressed in cancer tissues and plays a crucial role in the progression of different cancers, including breast cancer (6). An in vitro study has confirmed that BCCs promote self-migration by secreting IL-8 to induce neutrophil extracellular traps (NET) formation (7). In vivo experiments revealed that mice injected with normal MDA-MB-231 cells were found liver metastasis of tumor cells, while MDA-MB-231 cells with knockdown IL-8 expression could not induce distant metastasis after injection on mice. These studies suggest that IL-8 can increase tumor cell migration in vivo and in vitro. Most earlier reports attribute it to IL-8’s chemotactic effect on neutrophils, while Deng et al.’s research has shown that IL-8 can up-regulate the expression levels of matrix metallopeptidase 2 (MMP-2) or matrix MMP-9 and activate PI3K-Akt/MAPK signaling pathways to increase TNBC cells invasiveness (8). Additionally, targeted inhibition of the IL-8-CXCR1/2 axis in vivo models can effectively organize BCCs’ growth and spread (9). Moreover, relevant data show that patients with lower plasma IL-8 concentration during chemotherapy have higher long-term survival rates (10). Accordingly, IL-8 plays a vital role in breast cancer diseases’ occurrence, development, and drug resistance.
NF-κB protein can be expressed in almost all animal cells. This protein family can selectively bind to the B cell κ-light chain enhancer, thereby regulating the expression of many genes. As previously described, NF-κB plays an essential role in regulating inflammation and immune response by controlling several important pro- and anti-inflammatory factors under its transcriptional control (11). NF-κB governs an internal relationship between inflammation and cancer, making it critical in controlling malignant cells and pre-tumor cells to escape from standard immune surveillance in the human body (12). Much evidence supports that NF-κB is closely related to tumorigenesis. Additionally, many studies have confirmed that NF-κB can promote breast cancer’s occurrence, development, and resistance, and because of this, NF-κB has become an excellent target for cancer treatment (13,14).
However, the interaction between ERβ and NF-κB pathway in breast cancer has not been thoroughly studied. Here, we propose a scientific hypothesis that ERβ can down-regulate IL-8 in breast cancer by negatively regulating NF-κB, thereby promoting apoptosis of BCCs and inhibiting their proliferation and migration. The objective of this study is to determine the effects of ERβ on the growth, proliferation and invasion of BCCs. Meanwhile, the regulation of ERβ/NF-κB/IL-8 pathway on BCCs was verified. On this basis, the value of ERβ and its related signaling pathways in evaluating the prognosis of breast cancer was further evaluated. We present this article in accordance with the MDAR reporting checklist (available at https://tcr.amegroups.com/article/view/10.21037/tcr-24-1267/rc).
Methods
Patients and tissue specimens
The study was conducted in accordance with the Declaration of Helsinki (as revised in 2013). The study was approved by the Ethics Committee of the Affiliated Hospital of Qingdao University (Qingdao, China) (approval number QYFY WZLL 26734). Informed consent was obtained from all individual participants.
The study involved collecting samples from 20 ERβ-positive patients undergoing modified breast resection or breast-conserving surgery without neoadjuvant chemotherapy at the Affiliated Hospital between June 2020 and December 2020. All the tissue used in this analysis was from fresh frozen.
Cell culture
The human BCC MDA-MB-231 and MCF-7 were purchased from Procell Life Science & Technology Co., Ltd. (Wuhan, China). We used a high glucose Dulbecco’s Modified Eagle Medium (DMEM) containing 10% fetal bovine serum (FBS) to cultivate the cells, which were placed in a humidified incubator with an atmosphere of 5% CO2 at 37 ℃. Next, the cells were transferred into new culture flasks at a ratio of 1:3. We constructed the ERβ-up cell line by lentiviral infection and set the blank lentivirus-infected cell line as vector group.
Cell viability analysis
A cell density of 1×103 cells/well was inoculated into 96-well plates. The Cell Counting Kit-8 (CCK-8) assay kit (Solarbio, Beijing, China) was used to analyze cell viability. The medium (90 µL) and CCK-8 reagent (10 µL) were added to each well. After 1–4 h, we measured the absorbance at 450 nm using a microplate reader (Tecan, Austria GmbH). Six samples were removed from each group into 96-well plates for the experiment.
Flow cytometry analysis
We cultured the cells until they reached approximately 90% confluence. Then, the cells were labeled with an Annexin V-PE/7-AAD apoptosis detection kit (Meilune Biotechnology Co. Ltd., Dalian, China) and analyzed on an Apogee A50-MICRO flow cytometer (UK) to quantify the percentage of apoptotic cells. This analysis was performed in triplicate.
Western blot analysis
The total proteins cleaved from the cells were electrophoresed in a 12.5% sodium dodecyl sulfate-polyacrylamide gel electrophoresis (SDS-PAGE). The proteins were transferred to polyvinylidene fluoride membranes (PVDF) and then blocked with 5% fat-free milk powder for 1.5 hours. Next, these membranes were incubated with primary antibody at 4 ℃ overnight and then incubated with secondary antibody at room temperature for 2 h. Enhanced chemiluminescence-plus (ECL-PLUS) reagent (MilliporeSigma, Burlington, MA, USA) was used to visualize the target bands, which were scanned using the BioSpectrum imaging system (UVP; Thermo Fisher Scientific, Waltham, MA, USA). The results were normalized to glyceraldehyde 3-phosphate dehydrogenase (GAPDH) levels. Following are all the antibodies involved: ERβ (Abcam, ab3576, 1:1,000, Cambridge, UK); IL-8 (Abcam, ab110727, 1:5,000); Bax (Cell Signaling Technology, #14796, 1:1,000, Danvers, MA, USA); Bcl2 (Cell Signaling Technology, #4223, 1:1,000); Cleaved capase3 (Cell Signaling Technology, #9661, 1:1,000); P65 (Cell Signaling Technology, #4764, 1:1,000); Phospho-P65 (p-P65) (Cell Signaling Technology, #3033, 1:1,000); GAPDH (Cell Signaling Technology, #5174, 1:1,000); Phospho-IκBα (p-IκBα) (Abclonal, AP1201, 1:1,000, Wuxi, China); HRP Goat Anti-Rabbit IgG (Abclonal, AS014, 1:5000); and Gat Anti-Mouse IgG (Abcam, ab6789, 1:5,000).
RNA extraction and quantitative real-time polymerase chain reaction (qRT-PCR)
Total RNA was extracted from the cells using Trizol reagent (Invitrogen; Thermo Fisher Scientific) according to the manufacturer’s protocol. The RNA was then reverse transcribed using oligo dT primers. Complementary DNA (cDNA) was used as a template to amplify target genes with the SYBR Premix Ex Tag kit (Takara Bio, Inc., Kusatsu, Japan) using primers listed in Table 1. Each RNA sample was evaluated in triplicate PCR cycles. Relative messenger RNA(mRNA) expression was determined using the 2-ΔΔCt method and normalized to GAPDH expression.
Table 1
Gene | Primer sequence (5'-3') |
---|---|
ERβ | F: GATTCCCGGCTTTGTGGAG |
R: TCTCGAAACCTTGAAGTAGTTGC | |
IL-8 | F: TAAACAAGTTTCAACCAGCAAG |
R: CTTGTCATTGCCAGCTGTGT | |
GAPDH | F: GGGAGCCAAAAGGCTCATCATCTC |
R: CCATGCCAGTGAGCTTCCCGT |
qRT-PCR, quantitative real-time polymerase chain reaction; ERβ, estrogen receptor β; IL-8, interleukin-8.
Plate clone formation assay
Cells in the logarithmic growth phase were inoculated into 6-well plates at a density of 1×103 cells/well and then cultured in a cell incubator for one week. Set up one 6-well plate for each group. Next, the medium was removed, and cells were fixed with 4% paraformaldehyde for 15 minutes. Then, they were stained with crystal violet dye for 10 minutes, washed off slowly with running water, and then air-dried for the following measurements.
Cell migration analysis
A density of 8×104 cells was seeded into the upper chamber containing 200 µL of serum-free DMEM. Then, 500 µL of complete DMEM containing 10% FBS was placed in the lower chamber. After 6 h of culture at 37 ℃, they were taken out of the upper chamber, fixed with 4% paraformaldehyde, and dyed the whole chamber with 0.05% crystal violet. Then, after three washes with phosphate buffer saline (PBS), the stained cells were counted under an inverted phase-contrast microscope. Repeat the above steps three times.
Wound healing assay
The cells of each group were inoculated into a 6-well culture plate and cultured until the fusion degree reached about 90%. Then, we drew a straight line perpendicular to the monolayer with the tip of a sterile pipette and rinsed it with PBS to remove cell debris. The scratch widths were recorded at different times (0, 24, 36, and 48 h), and Image J software was used to calculate the scratch healing rate.
Statistical analysis
The Student’s t-test was used for statistical analysis, and P values of 0.05 or less were considered statistically significant.
Data availability
The authors confirm that the data supporting the findings of this study are available within the article.
Results
Differential expression of ERβ and IL-8 in breast cancer and adjacent tissues
In the 20 pairs of breast cancer and adjacent tissue samples collected, we used Western blot to detect the protein levels of ERβ and IL-8 in 3 pairs of tissues. The expression of ERβ protein was higher in the adjacent tissues, and the expression of IL-8 protein was higher in the cancer tissues (Figure 1A, P=0.006; P=0.002). We further investigated ERβ and IL-8 expression at the mRNA level using qRT-PCR in all 20 tissue pairs. Consistent with the Western blot results, qRT-PCR analysis revealed a significant negative correlation between ERβ and IL-8 mRNA expression (Figure 1B, both P<0.001).
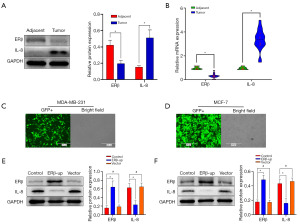
Role of ERβ in regulating BCC mobility, migration, and apoptosis via IL-8 expression, comparable to pyrrolidinedithiocarbamate ammonium (PDTC) effects
We verified the ERβ expression in the two BCC lines, transfected with lentivirus, using fluorescence observation (Figure 1C,1D) and Western blotting (Figure 1E, P<0.001; Figure 1F, P<0.001). The expression of IL-8 was significantly reduced in the ERβ-up group (Figure 1E, P<0.001; Figure 1F, P<0.001).
It was observed that the cell viability of the ERβ-up group was significantly delayed compared to that of the control group (Figure 2A, P<0.001; Figure 2B, P<0.001). We then performed a colony formation assay to record the number of colony formations in each group of cells. The results suggested that with the overexpression of ERβ, the number of established colonies became smaller (Figure 3A, P<0.001; Figure 3B, P<0.001).
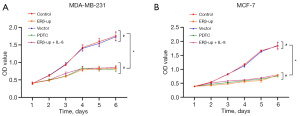
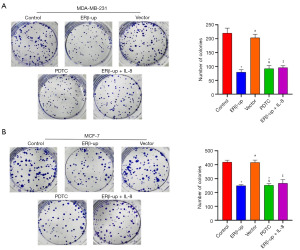
To investigate the effect of ERβ on BCC mobility, we employed a wound-healing assay. We monitored scratch width at 0 and 48 hours, and the calculated healing rate indicated that ERβ significantly suppressed BCC mobility (Figure 4A, P<0.001; Figure 4B, P<0.001). Subsequently, a transwell assay was used to assess BCC migration. The results showed that BCCs with ERβ overexpression exhibited significantly reduced migration capability compared to the control group (Figure 5A, P<0.001; Figure 5B, P<0.001). Collectively, these findings demonstrate that ERβ inhibits both mobility and migration of BCCs. After elucidating that ERβ inhibits the proliferation and migration of BCCs, we further explored whether ERβ can promote the apoptosis of BCCs. The results revealed that the percentage of apoptotic cells in the ERβ-up group increased significantly compared to the control group (Figure 6A, P<0.001; Figure 6B, P<0.001).
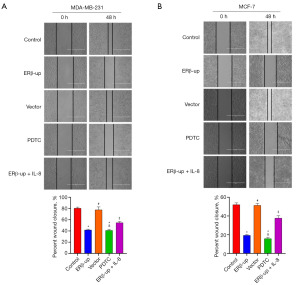
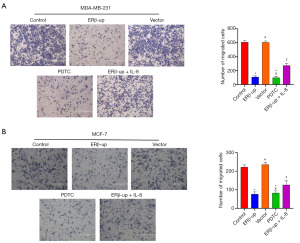
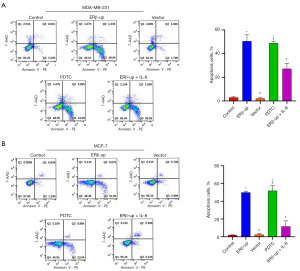
These results demonstrated that ERβ inhibited the proliferation and viability of BCCs, suggesting its potential to suppress tumor growth. However, this effect may not be solely mediated by down-regulating IL-8. To explore this further, we exogenously added IL-8 (PEPRO TECH, 150 ng/mL) to ERβ-overexpressing cells. While the addition of IL-8 partially rescued the inhibitory effect of ERβ on migration (Figures 4,5) and apoptosis (Figure 6), its effect on cell viability (Figure 2) and cell proliferation (Figure 3) remained unchanged. Interestingly, treatment with PDTC (AbMole, 25 uM), a specific NF-κB pathway inhibitor, in normal cells (MDA-MB-231 and MCF-7 that did not overexpress ERβ) mimicked the effects of ERβ overexpression on BCCs (Figures 2-6, all P<0.001). Western blot analysis revealed that both ERβ overexpression and PDTC treatment promoted apoptosis in BCCs by upregulating Bax and cleaved caspase-3, and down-regulating Bcl-2 (Figure 7, all P<0.001).
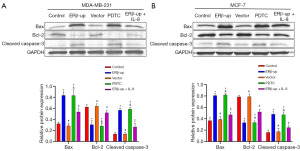
ERβ inhibits the NF-κB/IL-8 signal axis by inhibiting IκBα and P65 phosphorylation
A previous study has shown that ERβ inhibits the NF-κB pathway in prostate cancer cells by preventing P65 nuclear translocation and IκBα phosphorylation (15). This mechanism aligns with how PDTC inhibits NF-κB. We investigated this potential mechanism in BCCs by analyzing protein expression using Western blot. P-P65 and p-IκBα levels were significantly reduced in both the ERβ-upregulated and PDTC groups (Figure 8A, p-P65/P65: ERβ vs. control, P=0.002, PDTC vs. control, P=0.005; p-IκBα: ERβ vs. control, P<0.001, PDTC vs. control, P<0.001; Figure 8B, p-P65/P65: ERβ vs. control, P=0.002, PDTC vs. control, P=0.001; p-IκBα: ERβ vs. control, P<0.001, PDTC vs. control, P<0.001). Notably, total P65 levels remained unchanged. These findings suggest that ERβ in BCCs might regulate NF-κB through a similar mechanism, potentially leading to reduced IL-8 expression.
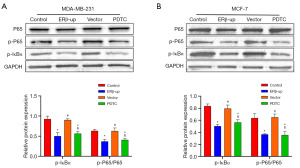
Discussion
Despite its established tumor suppressor role in various cancers (16), the function of ERβ in breast cancer, particularly TNBC, remains controversial. This ambiguity might be due to two factors. First, ERβ shares high homology (95% and 55%) in its DNA binding and ligand-binding domains with ERα (17). Second, ERβ exists in five splice variants (ERβ1–5). Except for ERβ1, the functional full-length form, other isoforms require ERβ1, ERα, or other factors to form functional dimers, leading to diverse functions (18). With the optimization of ERβ monoclonal antibodies and the emergence of highly selective ERβ agonists, more and more in vivo and in vitro studies have confirmed the anti-tumor effect of ERβ in breast cancer (19,20). ERβ status has also become a well-recognized predictor of the survival rate of breast cancer in female patients (21).
As an essential member of inflammatory cytokines, IL-8 has been demonstrated to be related to poor prognosis in patients with ERα (+) breast cancer and TNBC (22,23). NF-κB is one of the critical regulatory pathways of IL-8 (24). The latest research reveals that the NF-κB/IL-8 signaling axis is crucial for the formation of breast cancer stem cells and may become a new target for future treatments (25).
Building on evidence that ERβ suppresses the NF-κB pathway and exerts anti-cancer effects in prostate cancer by inhibiting P65 and p-IκBα (15), we investigated its role in breast cancer. The NF-κB/IL-8 signaling axis is known to influence BCC behavior (25). Our study revealed a negative correlation between ERβ and IL-8 expression in breast cancer tissues compared to adjacent healthy tissues. Furthermore, BCCs overexpressing ERβ displayed downregulated IL-8 expression. Interestingly, exogenous IL-8 partially rescued the inhibitory effect of ERβ on BCC migration and apoptosis, but not on cell proliferation. This is consistent with a previous study that IL-8 seems not to affect BCC proliferation and cell cycle (26), suggesting that ERβ may regulate BCC proliferation through an IL-8 independent pathway. However, previous research reported that recombinant IL-8 could up-regulate the proliferation and migration ability of BCCs (27), which seems to require more experiments to verify. In addition, animal experiments are missing from our experiments, which can be used to explore the role of ERβ on breast cancer in the in vivo environment.
Likewise, the addition of PDTC and the overexpression of ERβ in normal cells (MDA-MB-231 and MCF-7 that did not overexpress ERβ) performed a similar role in this study case, and the overexpression of ERβ significantly inhibited the phosphorylation of IκBα and P65; an indication that ERβ inhibits the conduction of the NF-κB/IL-8 signaling pathway, thereby enabling a tumor suppressor effect.
Conclusions
This study demonstrates that ERβ inhibits the NF-κB/IL-8 signaling axis by suppressing IκBα and P65 phosphorylation, consequently restricting BCC mobility and migration while promoting apoptosis. Furthermore, we provide mechanistic evidence supporting ERβ’s role in suppressing breast cancer progression. These findings suggest the potential of ERβ as a novel therapeutic target for breast cancer, particularly TNBC, warranting further investigation.
Acknowledgments
None.
Footnote
Reporting Checklist: The authors have completed the MDAR reporting checklist. Available at https://tcr.amegroups.com/article/view/10.21037/tcr-24-1267/rc
Data Sharing Statement: Available at https://tcr.amegroups.com/article/view/10.21037/tcr-24-1267/dss
Peer Review File: Available at https://tcr.amegroups.com/article/view/10.21037/tcr-24-1267/prf
Funding: The study was supported by
Conflicts of Interest: All authors have completed the ICMJE uniform disclosure form (available at https://tcr.amegroups.com/article/view/10.21037/tcr-24-1267/coif). The authors have no conflicts of interest to declare.
Ethical Statement: The authors are accountable for all aspects of the work in ensuring that questions related to the accuracy or integrity of any part of the work are appropriately investigated and resolved. The study was conducted in accordance with the Declaration of Helsinki (as revised in 2013). The study was approved by the Ethics Committee of the Affiliated Hospital of Qingdao University (Qingdao, China) (approval number QYFY WZLL 26734). Informed consent was obtained from all individual participants.
Open Access Statement: This is an Open Access article distributed in accordance with the Creative Commons Attribution-NonCommercial-NoDerivs 4.0 International License (CC BY-NC-ND 4.0), which permits the non-commercial replication and distribution of the article with the strict proviso that no changes or edits are made and the original work is properly cited (including links to both the formal publication through the relevant DOI and the license). See: https://creativecommons.org/licenses/by-nc-nd/4.0/.
References
- Hashemzadeh N, Dolatkhah M, Adibkia K, et al. Recent advances in breast cancer immunotherapy: The promising impact of nanomedicines. Life Sci 2021;271:119110. [Crossref] [PubMed]
- Hawse JR, Carter JM, Aspros KGM, et al. Optimized immunohistochemical detection of estrogen receptor beta using two validated monoclonal antibodies confirms its expression in normal and malignant breast tissues. Breast Cancer Res Treat 2020;179:241-9. [Crossref] [PubMed]
- Mal R, Magner A, David J, et al. Estrogen Receptor Beta (ERβ): A Ligand Activated Tumor Suppressor. Front Oncol 2020;10:587386. [Crossref] [PubMed]
- Hussein S, Khanna P, Yunus N, et al. Nuclear Receptor-Mediated Metabolic Reprogramming and the Impact on HR+ Breast Cancer. Cancers (Basel) 2021;13:4808. [Crossref] [PubMed]
- Alexandrova E, Giurato G, Saggese P, et al. Interaction Proteomics Identifies ERbeta Association with Chromatin Repressive Complexes to Inhibit Cholesterol Biosynthesis and Exert An Oncosuppressive Role in Triple-negative Breast Cancer. Mol Cell Proteomics 2020;19:245-60. [Crossref] [PubMed]
- Alraouji NN, Aboussekhra A. Tocilizumab inhibits IL-8 and the proangiogenic potential of triple negative breast cancer cells. Mol Carcinog 2021;60:51-9. [Crossref] [PubMed]
- Cai Z, Zhang M, Boafo Kwantwi L, et al. Breast cancer cells promote self-migration by secreting interleukin 8 to induce NET formation. Gene 2020;754:144902. [Crossref] [PubMed]
- Deng F, Weng Y, Li X, et al. Overexpression of IL-8 promotes cell migration via PI3K-Akt signaling pathway and EMT in triple-negative breast cancer. Pathol Res Pract 2021;223:152824. [Crossref] [PubMed]
- Bouris P, Manou D, Sopaki-Valalaki A, et al. Serglycin promotes breast cancer cell aggressiveness: Induction of epithelial to mesenchymal transition, proteolytic activity and IL-8 signaling. Matrix Biol 2018;74:35-51. [Crossref] [PubMed]
- Tiainen L, Hämäläinen M, Luukkaala T, et al. Low Plasma IL-8 Levels During Chemotherapy Are Predictive of Excellent Long-Term Survival in Metastatic Breast Cancer. Clin Breast Cancer 2019;19:e522-33. [Crossref] [PubMed]
- Tapryal N, Shahabi S, Chakraborty A, et al. Intrapulmonary administration of purified NEIL2 abrogates NF-κB-mediated inflammation. J Biol Chem 2021;296:100723. [Crossref] [PubMed]
- Suhail M, Tarique M, Muhammad N, et al. A Critical Transcription Factor NF-κB as a Cancer Therapeutic Target and its Inhibitors as Cancer Treatment Options. Curr Med Chem 2021;28:4117-32. [Crossref] [PubMed]
- Abdin SM, Tolba MF, Zaher DM, et al. Nuclear factor-κB signaling inhibitors revert multidrug-resistance in breast cancer cells. Chem Biol Interact 2021;340:109450. [Crossref] [PubMed]
- Xu Y, Zhang S, Liao X, et al. Circular RNA circIKBKB promotes breast cancer bone metastasis through sustaining NF-κB/bone remodeling factors signaling. Mol Cancer 2021;20:98. [Crossref] [PubMed]
- Xiao L, Luo Y, Tai R, et al. Estrogen receptor β suppresses inflammation and the progression of prostate cancer. Mol Med Rep 2019;19:3555-63. [Crossref] [PubMed]
- Song P, Li Y, Dong Y, et al. Estrogen receptor β inhibits breast cancer cells migration and invasion through CLDN6-mediated autophagy. J Exp Clin Cancer Res 2019;38:354. [Crossref] [PubMed]
- Kuiper GG, Enmark E, Pelto-Huikko M, et al. Cloning of a novel receptor expressed in rat prostate and ovary. Proc Natl Acad Sci U S A 1996;93:5925-30. [Crossref] [PubMed]
- Lee MT, Ouyang B, Ho SM, et al. Differential expression of estrogen receptor beta isoforms in prostate cancer through interplay between transcriptional and translational regulation. Mol Cell Endocrinol 2013;376:125-35. [Crossref] [PubMed]
- Sotoca AM, van den Berg H, Vervoort J, et al. Influence of cellular ERalpha/ERbeta ratio on the ERalpha-agonist induced proliferation of human T47D breast cancer cells. Toxicol Sci 2008;105:303-11. [Crossref] [PubMed]
- Yuan B, Cheng L, Gupta K, et al. Tyrosine phosphorylation regulates ERβ ubiquitination, protein turnover, and inhibition of breast cancer. Oncotarget 2016;7:42585-97. [Crossref] [PubMed]
- Hwang NM, Stabile LP. Estrogen Receptor ß in Cancer: To ß(e) or not to ß(e)? Endocrinology 2021;162:bqab162. [Crossref] [PubMed]
- Hartman ZC, Poage GM, den Hollander P, et al. Growth of triple-negative breast cancer cells relies upon coordinate autocrine expression of the proinflammatory cytokines IL-6 and IL-8. Cancer Res 2013;73:3470-80. [Crossref] [PubMed]
- Milovanović J, Todorović-Raković N, Radulovic M. Interleukin-6 and interleukin-8 serum levels in prognosis of hormone-dependent breast cancer. Cytokine 2019;118:93-8. [Crossref] [PubMed]
- Freund A, Jolivel V, Durand S, et al. Mechanisms underlying differential expression of interleukin-8 in breast cancer cells. Oncogene 2004;23:6105-14. [Crossref] [PubMed]
- Choi HS, Kim JH, Kim SL, et al. Disruption of the NF-κB/IL-8 Signaling Axis by Sulconazole Inhibits Human Breast Cancer Stem Cell Formation. Cells 2019;8:1007. [Crossref] [PubMed]
- Yao C, Lin Y, Chua MS, et al. Interleukin-8 modulates growth and invasiveness of estrogen receptor-negative breast cancer cells. Int J Cancer 2007;121:1949-57. [Crossref] [PubMed]
- Tang Z, Ding S, Huang H, et al. HDAC1 triggers the proliferation and migration of breast cancer cells via upregulation of interleukin-8. Biol Chem 2017;398:1347-56. [Crossref] [PubMed]