Biological and clinical significance of circulating mucosal-associated invariant T cells in lung cancer
Highlight box
Key findings
• Lower percentage and absolute values of the circulating mucosal-associated invariant T (cMAIT) cells in lung cancer patients may be due to their migration into tumor tissues.
What is known and what is new?
• MAIT cells play an important role in malignant diseases.
• This study deepened the knowledge on biological and clinical significance of cMAIT in lung cancer.
What is the implication, and what should change now?
• In lung cancer patients, cMAIT may exert anti-tumor or pro-tumor effects through migration into tumor tissues.
• The role of cMAIT in lung cancer patients warrants further exploration.
Introduction
Mucosal-associated invariant T (MAIT) cells constitute a subset of innate-like T cells and were so named because they were initially discovered in the intestinal mucosa (1). MAIT cells express semi-invariant T cell receptors (TCRs), which consist of a constant alpha (α) chain paired with a semi-invariant beta (β) chain. TCRs vary among species. In humans, the α chain is Va7.2-Ja33, and the β chain is Vβ13 and Vβ2 (2,3). TCRs operate by recognizing microbial riboflavin (vitamin B2) ligands bound to the major histocompatibility complex class I-related protein (MR1) (4). In addition to the semi-invariant TCRs, cytokine receptors, chemokine receptors, and the transcription factor promyelocytic leukemia, zinc finger protein is present on the surface of MAIT cells and determines their functions (5). When stimulated by microbial antigens, MAIT cells can be recognized and activated by both MR1-dependent and MR1-independent mechanisms. Once activated, they can promptly generate certain cytokines, such as interferon γ (IFN-γ) and tumor necrosis factor α (TNF-α) and produce granzymes and perforin to mediate cytotoxic effects (6,7).
MAIT cells play important roles in infections, immunity, and malignant diseases (8-17). The data on MAIT cell alterations in cancer are inconsistent. For example, compared to healthy controls, the levels of circulating MAIT cells (cMAIT) are generally lower in mucosal malignancies, such as colorectal and gastric cancers, than in non-mucosal tumors, such as thyroid cancer, liver cancer, and breast cancer (18,19), but also in bladder cancer (20). Lower percentages of cMAIT in colorectal cancer patients than in healthy controls may be caused by the migration of MAIT cells induced by chemokines. In turn, a negative correlation with the lymph node stage suggests that the levels of MAIT cells can reflect the tumor advancement (19). A study suggests that the ability of MAIT cells to secrete IFN-γ in colorectal cancer tissues is significantly lower than that in the adjacent tissues, possibly due to the influence of the tumor microenvironment (21). One study showed that MAIT cells play a protective role in a mouse colon cancer model and are involved in regulating the immune response to cancer (22).
The data on biological and clinical relevance of cMAIT in lung cancer are scarce and inconsistent. Some studies demonstrated significantly higher values of peripheral blood MAIT cells in lung cancer patients than in healthy controls, while others reported the opposite (22,23). There were also differences in the serum levels of some cytokines, e.g., interleukin-6 (IL-6), interleukin-8 (IL-8), and IFN-γ between lung cancer patients and healthy controls (19,23) and the numbers of MAIT cells in lung cancer and adjacent tissues (24,25).
Increased baseline neutrophil-to-lymphocyte ratio (NLR) was shown to carry adverse prognosis in several malignancies (26,27). In tumors of mucosal origin, the negative correlation between cMAIT levels and lymph node stage suggests their relationship with tumor advancement and prognosis (19). There is no data on the relationship between cMAIT and the NLR and on the prognostic value of this correlation in lung cancer. MAIT cell subpopulations have been found to predict the efficacy of immunotherapy, similarly to the expression level of programmed cell death-ligand 1 (PD-L1) in tumor tissue (24,28). The relationship between cMAIT and PD-L1 expression in tumor tissue, as well as their predictive value to immunotherapy efficacy, has not been investigated.
This study used flow cytometry to detect the number of MAIT cells and their subpopulations in the blood and lung cancer tissues and analyzed their correlation with clinical indicators, NLR, and tissue PD-L1 expression. We aimed to explore the alterations in cMAIT in lung cancer and their clinical significance. Additionally, we assayed by enzyme-linked immunosorbent assay (ELISA) plasma levels of IL-6, IL-8, and IFN-γ in lung cancer patients and healthy controls to better understand the role of cMAIT in lung cancer. We present this article in accordance with the STROBE reporting checklist (available at https://tcr.amegroups.com/article/view/10.21037/tcr-2025-178/rc).
Methods
Study subjects
The study group included 30 lung cancer patients admitted to The First Affiliated Hospital of Zhengzhou University between December 2022 and March 2023 and 30 healthy control subjects (age and gender-matched). Inclusion criteria included newly diagnosed lung cancer confirmed by histopathology, at least one measurable lesion on imaging, age between 18 and 80 years, a life expectancy >3 months, and the Eastern Cooperative Oncology Group score of 0–1. Exclusion criteria included immune deficiency, autoimmune disease or immunotherapy administration, a secondary lung cancer or other malignancies, recent history of fever and infection, bronchial asthma, a serious cardiovascular disease (including coronary heart disease, abnormal electrocardiogram results, and uncontrolled hypertension), severe lung function impairment, and serious liver and kidney function insufficiency. The clinical and demographic patient data, including age, sex, laboratory tests, imaging and pathology, were retrieved from the Jiahe medical records system. The study was conducted in accordance with the Declaration of Helsinki (as revised in 2013). This study was approved by the Clinical Trial Ethics Committee of The First Affiliated Hospital of Zhengzhou University (No. 2025-KY-0302-002). All patients have signed informed consent for this study.
Experimental procedure
Principal reagent
The principal experimental reagents are detailed in supplementary materials (Table S1).
To prepare the complete medium, the following steps were performed:
The fetal bovine serum was removed from the –20 ℃ refrigerator and allowed to thaw at room temperature.
The necessary materials [i.e., basic Roswell Park Memorial Institute-1640 (RPMI-1640) penicillin-streptomycin, a 500-mL sterile glass bottle, a pipette gun, and a pipette gun tip] were placed on a super-clean table under an ultraviolet lamp for disinfection for about 20–30 minutes.
180 mL of the RPMI-1640 basic medium, 20 mL of fetal bovine serum, and 2 mL of 100 × penicillin-streptomycin double antibody were added to the 500-mL aseptic culture bottle, and the mixture was thoroughly mixed by slow shaking.
The prepared complete medium was divided into 50-mL centrifuge tubes, marked with the name and date, stored in a refrigerator at 4 ℃, and used within 2 weeks.
Peripheral blood mononuclear cell (PBMC) extraction
- Fasting peripheral venous blood samples from newly diagnosed lung cancer patients and healthy controls were collected with 5 mL ethylene diamine tetraacetic acid.
- The collected whole blood samples were centrifuged as follows: rise 5, fall 5, 25 ℃, and 2,500 r/minute for 10 minutes. After centrifugation, the whole blood was divided into the upper part (comprising light yellow plasma) and the lower part (comprising blood cells). The upper plasma was absorbed with a 2-mL pipette into a 1.5-mL Eppendorf (EP) tube, and placed in the refrigerator at –80 ℃ for subsequent cytokine detection
- The remaining blood was sucked and mixed with a pipette, then transferred to a 15-mL centrifuge tube, and an equal amount of phosphate buffered saline (PBS) was added to dilute the blood.
- Lymphocyte separation fluid was added to another 15-mL centrifuge tube. The ratio of the volume of lymphocyte separation fluid to blood volume before dilution was 2:1.
- The diluted blood was drawn and mixed with a straw. The centrifuge tube containing the lymphocyte separation solution was tilted slightly, a blood sample was drawn, and the tip of the straw was pushed against the wall of the tube and slowly dropped into the surface of the separation solution, trying not to let the blood drip into the separation solution.
- Centrifugation was performed as follows: rise 5, fall 5, 25 ℃, and 2,500 r/minute for 25 minutes.
- After centrifugation, the fluid in the tube was clearly stratified from top to bottom into plasma, PBMCs, lymphocyte isolation fluid, and the red blood cell layer. The PBMCs in a cloud shape were collected with a straw, placed in another 15-mL centrifuge tube, and washed with 10 mL of 1×PBS buffer.
- Centrifugation was performed as follows: rise 9, fall 9, 25 ℃, and 2,000 r/minute for 5 minutes.
- The supernatant was discarded and left to precipitate. The precipitates are PBMC and other components, such as plasma. Another 10 mL of 1×PBS buffer was added to the precipitate, and step 8 was repeated.
- The sediment in the tube was PBMCs, and if the precipitate was visibly red, it was cleaved by adding a Red Blood Cell Lysis Buffer.
- Depending on the volume of sediment, 1–2 mL of 1×PBS buffer was added, and the PBMCs were evenly distributed in the buffer by blowing with a straw and then transferred to a 1.5-mL EP tube for the subsequent treatment. If freeze storage was necessary, 1 or 2 mL of cell freeze storage solution was added to PBMCs. The PBMCs mixed with the cell freeze solution were blown with a straw to mix well and then transferred to a 2 mL freeze tube. The freeze storage tube was placed in a gradient cooling box, which was then placed in the refrigerator at –80 ℃. Resuscitation was required for use.
Structure treating
- 5–10 mg of fresh lung cancer tissue and adjacent normal lung tissue were taken and stored in 10 mL of RPMI-1640 at 4 ℃.
- The tissue was washed twice with 10 mL of complete culture medium containing penicillin and streptomycin. The washing times were adjusted appropriately according to the tissue color until the tissue was gray-white, and no obvious red color was observable by the naked eye. The tissue was then cut into small pieces to destroy the tissue mechanically. Next, a small amount of RPMI-1640 was used to wash the scissors, and the flushing solution was collected in a sample tube to avoid any tissue attachment to the scissors and the loss of target cells.
- The medium used to prepare the digestive tissue was added to the RPMI-1640 with 2% fetal bovine serum, 2 mg/mL type IV collagenase, and 0.05 mg/mL DNase I. The tube mouth was sealed with a sealing film. The tissue in the tube was digested in a water bath at 37 ℃ for 60 minutes and shaken well every 15–20 minutes.
- After mechanical and enzymatic digestion of the tissue, the single-cell suspension was obtained. A 70 µm sterile cell filter was moistened with RPMI-1640 in advance and filtered after moistening.
Preparation before flow cytometry
- 300 µL of the prepared sample was placed in a 1.5-mL EP tube, and the remaining samples were kept for later use.
- In each sample, 1 µL of Live-Dead Cell dye was added to mark the dead cells, and 1 µL of the following antibodies were then added: fluorescein isothiocyanate-cluster of differentiation 3 (FITC-CD3), phycoerythrin-TCR/γδ (PE-TCR/γδ), allophycocyanin-TCR Vα7.2 (APC-TCRVα7.2), Brilliant Violet 605-cluster of differentiation 161 (BV605-CD161), phycoerythrin/cyanine 7-cluster of differentiation 4 (PE/Cy7-CD4), and allophycocyanin/cyanine 7-cluster of differentiation 8 (APC/Cy7-CD8). The tube wall was finger-tapped several times to mix the sample with the antibody.
- After incubation at room temperature for 15 minutes in the dark, the target cells in the sample were fully bound to the labeled antibody.
ELISA
The plasma levels of IL-6, IL-8, and IFN-γ of lung cancer patients and healthy controls were measured by ELISA.
Preparations before operation:
- Take the tested plasma out of the −80 ℃ refrigerator and fully thaw at room temperature before use.
- Take the ELISA kit out of the 4 ℃ refrigerator and place it at room temperature for 20–30 min.
- Open the enzyme marker and the connected computer, open the SoftMax Pro6.4.2 software, and set the temperature to 37 ℃ for preheating.
Operation process:
- Take out the required slats according to the number of samples to be tested.
- Set blank holes, standard holes and sample holes to be measured on the slats, and add samples according to the instructions.
- Cover each sample hole with sealing plate film and incubate at 37 ℃ for 2 hours.
- Washing: gently uncover the sealing plate film, pour out the liquid in the hole, and pat it on the absorbent paper to dry it. Wash each sample hole with washing liquid, pour out the liquid in the hole after washing, pat it on the absorbent paper to dry it, repeat washing 4 times.
- Add 100 µL antibody (1×) to each sample hole, cover with sealing plate film, and incubate at 37 ℃ for 1 hour.
- Washing: same as step 4.
- Add 100 µL horseradish peroxidase (HRP) labeled secondary antibody (1×) to each sample hole, cover with sealing plate film, and incubate at 37 ℃ for 40 min.
- Washing: same as step 4.
- Color development: add 100 µL of tetramethylbenzidine (TMB) color development solution to each sample hole, and incubate at 37 ℃ for 15–20 minutes in the dark state for color development.
- Termination reaction: add 100 µL termination solution to each sample adding hole, and the liquid in the hole can be observed to change from blue to yellow.
- Measurement: Put the strip to be measured into the enzyme label instrument within 5 minutes after adding the termination solution, set the wavelength to 450 nm, and measure the optical density (OD) of each hole.
- Drawing of the standard curve: the OD value of the standard product is subtracted from the OD value of the blank hole, and the average value of the two multiple holes is the final value. With the standard concentration of each gradient as the horizontal coordinate and OD value as the vertical coordinate, the standard curve can be obtained by using ELISA Calc software for four-parameter fitting.
- Calculation of sample concentration: the OD value of the sample is substituted into the standard curve to obtain its fitting concentration, and the actual concentration of the sample can be obtained by multiplying its dilution.
Data synthesis and statistical analysis
All experimental operations were performed independently by three researchers and the average values were taken as the result to eliminate potential bias. The absolute values of MAIT cells were calculated as follows: absolute value of MAIT cells = absolute value of lymphocytes × MAIT/lymphocytes (%).
SPSS version 25.0 (IBM company) and GraphPad Prism version 8.0.2 (GraphPad Software) were used for the statistical analysis. The data were expressed as the mean ± standard error of the mean (SEM). An independent or paired sample t test was used for comparisons between two groups, and a Pearson correlation analysis was used for the correlation analysis. A one-way analysis of variance was used to compare the means of multiple groups. A P value <0.05 indicated a statistically significant difference. The flow diagram was analyzed and processed using Cytexpert for Dxflex version 2.0 (Beckman Coulter, Inc.) and FlowJo version 10.6 (BD Biosciences).
Results
Patients
Study group included 30 lung cancer patients (six, seventeen and seven with squamous cell, adenocarcinoma and small cell carcinoma, respectively); 21 males and nine females, a mean age of 63 years. The control group included age and gender matched 30 healthy subjects (Table 1).
Table 1
Characteristics | Lung cancer group (n=30) | Control group (n=30) |
---|---|---|
Age (years) (mean ± SD) | 63.0±7.7 | 56.9±7.4 |
Gender, n (%) | ||
Male | 21 (70.0) | 19 (63.3) |
Female | 9 (30.0) | 11 (36.7) |
Pathological pattern, n (%) | ||
Squamous-cell carcinoma | 6 (20.0) | – |
Adenocarcinoma | 17 (56.7) | – |
Small cell lung cancer | 7 (23.3) | – |
Clinical stage, n (%) | ||
I | 3 (10.0) | – |
II | 1 (3.33) | – |
III | 16 (53.3) | – |
IV | 10 (33.3) | – |
Gene mutation type, n (%) | ||
EGFR19 or 21 exon mutation | 6 (20.0) | – |
ALK gene fusion | 1 (3.33) | – |
KRAS mutation | 2 (6.67) | – |
PD-L1 expression, n (%) | ||
<1% | 5 (16.67) | – |
1–49% | 4 (13.33) | – |
>50% | 3 (10.0) | – |
EGFR, epidermal growth factor receptor; ALK, anaplastic lymphoma kinase; KRAS, kirsten rat sarcoma viral oncogene; PD-L1, programmed cell death-ligand 1; SD, standard deviation.
cMAIT in healthy controls
cMAIT were defined as the T cells showing positive expression of CD3 and negative expression of TCRγδ, positive expression of TCR Va7.2, and strong expression of CD161, i.e., CD3+ TCRγδ–TCR Va7.2+ CD161++ T cells. The lymphocyte population was first identified out of the entire cell populations and the CD3+ T cell population was then identified out of the lymphocyte population (Figure 1A). Hence, the gating was gradual until the cell group CD3+ TCRγδ–TCR Va7.2+ CD161++ T cells (i.e., MAIT cells) were finally identified. In healthy controls, both the percentage and absolute values of cMAIT were negatively correlated with age (percentage: r=–0.628; P<0.001, absolute value: r=–0.432; P=0.02) (Figure 1B) but not with gender (percentages: male 3.98%, female 4.88%; P=0.41, absolute values: 1.24×104/mL vs. 0.96×104/mL, respectively; P=0.27) (Figure 1C).
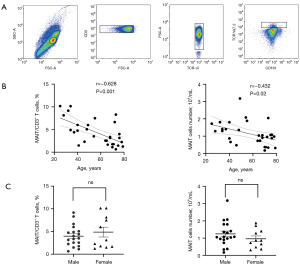
The percentage and absolute values of cMAIT in lung cancer patients vs. healthy controls
The percentage of cMAIT among the CD3+T cells was significantly lower in lung cancer patients than in healthy controls (2.11% vs. 4.31%, P<0.001) (Figure 2A). The same was true for the absolute values of the cMAIT (0.72×104/mL vs. 1.14×104/mL in lung cancer patients healthy controls, respectively; P=0.008) (Figure 2B).
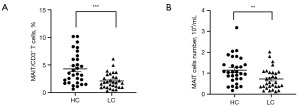
Comparison of the percentages of MAIT cells in tumor tissue and adjacent tissues
In this experiment, lung cancer tissues and corresponding adjacent tissues were collected and prepared into a single-cell suspension, which was combined with antibodies. The MAIT cells among the CD3+ T cells were identified by flow cytometry. The percentage of MAIT cells was higher in cancer tissues than in the adjacent tissues (1.44% vs. 1.29%, P=0.044) (Figure 3).
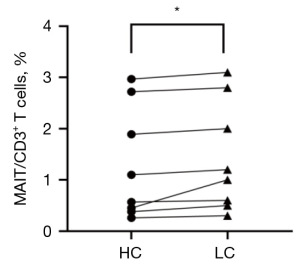
Analysis of the cMAIT cell subsets
According to the expression of CD4 and CD8 on the cell surface, MAIT cells were divided into the CD8+CD4–, CD8–CD4+, CD8+CD4+, and CD8–CD4– subgroups. The cMAIT of lung cancer patients and healthy controls mainly comprised the CD8+ CD4– subsets (80.4% vs. 83.6%; P=0.63) (Figure 4A). However, the absolute values of cMAIT were lower in lung cancer patients (0.58×104/mL vs. 0.92×104/mL, respectively; P=0.01) (Figure 4B).
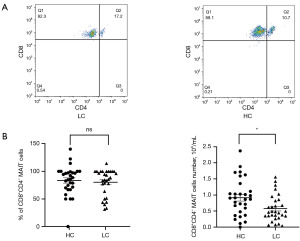
Relationship between the percentage and absolute values of cMAIT and other variables in lung cancer patients
The percentage of cMAIT in lung cancer patients was negatively correlated with age (r=–0.371, P=0.044) and positively correlated with blood albumin level (r=0.458, P=0.01) (Table 2). The absolute values of cMAIT in lung cancer patients were negatively correlated with age (r=–0.658; P<0.001) and positively correlated with blood albumin level (r=0.396; P=0.03) (Table 3).
Table 2
Clinical variable | Correlation coefficient (γs) | P value |
---|---|---|
Age | –0.371 | 0.044 |
Neutrophil absolute value | –0.019 | 0.92 |
Lymphocyte absolute value | –0.117 | 0.54 |
Monocyte absolute value | 0.068 | 0.72 |
Hemoglobin | –0.034 | 0.86 |
Platelet count | 0.117 | 0.54 |
Glutamic-pyruvic transaminase | –0.041 | 0.83 |
Glutamic oxalacetic transaminase | –0.307 | 0.10 |
Total protein | 0.107 | 0.57 |
Albumin | 0.458 | 0.01 |
Globulin | –0.205 | 0.28 |
Carbamide | 0.069 | 0.72 |
Creatinine | 0.326 | 0.08 |
Total bilirubin | –0.069 | 0.72 |
Total cholesterol | –0.031 | 0.88 |
Triglyceride | –0.253 | 0.21 |
High-density lipoprotein | –0.111 | 0.59 |
Low-density lipoprotein | 0.064 | 0.75 |
CEA | 0.036 | 0.85 |
CYFRA21-1 | –0.290 | 0.13 |
NSE | 0.120 | 0.54 |
CEA, carcinoembryonic antigen; CYFRA21-1, cytokeratin 19 fragment antigen 21-1; MAIT, mucosal-associated invariant T; NSE, neuron-specific enolase.
Table 3
Clinical variable | Correlation coefficient (γs) | P value |
---|---|---|
Age | –0.658 | <0.001 |
Neutrophil absolute value | 0.038 | 0.84 |
Lymphocyte absolute value | 0.097 | 0.61 |
Monocyte absolute value | 0.240 | 0.20 |
Hemoglobin | 0.120 | 0.53 |
Platelet count | 0.055 | 0.77 |
Glutamic-pyruvic transaminase | –0.113 | 0.55 |
Glutamic oxalacetic transaminase | –0.276 | 0.14 |
Total protein | 0.121 | 0.53 |
Albumin | 0.396 | 0.03 |
Globulin | –0.151 | 0.42 |
Carbamide | –0.229 | 0.22 |
Creatinine | –0.019 | 0.92 |
Total bilirubin | –0.283 | 0.13 |
Total cholesterol | 0.099 | 0.63 |
Triglyceride | 0.135 | 0.51 |
High-density lipoprotein | –0.214 | 0.30 |
Low-density lipoprotein | 0.128 | 0.53 |
CEA | 0.267 | 0.16 |
CYFRA21-1 | –0.080 | 0.68 |
NSE | 0.089 | 0.65 |
CEA, carcinoembryonic antigen; CYFRA21-1, cytokeratin 19 fragment antigen 21-1; MAIT, mucosal-associated invariant T; NSE, neuron-specific enolase.
Relationship between the percentage of the cMAIT and tumor histology and stage in lung cancer patients
No significant correlation was found between the percentage of cMAIT and lung cancer histology (P>0.99 for all comparisons) and stage (2.77 vs. 2.20 for stages I–II and III–IV, respectively; P=0.43) (Figure 5).
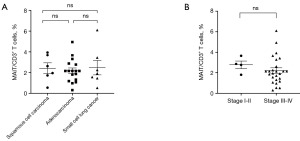
Relationship between the percentage of cMAIT and tumor size (T), lymph node metastasis (N), and distant metastasis (M) in lung cancer patients
Lung cancer patients were divided according to the tumor diameter (<3 and ≥3 cm), lymph node metastasis (N0 and N+), and the presence of distant metastasis (M0 and M1). There was no significant correlation between the percentage of cMAIT and tumor size (2.87 vs. 2.10, P=0.17), lymph node metastasis (2.91 vs. 2.30, P=0.39), and distant metastasis (2.51 vs. 1.93; P=0.29) (Figure 6).
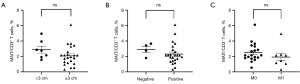
IL-6, IL-8, and IFN-γ levels in lung cancer patients and healthy controls
There were no significant differences in the IL-6, IL-8, and IFN-γ levels between lung cancer and control groups (IL-6: 13.00 vs. 12.14 pg/mL, P=0.63; IL-8: 29.95 vs. 37.63 pg/mL, P=0.052; and IFN-γ: 18.78 vs. 13.99 pg/mL, P=0.13) (Figure 7).
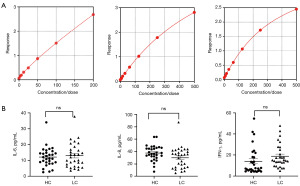
Relationship between the number of cMAIT and baseline NLR in lung cancer patients
A negative correlation was found between the percentage of cMAIT and the baseline NLR in lung cancer patients (r=–0.537; P=0.004) (Figure 8A). The absolute values of cMAIT in lung cancer patients were negatively correlated with the baseline NLR (r=–0.423; P=0.03) (Figure 8B).
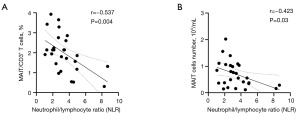
Relationship between cMAIT percentage and lung cancer PD-L1 expression
Lung cancer patients were divided into three groups according to the level of PD-L1 in the tumor tissues: <1% (negative-expression), 1–49% (low-expression), and ≥50% (high-expression). There was no significant correlation between the percentage of cMAIT and tumor PD-L1 expression (P=0.59) (Figure 9A) and the level of PD-L1 expression (P>0.99) (Figure 9B).
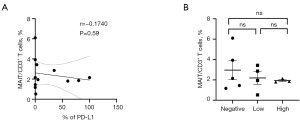
Discussion
This study analyzed the relationship between cMAIT values and other variables in lung cancer patients and healthy controls. Previous studies have demonstrated that the percentages of cMAIT in healthy subjects do not correlate with gender but decrease with age (29,30). Our study confirms these observations. The decreasing percentage of cMAIT with age may be related to the reduction of immune cells and the decline of immune functions.
In patients with infectious and autoimmune diseases, cMAIT participate and are consumed in anti-infection and autoimmunity processes, which decreases their number (31-34). In some malignancies, e.g., colorectal and gastric cancer, the values of cMAIT are lower than in healthy individuals, while the number of MAIT cells is higher in tumor than in healthy tissues. This phenomenon is presumed to be caused by cMAIT migrating to cancer tissues to play an immune role (31,32). The increased expression of chemokine receptors, such as C-C motif receptor 6 and C-X-C motif receptor 6 CCR6, on the surface of cMAIT, and the release of chemokines into peripheral blood by tumor tissues co-mediate the migration of cMAIT into tumor tissues (19).
Some studies showed significantly lower numbers of cMAIT in lung cancer patients than in healthy individuals, while others reported the opposite (19,23). A study has found increased levels of chemokines in the peripheral blood of lung cancer patients and higher numbers of MAIT cells in lung cancer tissues compared with adjacent tissues, but no significant differences in cell proliferation and apoptosis between these tissues (24), suggesting that cMAIT migrate to tumor tissues to play a tumor promoting roles. In the present study, the percentages and absolute values of cMAIT in lung cancer patients were lower than in healthy controls and the percentage of MAIT cells in lung cancer tissues was higher than that in the adjacent normal lung tissues. This suggests that a lower number of cMAIT in lung cancer patients may be caused by their migration into tumor tissues. The difference between this study and the study, “Participation of Increased Circulating MAIT cells in lung Cancer: a Pilot Study”, is that the innovation is that it demonstrates that cMAIT can migrate to the tissue to function by showing that lung cancer patients have fewer cMAIT and tumor tissues have more MAIT cells (23).
Of the four phenotypes of MAIT cells (CD4+CD8–, CD4+CD8+, CD4–CD8+, and CD4–CD8–), CD8+ phenotypes accounted for the highest proportion in the peripheral blood of healthy participants (33,34). CD8+ cMAIT are the most common in gastric cancer patients, but the numbers of CD8+CD4– cMAIT are lower than those in healthy controls (19). In lung cancer patients, the number of CD4+ and CD8+ cMAIT was found to be higher than in healthy controls, with no significant difference in the values of CD4– and CD8– cells between both groups (23). This suggests that the increase of CD4+ and CD8+ cMAIT may be related to immune dysfunction in lung cancer patients. The role of CD8 and CD4 cells among MAIT cells is unclear. Our study showed the highest proportion of CD8+CD4– subgroup among cMAIT in lung cancer patients. There was no significant difference in percentages of the CD8+CD4– subgroup between lung cancer patients and healthy subjects, but the absolute values of CD8+CD4– cells were lower in lung cancer patients. The specific role of this cell subgroup needs to be explored.
Several studies have analyzed the relationship between cMAIT and clinical variables in cancer patients. In tumors of mucosal origin, cMAIT were found to be negatively correlated with age, but the difference was insignificant (19). A negative correlation between cMAIT and lymph node stage has been attributed to their relationship with tumor extension (19). Additionally, in colorectal cancer, higher MAIT cell infiltration in tumor tissues was correlated with worse survival (35). In gastric cancer, no significant correlation was found between MAIT cell numbers and tumor stage (29). In non-small cell lung cancer, a study found lower percentages of tumor-infiltrating MAIT cells in patients with, compared to those without lymph node metastasis (25). The present study showed that the percentage and absolute values of cMAIT in lung cancer patients were negatively correlated with age, positively correlated with blood albumin levels, and not significantly correlated with tumor histology, tumor stage, and lung cancer serum markers [carcinoembryonic antigen (CEA); cytokeratin fragment 21-1 (CYFRA21-1); neuron-specific enolase (NSE)]. There is scarce knowledge on the correlation of cMAIT with blood albumin levels in cancer patients. The lack of correlation between cMAIT and lung cancer stage is consistent with the results of gastric cancer study (29).
Cytokines are active in many inflammatory processes and play a key role in the communication and connection of various cells. Cells often communicate with each other through cytokines. IL-8 can promote angiogenesis and the invasion and migration of tumor cells, and an increased IL-8 level is associated with tumor burden and poor prognosis (31,32). IL-6 not only promotes tumor proliferation but also plays an anti-tumor role. IFN-γ is an important anti-tumor factor that can act on natural killer cells and CD8 cytotoxic T cells. These cells can kill major histocompatibility complex-negative and positive tumor cells (36-38). The number of cMAIT and the plasma levels of IL-6, IL-8, and IFN-γ have been shown to be increased in lung cancer (23). Thus, MAIT cells may play a role in immunosuppression through the production or recruitment of IL-6, IL-8, and IFN-γ. cMAIT in lung cancer patients can produce IFN-γ, which in turn triggers an anti-tumor activity of lymphokine-activated killer cells. However, no significant difference was found in the plasma levels of IFN-γ, IL-17, and TNF-α in gastrointestinal cancers compared with healthy subjects (19). The present study showed no significant differences in plasma levels of IL-6, IL-8, and IFN-γ between lung cancer patients and healthy controls, which was inconsistent with the trend of decreasing cMAIT. cMAIT migrating into tissues can possibly still secrete these cytokines, but their role in lung cancer has not yet been determined. Thus, no conclusions can be drawn whether MAIT cells promote or prevent tumor growth.
In tumors of mucous origin, the values of cMAIT are negatively correlated with the lymph node stage (19). In solid tumors, including non-small and small cell lung cancer, higher NLR is an adverse prognostic factor (26,39,40). We showed the negative correlation between percentages and absolute values of cMAIT and NLR, which indirectly suggests their prognostic value in lung cancer. However, due to a short follow-up time we have not directly explored the relationship between MAIT cells and survival outcomes.
High expression of anti-PD-L1 has been shown to correlate with the efficacy of anti-PD-1 and PD-L1 immunotherapy (28). The subsets of MAIT cells with high expression of depletion genes and T helper-17-related genes were demonstrated to correlate with anti-PD-1 therapy resistance, while MAIT cells expressing genes related to cytotoxic function indicated the effectiveness of immunotherapy (24). These results suggest that cMAIT may predict the efficacy of anti-PD-1 agents (24). A study of non-small cell lung cancer showed that immune checkpoint blockade (ICB) of PD-1 or PD-L1 antibodies increased proliferation and co-expression of the activating markers HLA-DR and CD38 on MAIT cells in most patients after the first treatment cycle. The production of cytokines (especially TNF and IL-2) and the versatility of MAIT cells were also increased. It demonstrated that ICB improves the activation and function of cMAIT in patients with non-small cell lung cancer (41). Our study demonstrated a negative correlation between cMAIT and tissue PD-L1 in lung cancer patients, but the difference was insignificant, likely due to an insufficient number of patients.
To summarize, our results suggest that cMAIT may migrate from peripheral blood to lung cancer tissues. We also found that the percentages of cMAIT in lung cancer patients correlate with NLR and PD-L1 expression in tumor tissues, suggesting their relationship with immune efficacy.
This study has several limitations. First, the expression of cell proteins secreted by MAIT cells has not been assessed, so their function could not be fully clarified. Second, we have not analyzed chemokine receptors and thus have not provided further insight into the migration theory. Third, a short follow-up did not allow for a direct analysis of the relationship between MAIT cells and the prognosis. Hence, the function of MAIT cells warrants further exploration.
Conclusions
Weaker expression and lower absolute values of the cMAIT in lung cancer patients may be due to their migration into tissues. The possible prognostic value of MAIT cells warrants further studies. Elucidating the migration mechanism and revealing the interaction between MAIT cells and the tumor microenvironment may contribute to the development of lung cancer immunotherapy and provide new targets for this treatment.
Acknowledgments
None.
Footnote
Reporting Checklist: The authors have completed the STROBE reporting checklist. Available at https://tcr.amegroups.com/article/view/10.21037/tcr-2025-178/rc
Data Sharing Statement: Available at https://tcr.amegroups.com/article/view/10.21037/tcr-2025-178/dss
Peer Review File: Available at https://tcr.amegroups.com/article/view/10.21037/tcr-2025-178/prf
Funding: This study was supported by
Conflicts of Interest: All authors have completed the ICMJE uniform disclosure form (available at https://tcr.amegroups.com/article/view/10.21037/tcr-2025-178/coif). J.J. receives consulting fees from BMS, Roche, Novartis and MSD; payment or honoraria for lectures, presentations, speakers bureaus, manuscript writing or educational events from Pfizer, Novartis, Astra Zeneca and MSD; support for attending meetings and/or travel from Novartis, Pfizer and Takeda. The other authors have no conflicts of interest to declare.
Ethical Statement: The authors are accountable for all aspects of the work in ensuring that questions related to the accuracy or integrity of any part of the work are appropriately investigated and resolved. The study was conducted in accordance with the Declaration of Helsinki (as revised in 2013). This study was approved by the Clinical Trial Ethics Committee of The First Affiliated Hospital of Zhengzhou University (No. 2025-KY-0302-002). All patients have signed informed consent for this study.
Open Access Statement: This is an Open Access article distributed in accordance with the Creative Commons Attribution-NonCommercial-NoDerivs 4.0 International License (CC BY-NC-ND 4.0), which permits the non-commercial replication and distribution of the article with the strict proviso that no changes or edits are made and the original work is properly cited (including links to both the formal publication through the relevant DOI and the license). See: https://creativecommons.org/licenses/by-nc-nd/4.0/.
References
- Porcelli S, Yockey CE, Brenner MB, et al. Analysis of T cell antigen receptor (TCR) expression by human peripheral blood CD4-8- alpha/beta T cells demonstrates preferential use of several V beta genes and an invariant TCR alpha chain. J Exp Med 1993;178:1-16. [Crossref] [PubMed]
- McWilliam HEG, Villadangos JA. MR1 antigen presentation to MAIT cells and other MR1-restricted T cells. Nat Rev Immunol 2024;24:178-92. [Crossref] [PubMed]
- Petley EV, Koay HF, Henderson MA, et al. MAIT cells regulate NK cell-mediated tumor immunity. Nat Commun 2021;12:4746. [Crossref] [PubMed]
- Godfrey DI, Uldrich AP, McCluskey J, et al. The burgeoning family of unconventional T cells. Nat Immunol 2015;16:1114-23. [Crossref] [PubMed]
- Keller AN, Corbett AJ, Wubben JM, et al. MAIT cells and MR1-antigen recognition. Curr Opin Immunol 2017;46:66-74. [Crossref] [PubMed]
- Konecny AJ, Huang Y, Setty M, et al. Signals that control MAIT cell function in healthy and inflamed human tissues. Immunol Rev 2024;323:138-49. [Crossref] [PubMed]
- Kurioka A, Ussher JE, Cosgrove C, et al. MAIT cells are licensed through granzyme exchange to kill bacterially sensitized targets. Mucosal Immunol 2015;8:429-40. [Crossref] [PubMed]
- Provine NM, Klenerman P. MAIT Cells in Health and Disease. Annu Rev Immunol 2020;38:203-28. [Crossref] [PubMed]
- El Morr Y, Fürstenheim M, Mestdagh M, et al. MAIT cells monitor intestinal dysbiosis and contribute to host protection during colitis. Sci Immunol 2024;9:eadi8954. [Crossref] [PubMed]
- van Wilgenburg B, Loh L, Chen Z, et al. MAIT cells contribute to protection against lethal influenza infection in vivo. Nat Commun 2018;9:4706. [Crossref] [PubMed]
- D'Souza C, Pediongco T, Wang H, et al. Mucosal-Associated Invariant T Cells Augment Immunopathology and Gastritis in Chronic Helicobacter pylori Infection. J Immunol 2018;200:1901-16. [Crossref] [PubMed]
- Chiba A, Murayama G, Miyake S. Characteristics of mucosal-associated invariant T cells and their roles in immune diseases. Int Immunol 2021;33:775-80. [Crossref] [PubMed]
- Koppejan H, Jansen DTSL, Hameetman M, et al. Altered composition and phenotype of mucosal-associated invariant T cells in early untreated rheumatoid arthritis. Arthritis Res Ther 2019;21:3. [Crossref] [PubMed]
- Toussirot É, Laheurte C, Gaugler B, et al. Increased IL-22- and IL-17A-Producing Mucosal-Associated Invariant T Cells in the Peripheral Blood of Patients With Ankylosing Spondylitis. Front Immunol 2018;9:1610. [Crossref] [PubMed]
- Rouxel O, Da Silva J, Beaudoin L, et al. Cytotoxic and regulatory roles of mucosal-associated invariant T cells in type 1 diabetes. Nat Immunol 2017;18:1321-31. [Crossref] [PubMed]
- Liu Y, Zhang Q, Xing B, et al. Immune phenotypic linkage between colorectal cancer and liver metastasis. Cancer Cell 2022;40:424-437.e5. [Crossref] [PubMed]
- Jeffery HC, van Wilgenburg B, Kurioka A, et al. Biliary epithelium and liver B cells exposed to bacteria activate intrahepatic MAIT cells through MR1. J Hepatol 2016;64:1118-27. [Crossref] [PubMed]
- Huang WC, Hsiao YC, Wu CC, et al. Less circulating mucosal-associated invariant T cells in patients with cervical cancer. Taiwan J Obstet Gynecol 2019;58:117-21. [Crossref] [PubMed]
- Won EJ, Ju JK, Cho YN, et al. Clinical relevance of circulating mucosal-associated invariant T cell levels and their anti-cancer activity in patients with mucosal-associated cancer. Oncotarget 2016;7:76274-90. [Crossref] [PubMed]
- Zhang B, Chen P, Zhu J, et al. The quantity, function and anti-tumor effect of Mucosal associated invariant T cells in patients with bladder cancer. Int Immunopharmacol 2024;133:111892. [Crossref] [PubMed]
- Sundström P, Ahlmanner F, Akéus P, et al. Human Mucosa-Associated Invariant T Cells Accumulate in Colon Adenocarcinomas but Produce Reduced Amounts of IFN-γ. J Immunol 2015;195:3472-81. [Crossref] [PubMed]
- Cheng OJ, Lebish EJ, Jensen O, et al. Mucosal-associated invariant T cells modulate innate immune cells and inhibit colon cancer growth. Scand J Immunol 2024;100:e13391. [Crossref] [PubMed]
- Zhang Q, Li P, Zhou W, et al. Participation of Increased Circulating MAIT Cells in Lung Cancer: a Pilot Study. J Cancer 2022;13:1623-9. [Crossref] [PubMed]
- Shi L, Lu J, Zhong D, et al. Clinicopathological and predictive value of MAIT cells in non-small cell lung cancer for immunotherapy. J Immunother Cancer 2023;11:e005902. [Crossref] [PubMed]
- Ouyang L, Wu M, Zhao J, et al. Mucosal-associated invariant T cells reduce and display tissue-resident phenotype with elevated IL-17 producing capacity in non-small cell lung cancer. Int Immunopharmacol 2022;113:109461. [Crossref] [PubMed]
- Templeton AJ, McNamara MG, Šeruga B, et al. Prognostic role of neutrophil-to-lymphocyte ratio in solid tumors: a systematic review and meta-analysis. J Natl Cancer Inst 2014;106:dju124. [Crossref] [PubMed]
- Mi HL, Wei WL, Zhang DH, et al. Neutrophil-to-Lymphocyte Ratio, Platelet-to-Lymphocyte Ratio, and Prognostic Nutritional Index as Prognostic Markers for Lung Carcinoma. Br J Hosp Med (Lond) 2024;85:1-13. [Crossref] [PubMed]
- Hinterleitner C, Strähle J, Malenke E, et al. Platelet PD-L1 reflects collective intratumoral PD-L1 expression and predicts immunotherapy response in non-small cell lung cancer. Nat Commun 2021;12:7005. [Crossref] [PubMed]
- Shao CY. Frequency, phenotype and function of mucosa-associated constant T(MAIT) cells in peripheral blood of patients with gastric cancer. Anhui Medical University, 2020.
- Ardolino M, Hsu J, Raulet DH. Cytokine treatment in cancer immunotherapy. Oncotarget 2015;6:19346-7. [Crossref] [PubMed]
- Schalper KA, Carleton M, Zhou M, et al. Elevated serum interleukin-8 is associated with enhanced intratumor neutrophils and reduced clinical benefit of immune-checkpoint inhibitors. Nat Med 2020;26:688-92. [Crossref] [PubMed]
- Gonzalez-Aparicio M, Alfaro C. Significance of the IL-8 pathway for immunotherapy. Hum Vaccin Immunother 2020;16:2312-7. Retracted Publication. [Crossref] [PubMed]
- Dias J, Boulouis C, Gorin JB, et al. The CD4(-)CD8(-) MAIT cell subpopulation is a functionally distinct subset developmentally related to the main CD8(+) MAIT cell pool. Proc Natl Acad Sci U S A 2018;115:E11513-22. [Crossref] [PubMed]
- Zheng Y, Han F, Wu Z, et al. MAIT cell activation and recruitment in inflammation and tissue damage in acute appendicitis. Sci Adv 2024;10:eadn6331. [Crossref] [PubMed]
- Zabijak L, Attencourt C, Guignant C, et al. Increased tumor infiltration by mucosal-associated invariant T cells correlates with poor survival in colorectal cancer patients. Cancer Immunol Immunother 2015;64:1601-8. [Crossref] [PubMed]
- Fujii S, Shimizu K, Okamoto Y, et al. NKT cells as an ideal anti-tumor immunotherapeutic. Front Immunol 2013;4:409. [Crossref] [PubMed]
- Masjedi A, Hashemi V, Hojjat-Farsangi M, et al. The significant role of interleukin-6 and its signaling pathway in the immunopathogenesis and treatment of breast cancer. Biomed Pharmacother 2018;108:1415-24. [Crossref] [PubMed]
- Karachaliou N, Gonzalez-Cao M, Crespo G, et al. Interferon gamma, an important marker of response to immune checkpoint blockade in non-small cell lung cancer and melanoma patients. Ther Adv Med Oncol 2018;10:1758834017749748. [Crossref] [PubMed]
- Aloe C, Wang H, Vlahos R, et al. Emerging and multifaceted role of neutrophils in lung cancer. Transl Lung Cancer Res 2021;10:2806-18. [Crossref] [PubMed]
- Mirili C, Guney IB, Paydas S, et al. Prognostic significance of neutrophil/lymphocyte ratio (NLR) and correlation with PET-CT metabolic parameters in small cell lung cancer (SCLC). Int J Clin Oncol 2019;24:168-78. [Crossref] [PubMed]
- Sundström P, Dutta N, Rodin W, et al. Immune checkpoint blockade improves the activation and function of circulating mucosal-associated invariant T (MAIT) cells in patients with non-small cell lung cancer. Oncoimmunology 2024;13:2312631. [Crossref] [PubMed]