Induction of ferroptosis in oxaliplatin-resistant colorectal cancer cells by extract from Actinidia chinensis Planch radix
Highlight box
Key findings
• Extract from Actinidia chinensis Planch radix significantly inhibited the proliferation of oxaliplatin-resistant colorectal cancer (CRC) cells, suggesting its potential to reverse drug resistance. The extract triggered ferroptosis, a type of iron-dependent cell death, characterized by lipid peroxidation, in the resistant CRC cells, indicating a novel mechanism for overcoming chemoresistance. These findings indicate that A. chinensis Planch radix extract could serve as a potential therapeutic strategy for overcoming oxaliplatin resistance in CRC through ferroptosis induction and gene modulation.
What is known and what is new?
• Oxaliplatin, a standard chemotherapeutic drug for CRC, often leads to the emergence of drug-resistant cancer cells, reducing treatment efficacy. Ferroptosis, a form of programmed cell death dependent on iron and characterized by lipid peroxidation, has been identified as a potential mechanism for killing cancer cells, but its role in overcoming chemoresistance is still under investigation.
• This study is the first to demonstrate that A. chinensis Planch radix can target oxaliplatin-resistant CRC cells, highlighting its potential use as an adjuvant therapy in patients with treatment-resistant CRC.
What is the implication, and what should change now?
• Our results may provide a new approach for the future treatment of CRC and inform the development of novel drugs and therapeutic technologies. Further clinical trials are warranted to further validate these findings.
Introduction
Colorectal cancer (CRC) is the third most common cancer worldwide. Although progress has been made in the screening and treatment of patients with CRC, the prevalence and mortality associated with this disease are increasing worldwide and affecting patients in both developing and industrialized countries. Moreover, CRC is the second most common cause of cancer-related death (1), and recent research has reported a rise in the incidence of early-onset CRC (2). Therefore, the development of additional therapeutic options for CRC is imperative.
Chemotherapy remains a mainstream treatment for advanced CRC, and cytotoxic agents with or without anti-epidermal growth factor receptor (EGFR) treatment remain the first-line chemotherapy for advanced CRC (3,4). Among the cytotoxic agents, oxaliplatin is one of the most critical to therapy, as indicated in clinical trials (5,6). However, resistance to oxaliplatin in patients with CRC can arise due to the selection against oxaliplatin-sensitive cancer cells (7,8). When chemoresistance occurs, patients experience a poorer outcome and have fewer therapeutic alternatives, and thus it is crucial that the means to restoring the responsiveness to oxaliplatin is clarified.
Traditional Chinese medicine has been historically used for the treatment of chronic disorders including cancer. Actinidia chinensis Planch radix is plentiful in the southern part of China, and its root can be used for medical purposes. According to traditional Chinese medicine book “The Yellow Emperor’s Inner Canon”, A. chinensis Planch radix exerts therapeutic effects in those with cancer, fever, toxicity, etc. (9), and A. chinensis Planch radix has been used in cancer treatment in modern laboratory settings (10,11). However, whether A. chinensis Planch radix can reverse resistance to oxaliplatin in CRC remains unclear.
Ferroptosis is a recently discovered iron-mediated cell death process and is characterized by the accumulation of intracellular reactive oxygen species (ROS) (12). Downregulation of glutathione peroxide (GPX), especially GPX4, is a characteristic marker of ferroptosis (13), and a decrease in the expression of transferrin receptor 1 (TFRC1) induces ferroptosis (14). It has been reported that ferroptosis might have a role in the anticancer effect in oxaliplatin-resistant CRC (15). Ubiquitin-specific protease (USP7), a member of the deubiquitinase protein family (DUB), has been extensively studied. It is highly expressed in multiple cancers, is regarded as a biomarker for poor outcomes in patients with cancer (16,17), and more importantly, is involved in chemoresistance (16,17). It has also been reported that the presence of the wild-type protein 53 (p53) protein is correlated with responsiveness to oxaliplatin in CRC cells (18,19). However, whether A. chinensis Planch radix induces ferroptosis in oxaliplatin-resistant CRC remains unclear.
In this study, we tested our hypothesis that extract from A. chinensis Planch radix can induce ferroptosis in oxaliplatin-resistant CRC cells by increasing the expression of p53 and decreasing that of USP7 and TFRC1. The findings of this study provide insights into the potential clinical applications of traditional Chinese medicine in incurable oxaliplatin-resistant CRC.
Methods
Preparation of A. chinensis Planch radix root extracts
To prepare the A. chinensis Planch radix root extracts, roots (donated by Zhengjiang Traditional Chinese Medical University) were washed, chopped, and placed in double-distilled water. The mixture was heated to 100 ℃ for 1 h under reflux condensation to prevent solvent loss, followed by filtration through a 0.22 µm cellulose membrane (MilliporeSigma, Burlington, MA, USA). The aqueous extract was freeze-dried using a Labconco FreeZone 2.5 L lyophilizer (−50 ℃, 48 h), yielding a dry powder. The final concentration of extract was 500 µg/mL.
Cell culture
HT29, a human colorectal adenocarcinoma cell line, was purchased from the American Type Culture Collection (Manassas, VA, USA). Cells were cultured in McCoy’s 5A medium supplemented with 10% of fetal bovine serum (FBS) (Gibco, Thermo Fisher Scientific, Waltham, MA, USA). Cells were cultured at 37 ℃ and 5% CO2 in a cell culture incubator. To generate an oxaliplatin-resistant daughter cell line, parental CRC cells were chronically exposed to stepwise increasing concentrations of oxaliplatin (Sigma-Aldrich, St. Louis, MO, USA) dissolved in serum-free Dulbecco’s modified Eagle's medium (DMEM) containing 0.1% dimethyl sulfoxide (DMSO) as vehicle. Starting from the baseline half maximal inhibitory concentration (IC50) concentration (0.1 µM) determined by preliminary Cell Counting Kit-8 (CCK-8) assay, the exposure gradient was progressively escalated over 8 weeks (0.1→0.5→1.0→5.0→10 µM) with medium replacement every 3 days. Prior to each concentration increment, cells underwent serum starvation for 24 h in 1% FBS medium to synchronize cell cycle progression. Drug resistance was confirmed through three consecutive passages showing >5-fold increase in IC50 (0.52±0.07 µM in parental vs. 2.89±0.12 µM in resistant cells, P<0.001) and maintained in 1.0 µM oxaliplatin during routine culture (20).
MTT (3-[4,5-dimethylthiazol-2-yl]-2,5-diphenyltetrazolium-bromide) assay
Cell proliferation was measured by MTT assays. Different types of cells seeded in 96-well plates and treated with various conditions were used for the MTT assays. At designated time points, cell culture medium was removed, and 100 µL of diluted MTT (Sigma-Aldrich) solution was added to each well. The plates were then placed in the incubator at 37 ℃ and 5% CO2 for another 2 h. At the end of the culture, DMSO was added to the system to dissolve the crystals. The absorbance at 540 nm was obtained from a plate reader.
Wound healing assay
Cells were seeded into six-well plates and cultured to complete confluence. Cells were then serum starved in serum-free medium. Artificial wounds were induced using as sterile 200-µL pipette tip. Cells were then washed using serum-free culture medium. Images were obtained at 0 and 36 h using an inverted microscope (Olympus, Tokyo, Japan). Migration ability was calculated according to changes in the size of the wound.
Quantitative polymerase chain reaction
Total RNA was isolated using a commercial RNA extraction kit (RNeasy Kit, Qiagen, Hilden, Germany). Complementary DNA was generated with PrimeScrip RT Reagent Kit (Takara Bio, Kusatsu, Japan) according to the manufacturer’s guidelines. The expression of genes of interest was examined via quantitative real-time polymerase chain reaction with Power SYBR Green PCR master mix (Applied Biosystems, Thermo Fisher Scientific). All primers were purchased from OriGene (Rockville, MD, USA).
Western blotting
Total protein was obtained using cell lysis buffer (KeyGene, Wageningen, The Netherlands), and the concentration of protein was measured using the Lowry method. Proteins were loaded and separated in 8% sodium dodecyl-sulfate polyacrylamide gel for electrophoresis. They were then transferred to polyvinylidene fluoride (PVDF) membranes (MilliporeSigma, Burlington, MA, USA). Membranes were blocked using 5% bovine serum albumin for 1 h before primary antibodies (Abcam, Cambridge, UK) were applied overnight at 4 ℃. After thorough washing, horseradish peroxidase-conjugated secondary antibodies (Abcam) were incubated to the membranes for 1 h at room temperature. Bands were visualized using an enhanced chemiluminescence reagent (MilliporeSigma).
Statistical analysis
Data are presented as the mean ± standard deviation (SD). Differences between more than two groups were compared using Student’s t-test (two-tailed, unpaired) to compare differences between two experimental groups. All statistical analyses were conducted using GraphPad Prism version 8.0 (GraphPad Software, La Jolla, CA, USA) for Windows. A P value of less than 0.05 was considered to indicate statistical significance.
Results
Generation of oxaliplatin-resistant CRC cells
In order to test the effects of A. chinensis Planch radix extract on oxaliplatin-resistant CRC cell lines, we sought to first generate such cell lines. As described above, we used an escalated gradient of oxaliplatin in the cell culture media to generate chemotherapy-resistant cell lines. A gradient between 3 and 20 µM was chosen. As shown in Figure 1, oxaliplatin-resistant HT29 cells were generated, which showed an increased ability to survive in various concentrations of oxaliplatin (Figure 1A) and at different time points (Figure 1B). These cells were examined in the subsequent experiments.
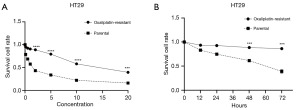
Extract from A. chinensis Planch radix inhibited the proliferation of oxaliplatin-resistant CRC cells
Next, we sought to determine if extract from A. chinensis Planch radix could suppress increased levels of proliferation in oxaliplatin-resistant CRC. When cultured in oxaliplatin-free culture media, oxaliplatin-resistant cells showed a fast rate of growth at 48 h after seeding (Figure 2A). As shown in Figure 2A, with the presence of oxaliplatin in the cell culture medium (10 µM), oxaliplatin-resistant HT29 cells showed enhanced survival over time; application of extract from A. chinensis Planch radix (treatment), however, decreased the rates of survival in oxaliplatin-resistant CRC cells (green line in Figure 2). It is worth noting that treatment did not decrease the survival rate of oxaliplatin-sensitive parent cells. We also conducted wound healing assays using oxaliplatin-resistant cells and their parental controls. As demonstrated in Figure 2B, oxaliplatin-resistant HT29 cells showed an increased ability to heal the artificial wounding in culture with the presence of oxaliplatin, but application of extract from A. chinensis Planch radix reduced the speed of healing to the levels of parental cells. These data indicate that extract from A. chinensis Planch radix is effective in reversing drug resistance in oxaliplatin-resistant HT29 cells.
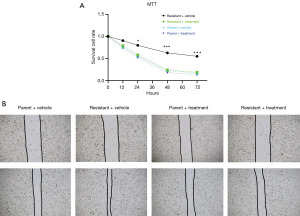
Extract from A. chinensis Planch radix induced ferroptosis in oxaliplatin-resistant CRC cells
We next aimed to clarify the mechanisms related to the reversal of responsiveness in oxaliplatin-resistant HT29 cells mediated by extract from A. chinensis Planch radix. It has been reported that extract from A. chinensis Planch induces ferroptosis in gastric cancer cells by decreasing the expression of GPX4 (21). We sought to determine whether a similar mechanism would apply in the context of oxaliplatin resistance in CRC cells. We added extract from A. chinensis Planch radix in the cell culture media with the presence of oxaliplatin. As shown in Figure 3A,3B, the expression of transferrin—a ferroptosis marker—was significantly elevated in oxaliplatin-resistant HT29 cells treated with the extract. Conversely, GPX4 expression was reduced in resistant cells supplemented with the extract (Figure 3A,3C). Notably, the ‘Parent + treatment’ group exhibited lower transferrin levels compared to the ‘Parent + vehicle’ group (Figure 3B), suggesting that the extract may exert distinct regulatory effects on ferroptosis markers in parental versus resistant cells. While GPX4 levels remained unchanged in parental cells, the observed reduction in transferrin implies that the treatment could modulate iron metabolism or ferroptosis sensitivity in a cell type-specific manner. This differential response may reflect inherent variations in redox homeostasis or iron-handling pathways between parental and resistant cells, potentially linked to oxaliplatin resistance mechanisms. Further investigation into the interplay between transferrin regulation, GPX4-independent ferroptosis pathways, and drug-resistant phenotypes is warranted to elucidate these findings.
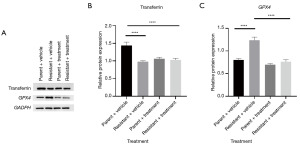
Gene expression profiles altered by extract from A. chinensis Planch radix
We then attempted to characterize the changes in gene expression associated with the addition of extract from A. chinensis Planch radix in the context of oxaliplatin. It has been reported that USP7 (16,17), wild-type p53 (18,19), and TFRC1 (22) are associated with chemoresistance. We subsequently examined the expression of these genes at the messenger RNA (mRNA) level. As shown in Figure 4, USP7 and TFRC1 were significantly upregulated in oxaliplatin-resistant HT29 cells, but application of extract remarkably reversed this increase, returning it to the baseline level. Moreover, the expression of wild-type p53 was reduced in oxaliplatin-resistant HT29 cells, and administration of extract increased its expression to reach that of parent cells.
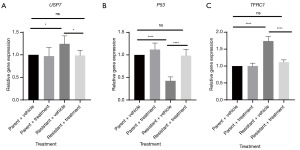
Protein levels of gene expression
We additionally examined the protein levels of gene expression mediated by extract from A. chinensis Planch radix. As shown in Figure 5, at the protein level, extract from A. chinensis Planch radix increased expression of USP7 and TFRC1 in oxaliplatin-resistant HT29 cells and decreased that of wild-type p53. These data, along with those provided in Figure 4, indicate that extract from A. chinensis Planch radix mediates the change in key elements associated with chemoresistance.
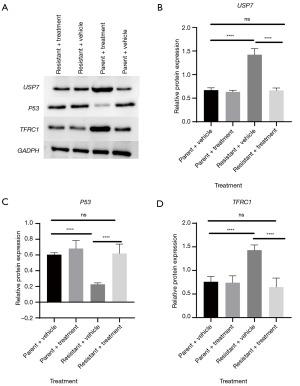
Discussion
In this study, we found that extract from A. chinensis Planch radix reversed chemoresistance in oxaliplatin-resistant CRC cells. This reversal was achieved via the induction of ferroptosis and the alteration of chemoresistance-related genes.
CRC, which accounts for approximately 10% of all cancer cases worldwide, is common in both genders. Although the primary treatment option for CRC is surgery, chemotherapy or radiotherapy can be used for adjuvant therapy. However, due to heterogeneity of tumors and repeated application of chemotherapy reagents, the prevalence of chemoresistant CRC has been increasing. Given that oxaliplatin-based regimens are involved in first-line chemotherapy, it is important to clarify the mechanism by which chemoresistance arises in order to develop novel treatments for patients.
In our study, we first established an oxaliplatin-resistant cell line. As shown in Figure 1, oxaliplatin-resistant HT29 cells. Upon being exposed to elevated concentrations of oxaliplatin in the culture medium, the selected cells exhibited resistance to oxaliplatin in cell culture.
Unsurprisingly, these oxaliplatin-resistant cells were proliferative and had an increased ability to migrate during wound healing assays. In the oxaliplatin-resistant CRC cells were generated, key genes related to chemoresistance, including USP7, TFRC1, and wild-type p53, showed different expression profiles compared to parental cells at both the mRNA and protein levels, indicating these oxaliplatin-resistant CRC cells harbor different transcription regulations. More importantly, when extract from A. chinensis Planch radix was applied to oxaliplatin-resistant CRC cells in our experimental system, the changes in expression of these abovementioned gene changes were reversed (Figures 4,5). Moreover, extract from A. chinensis Planch radix induced ferroptosis in oxaliplatin-resistant CRC cells, proposing a mechanism to suppress the proliferation of oxaliplatin-resistant CRC cells.
Extract from A. chinensis Planch radix has been shown to be effective in killing cancer cells, including hepatocellular carcinoma (9,23), gastric cancer (24), lung cancer (10,25), and others. In this study, we also found that extract from A. chinensis Planch radix reverses oxaliplatin resistance in CRC cells, suggesting this plant might have broader applications in cancers. A. chinensis Planch is an angiosperm grown in southern China, and its root extract has considerable medical value; however, given the complexity of the chemical composition of this extract, it is extremely difficult to identify the specific molecular components responsible for its anticancer properties. Triterpenes, flavonoids, phenolics, polysaccharides, and steroids have been speculated to be responsible for these anticancer effects (10). In our study, we were unable to isolate those chemical compounds with anticancer features, and thus additional studies involving chemical isolation and purification are needed.
A few other limitations to this study should also be acknowledged. Firstly, how extract from A. chinensis Planch radix induces ferroptosis remains unclear. It is possible that the application of extract from A. chinensis Planch radix renders cancer cells more sensitive to iron, but more studies are needed to confirm this. Secondly, it remains unclear whether the administration of A. chinensis Planch radix extract can suppress cancer in animal models. Therefore, it is imperative to conduct preclinical experiments to elucidate its potential anticancer properties.
Conclusions
Application of extract from A. chinensis Planch radix induces ferroptosis in oxaliplatin-resistant CRC cells by altering gene expression profiles. Once the specific anticancer reagents are identified, it may be possible to apply treatments based on A. chinensis Planch radix in cancer therapy.
Acknowledgments
None.
Footnote
Data Sharing Statement: Available at https://tcr.amegroups.com/article/view/10.21037/tcr-2024-2567/dss
Peer Review File: Available at https://tcr.amegroups.com/article/view/10.21037/tcr-2024-2567/prf
Funding: This study was supported by
Conflicts of Interest: All authors have completed the ICMJE uniform disclosure form (available at https://tcr.amegroups.com/article/view/10.21037/tcr-2024-2567/coif). The authors have no conflicts of interest to declare.
Ethical Statement: The authors are accountable for all aspects of the work in ensuring that questions related to the accuracy or integrity of any part of the work are appropriately investigated and resolved.
Open Access Statement: This is an Open Access article distributed in accordance with the Creative Commons Attribution-NonCommercial-NoDerivs 4.0 International License (CC BY-NC-ND 4.0), which permits the non-commercial replication and distribution of the article with the strict proviso that no changes or edits are made and the original work is properly cited (including links to both the formal publication through the relevant DOI and the license). See: https://creativecommons.org/licenses/by-nc-nd/4.0/.
References
- Kwendakwema CN, Hopkins T, Bell-Brown A, et al. Clinician perceptions on barriers and facilitators to 1-year surveillance colonoscopy completion in survivors of colorectal cancer. Cancer Med 2024;13:e70244. [Crossref] [PubMed]
- Sinicrope FA. Increasing Incidence of Early-Onset Colorectal Cancer. N Engl J Med 2022;386:1547-58. [Crossref] [PubMed]
- Benson AB, Venook AP, Al-Hawary MM, et al. Colon Cancer, Version 2.2021, NCCN Clinical Practice Guidelines in Oncology. J Natl Compr Canc Netw 2021;19:329-59. [Crossref] [PubMed]
- Van Cutsem E, Cervantes A, Adam R, et al. ESMO consensus guidelines for the management of patients with metastatic colorectal cancer. Ann Oncol 2016;27:1386-422. [Crossref] [PubMed]
- Moretto R, Vetere G, Carullo M, et al. Induction treatment with FOLFIRINOX or oxaliplatin-based doublet followed by long-course chemoradiotherapy and surgery in locally advanced rectal cancer. A systematic review and pooled analysis from phase II and III trials. Cancer Treat Rev 2024;130:102829. [Crossref] [PubMed]
- Aizawa F, Kajimoto H, Okabayashi A, et al. Statins ameliorate oxaliplatin- and paclitaxel-induced peripheral neuropathy via glutathione S-transferase. Neurochem Int 2024;180:105863. [Crossref] [PubMed]
- Van Schaeybroeck S, Allen WL, Turkington RC, et al. Implementing prognostic and predictive biomarkers in CRC clinical trials. Nat Rev Clin Oncol 2011;8:222-32. [Crossref] [PubMed]
- Song M, Huang S, Wu X, et al. UBR5 mediates colorectal cancer chemoresistance by attenuating ferroptosis via Lys 11 ubiquitin-dependent stabilization of Smad3-SLC7A11 signaling. Redox Biol 2024;76:103349. [Crossref] [PubMed]
- Fang T, Zhao Z, Yuan F, et al. Actinidia Chinensis Planch Root extract attenuates proliferation and metastasis of hepatocellular carcinoma by inhibiting the DLX2/TARBP2/JNK/AKT pathway. J Ethnopharmacol 2020;251:112529. [Crossref] [PubMed]
- Cheng QL, Li HL, Huang ZQ, et al. 2β, 3β, 23-trihydroxy-urs-12-ene-28-olic acid (TUA) isolated from Actinidia chinensis Radix inhibits NCI-H460 cell proliferation by decreasing NF-κB expression. Chem Biol Interact 2015;240:1-11. [Crossref] [PubMed]
- Liu B, Yan Y, Zhang L. Radix Actinidiae chinensis induces the autophagy and apoptosis in renal cell carcinoma cells. Eur J Med Res 2024;29:291. [Crossref] [PubMed]
- Peng S, Yao L, Zhu X, et al. Ultrasound combined with FeSO4 facilitated the occurrence of ferroptosis in Vibrio parahaemolyticus. Ultrasonics Sonochemistry 2024;111:107080. [Crossref] [PubMed]
- Li C, Cui K, Zhu X, et al. 8-weeks aerobic exercise ameliorates cognitive deficit and mitigates ferroptosis triggered by iron overload in the prefrontal cortex of APP (Swe)/PSEN (1dE9) mice through Xc(-)/GPx4 pathway. Front Neurosci 2024;18:1453582. [Crossref] [PubMed]
- Wang X, Zhou Y, Ning L, et al. Knockdown of ANXA10 induces ferroptosis by inhibiting autophagy-mediated TFRC degradation in colorectal cancer. Cell Death Dis 2023;14:588. [Crossref] [PubMed]
- Yang C, Zhang Y, Lin S, et al. Suppressing the KIF20A/NUAK1/Nrf2/GPX4 signaling pathway induces ferroptosis and enhances the sensitivity of colorectal cancer to oxaliplatin. Aging (Albany NY) 2021;13:13515-34. [Crossref] [PubMed]
- Lu J, Zhao H, Yu C, et al. Targeting Ubiquitin-Specific Protease 7 (USP7) in Cancer: A New Insight to Overcome Drug Resistance. Front Pharmacol 2021;12:648491. [Crossref] [PubMed]
- Lim CH, Fang XQ, Kang H, et al. ER Stress-Activated HSF1 Governs Cancer Cell Resistance to USP7 Inhibitor-Based Chemotherapy through the PERK Pathway. Int J Mol Sci 2024;25:2768. [Crossref] [PubMed]
- Yang C, Zhou Q, Li M, et al. Upregulation of CYP2S1 by oxaliplatin is associated with p53 status in colorectal cancer cell lines. Sci Rep 2016;6:33078. [Crossref] [PubMed]
- Ottaiano A, Santorsola M, Capuozzo M, et al. The prognostic role of p53 mutations in metastatic colorectal cancer: A systematic review and meta-analysis. Crit Rev Oncol Hematol 2023;186:104018. [Crossref] [PubMed]
- Sun W, Ge Y, Cui J, et al. Scutellarin resensitizes oxaliplatin-resistant colorectal cancer cells to oxaliplatin treatment through inhibition of PKM2. Mol Ther Oncolytics 2021;21:87-97. [Crossref] [PubMed]
- Gao Z, Deng G, Li Y, et al. Actinidia chinensis Planch prevents proliferation and migration of gastric cancer associated with apoptosis, ferroptosis activation and mesenchymal phenotype suppression. Biomed Pharmacother 2020;126:110092. [Crossref] [PubMed]
- Lin JF, Hu PS, Wang YY, et al. Phosphorylated NFS1 weakens oxaliplatin-based chemosensitivity of colorectal cancer by preventing PANoptosis. Signal Transduct Target Ther 2022;7:54. [Crossref] [PubMed]
- Fang T, Fang Y, Xu X, et al. Actinidia chinensis Planch root extract attenuates proliferation and metastasis of hepatocellular carcinoma by inhibiting epithelial-mesenchymal transition. J Ethnopharmacol 2019;231:474-85. [Crossref] [PubMed]
- Huo J, Qin F, Cai X, et al. Chinese medicine formula "Weikang Keli" induces autophagic cell death on human gastric cancer cell line SGC-7901. Phytomedicine 2013;20:159-65. [Crossref] [PubMed]
- Lv J, Wang L, Shen H, et al. Regulatory roles of OASL in lung cancer cell sensitivity to Actinidia chinensis Planch root extract (acRoots). Cell Biol Toxicol 2018;34:207-18. [Crossref] [PubMed]
(English Language Editor: J. Gray)