A narrative review of papillary thyroid carcinoma-related long non-coding RNAs and their relevance to malignant tumors
Introduction
Papillary thyroid carcinoma (PTC) represents the predominant histological subtype among thyroid cancer cases, constituting 89.1% of all instances (1). The incidence of thyroid cancer has witnessed a substantial rise in recent decades, primarily attributed to the heightened diagnosis of the PTC subtype (2). PTC, a well-differentiated subtype, has been reported to have the potential for progression towards a poorly differentiated state, resulting in a significant decrease in life expectancy (3,4). Due to our incomplete understanding of the molecular mechanisms underlying PTC progression, further research is imperative to uncover novel therapeutic targets.
Long non-coding RNAs (lncRNAs) are a class of transcripts exceeding 200 nucleotides in length with minimal or virtually no protein-coding capacity (5). Recent studies have revealed a pivotal role of lncRNAs in modulating the growth, invasion, and metastasis of colorectal cancer (6-9), hepatic cancer (10-16), breast cancer (17-19), etc. It is evident that lncRNAs play a significant role in the initiation and progression of various types of cancer through diverse molecular interaction mechanisms. We identified lncRNAs related to PTC that have been reported in the past 2 years and provided an overview of the roles and mechanisms of these lncRNAs in PTC, along with their roles and mechanisms in other cancers. This comprehensive exploration serves as a valuable resource for researchers seeking a thorough understanding of the latest PTC-associated lncRNAs. We present this article in accordance with the Narrative Review reporting checklist (available at https://tcr.amegroups.com/article/view/10.21037/tcr-24-1038/rc).
Methods
We conducted a review of articles published during the period from Jan 2022 to May 2024 pertaining to the relationship between lncRNAs and PTC. This ensured the novelty of the research object. We conducted a search in large literature databases such as PubMed, Web of Science, and Embase. The search terms for PubMed are: (PTC[Title/Abstract] OR “papillary thyroid”[Title/Abstract]) AND (lncRNA[Title/Abstract] OR “long non-coding RNA”[Title/Abstract]). For Web of Science, the search terms are: “PTC” OR “Papillary thyroid” (Topic) and lncRNA OR “long non-coding RNA” (Topic). In Embase, the search terms are: (ptc:ti,ab,kw OR ‘papillary thyroid’:ti,ab,kw) AND (lncrna:ti,ab,kw OR ‘long non-coding rna’:ti,ab,kw). The summary of the search strategy can be found in Table 1. Subsequently, we filtered out studies with low quality and those lacking experimental validation, focusing our review on the high-confidence PTC-associated lncRNAs that remained. We centered our research around these latest PTC-related lncRNAs, conducted searches for relevant articles exploring their relationships with various types of tumors, and analyzed their modes of regulation on tumors. We provided summaries for the key aspects and analyzed the patterns present in these studies. And specific regulatory axes of each lncRNA regulating PTC are presented in Figure 1.
Table 1
Items | Specification |
---|---|
Date of search | May 1st, 2024 |
Databases and other sources searched | PubMed, Web of Science, Embase |
Search terms used | “Thyroid Cancer, Papillary”, “RNA, Long Noncoding”, Papillary Thyroid Carcinoma, Carcinoma, Cancer |
Timeframe | From Jan 2022 to May 2024 |
Inclusion criteria | (I) The effects of lncRNA on PTC and other cancers have been experimentally validated |
(II) The methodology is rational and rigorous after undergoing a comprehensive evaluation | |
(III) The reporting is accurate and comprehensive, with no selective reporting | |
(IV) The article is written in English | |
Selection process | Y.S. and Y.J., independently conducted the literature search and assessment. Discrepancies were resolved through consultation |
lncRNA, long non-coding RNA; PTC, papillary thyroid carcinoma.
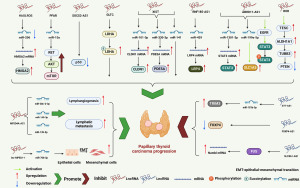
PTC-related lncRNAs
lncRNA HOXD antisense growth-associated lncRNA (HAGLROS)
HAGLR opposite strand lncRNA (HAGLROS) has only one transcript, a 699 bp lncRNA, according the National Center for Biotechnology Information (NCBI) tified (NR_110457.1). Studies have indicated that lncRNA HAGLROS may promote the malignant behavior of cancer cells, suggesting its potential involvement in cancer initiation and progression (20). All the currently retrieved studies consistently indicate that lncRNA HAGLROS plays a positive role in the development of malignant tumors. This is achieved through its interactions with various microRNAs (miRNAs), utilizing miRNAs as downstream targets, and influencing the expression of tumor-related proteins via miRNA modulation. The regulatory axes of each lncRNA in regulating tumors other than PTC are displayed in Table 2. Among the downstream miRNAs affected by HAGLROS, notable ones include miR-138-5p, miR-330-5p, miR-26b-5p, miR-100, miR-206, miR-26b-5p, miRNA-152, miR-5095, and miR-100-5p (21-31). HAGLROS’s regulatory impact extends to particular proteins or pathways, many of which are associated with apoptosis, such as Bcl-2, Bax, caspase-3, and NOTCH3. In the context of PTC, The study conducted by Mutlu Icduygu et al. indicates that HAGLROS exhibits higher expression levels in clinical samples of PTC (32). Specific regulatory axes of each lncRNA regulating PTC are presented in Table 3, Research conducted by Zeng and colleagues has revealed the existence of the HAGLROS/miR-206/HMGA2 pathway in PTC (33). Research on this type of lncRNA in the context of PTC is relatively limited. Existing studies generally suggest a positive role for it in the development of PTC. However, further research is needed to validate its credibility. The specific regulatory network can be seen in Figure 2.
Table 2
LncRNA | Cancer type | Type of interaction | Target miRNA | Regulated protein(s) |
---|---|---|---|---|
HAGLROS | Hepatocellular carcinoma | pos | miR-26b-5p | KPNA2 |
Hepatocellular carcinoma | pos | miR-5095 | ATG12 | |
Esophageal cancer | pos | miR-206 | NOTCH3 | |
Hepatocellular carcinoma | pos | miR-5095 | ATG12 | |
Gastric cancer | pos | miR-100-5p | mTOR | |
Lung carcinoma | pos | miR-100 | SMARCA5 | |
Lung carcinoma | pos | miRNA-152 | NA | |
Laryngeal cance | pos | miR-138-5p | CLN5 | |
TNBC | pos | miR-330-5p | PAX5 | |
Ovarian cancer | pos | miR-26b-5p | Ki67/Bcl-2/Bax/caspase-3 | |
Bladder cancer | pos | miR-330-5p | SPRR1B | |
LargeB-cell lymphoma | pos | miR-100 | NA | |
SOCS2-AS1 | Hepatocellular carcinoma | neg | miR-454-3p | CPEB1 |
Endometrial cancer | neg | NA | AURKA | |
Colorectal cancer | neg | miR-1264 | SOCS2 | |
Prostate cancer | pos | NA | Proteins related to the apoptosis | |
Glioma | pos | NA | ITGB1 | |
XIST | Esophageal cancer | pos | miR-101 | EZH2 |
Gastric cancer | pos | miR-101 | EZH2 | |
Colorectal cancer | pos | miR-137 | EZH2 | |
Laryngeal cancer | pos | miR-124 | EZH2 | |
Pancreatic cancer | pos | miR-140/124 | iASPP/CDK1 | |
Lung cancer | pos | miR-140 | iASPP | |
Pancreatic cancer | pos | miR-137 | Notch1 | |
Colorectal cancer | pos | miR-137 | PKM2/PKM1 | |
Lung cancer | pos | miR-137 | Notch1 | |
Glioma | pos | miR-137 | Rac1 | |
Glioma | pos | miR-137 | ZO-2/FOXC1 | |
Osteosarcoma | pos | miR-137 | NA | |
ST7-AS1 | Gastric cancer | pos | NA | EZH2 |
Gastric cancer | pos | NA | PI3K-AKT/EMT | |
Laryngeal carcinoma | pos | NA | CARM1/Sox-2 | |
FOXP4-AS1 | Hepatocellular carcinoma | pos | NA | EZH2/ZC3H12D |
Nasopharyngeal carcinoma | pos | miR-136-5p | MAPK1 | |
Ewing sarcoma | pos | NA | TME | |
Colorectal cancer | pos | miR-423-5p | NACC1 | |
Cervical cancer | pos | miR-136-5p | CBX4 | |
Lung cancer | pos | miR-3184-5p | EIF5A | |
Esophageal carcinoma | pos | NA | FOXP4/β-catenin | |
Prostate cancer | pos | miR-3184-5p | FOXP4 | |
Mantle cell lymphoma | pos | miR‑423‑5p | NACC1 | |
Osteosarcoma | pos | NA | LATS1 | |
SLC8A1-AS1 | Glioma | pos | NA | Wnt/β-catenin signaling pathway |
RNF185-AS1 | Hepatocellular carcinoma | pos | miR-221-5p | Integrin β5 |
ROR | Breast cancer | pos | miRNA-205 | ZEB1/ZEB2/vimentin/E-cadherin |
Gastric cancer | pos | NA | MRP1 | |
Gastric cancer | pos | miR-519d-3p | HMGA2 | |
Gastric cancer | pos | NA | OCT4/SOX2/NANOG | |
Colorectal cancer | pos | miR-6833-3p | SMC4 | |
Colorectal cancer | pos | NA | p53 | |
Colorectal cancer | pos | miR-223-3p | NF2 | |
Hepatocellular carcinoma | pos | miRNA-145 | RAD18 | |
Endometrial cancer | pos | miR-34a | Notch1 | |
Renal cancer | pos | NA | p53/c‑Myc | |
Osteosarcoma | pos | miR-153-3p | ABCB1 | |
Ovarian cancer | pos | miR-145 | FLNB-EMT | |
Esophageal cancer | pos | miR-145 | LMNB2 | |
Bladder cancer | pos | NA | ZEB1/EMT | |
Prostate cancer | pos | miR-145 | Oct4 | |
Gallbladder cancer | pos | NA | EMT | |
ABHD11-AS1 | Cervical cancer | pos | NA | ABHD11/EGFR |
Cervical carcinoma | pos | miR-1254 | NA | |
Cervical cancer | pos | miR-330-5p | MARK2 | |
Ovarian cancer | pos | miR-133a-3p | NA | |
Ovarian cancer | pos | NA | RhoC | |
Endometrial carcinoma | pos | NA | cyclin D1 | |
Triple-negative breast cancer | pos | miR-199a-5p | NA | |
Pancreatic cancer | pos | NA | EMT/PI3K-AKT pathway | |
Pancreatic cancer | pos | miR-1231 | cyclin E1 | |
Colorectal cancer | pos | miR-1254 | WNT11 | |
Colorectal cancer | pos | miR-133a | SOX4 | |
Colorectal cancer | pos | NA | ITGA5/Fak/PI3K/Akt | |
Gastric cancer | pos | miR-361-3p | PDPK1 |
neg: overexpression inhibits tumor progression. Decreased expression promotes tumor progression. pos: overexpression promotes tumor progression or increases resistance to radiotherapy and chemotherapy. Decreased expression inhibits tumor progression. ABHD11-AS1, alpha/beta hydrolase domain containing 11 antisense RNA 1; FOXP4-AS1, forkhead box P4 antisense RNA 1; HAGLROS, HOXD antisense growth-associated lncRNA; lncRNA, long non-coding RNA; NA, not involved or not specified; PTC, papillary thyroid carcinoma; RNF185-AS1, ring finger protein 185 antisense RNA 1; ROR, regulator of reprogramming; SLC8A1-AS1, solute carrier family 8 member A1 antisense RNA 1; SOCS2-AS1, suppressor of cytokine signaling 2-antisense transcript 1; ST7-AS1, suppressing tumorigenicity 7 antisense RNA 1; XIST, X inactivate-specific transcript.
Table 3
LncRNA | Type of interaction | Target miRNA | Regulated protein(s) |
---|---|---|---|
HAGLROS | pos | miR-206 | HMGA2 |
PFAR | pos | miR-15a | RET-AKT-mTOR |
SOCS2-AS1 | pos | NA | p53 |
GLTC | pos | NA | LDHA |
XIST | pos | miR-101-3p | CLDN1 |
pos | miR-330-3p | PDE5A | |
pos | miR-141 | NA | |
MFSD4A-AS1 | pos | miR-30c-2-3p/miR-145-3p/miR-139-5p | NA |
ST7-AS1 | neg | miR-181b-5p | TRIM3 |
FOXP4-AS1 | neg | NA | AKT signaling pathway |
SLC8A1-AS1 | neg | NA | FUS/Numbl |
lnc-MPEG1-1 | pos | miR-766-5p | EMT |
RNF185-AS1 | pos | miR-429 | LRP4 |
ROR | pos | NA | TESC/ALDH1A1/TUBB3/PTEN |
ABHD11-AS1 | pos | NA | EPS15L1/EGFR signaling pathway |
pos | miR-199a-5p | SLC1A5 | |
pos | miR-1301-3p | STAT3 |
neg: overexpression inhibits tumor progression. Decreased expression promotes tumor progression. pos: overexpression promotes tumor progression or increases resistance to radiotherapy and chemotherapy. Decreased expression inhibits tumor progression. ABHD11-AS1, alpha/beta hydrolase domain containing 11 antisense RNA 1; FOXP4-AS1, forkhead box P4 antisense RNA 1; HAGLROS, HOXD antisense growth-associated lncRNA; lncRNA, long non-coding RNA; NA, not involved or not specified; PTC, papillary thyroid carcinoma; RNF185-AS1, ring finger protein 185 antisense RNA 1; ROR, regulator of reprogramming; SLC8A1-AS1, solute carrier family 8 member A1 antisense RNA 1; SOCS2-AS1, suppressor of cytokine signaling 2-antisense transcript 1; ST7-AS1, suppressing tumorigenicity 7 antisense RNA 1; XIST, X inactivate-specific transcript.
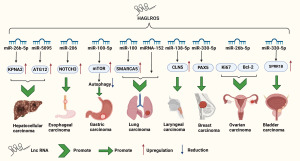
In summary, we have observed that lncRNA HAGLROS primarily exerts its influence on cancer through interactions with downstream miRNAs, acting as a miRNA sponge to modulate the expression of miRNA target proteins, ultimately promoting cancer development. Notably, miRNA-330-5p and miR-100 are targeted by HAGLROS. This underscores the significance of these two classes of miRNAs in the context of HAGLROS-mediated oncogenic effects.
lncRNA pancreatic functional islet-associated regulator (PFAR)
Pancreatic fibrosis-associated lncRNA (lnc-PFAR) facilitates autophagy and exacerbates pancreatic fibrosis (34). Fang’s research proposed that lncRNA PFAR acts as a sponge for miR-15a, leading to the upregulation of RET and consequently promoting the activation of the AKT/mTOR signaling pathway, thereby facilitating the progression of PTC (35). Activation of RET was found closely related to thyroid cancer (36), found to be targeted by miR-15a (37), found to contribute to the progression of cancer by triggering the AKT/mTOR signaling pathway (38). Akt/mTOR pathway was reported to inhibit autophagy (39,40).
In summary, lncRNA PFAR orchestrates a regulatory axis by modulating miR-15a, which in turn targets RET. This interaction results in the activation of RET, subsequently triggering the AKT/mTOR signaling pathway. Ultimately, this cascade leads to the inhibition of apoptosis in PTC cells and promotes PTC progression. The establishment of this regulatory axis appears to be robust and reliable. However, there is currently no other research indicating the role of lncRNA PFAR in the development of PTC, nor is there evidence to suggest its specific involvement in the occurrence of other cancers. Therefore, there is significant room for exploration in this field.
lncRNA suppressor of cytokine signaling 2-antisense transcript 1 (SOCS2-AS1)
LncRNA SOCS2-AS1 is situated on the reverse strand of chromosome 12. In recent years, complex associations have been unveiled between SOCS2-AS1 and various types of cancer, exhibiting both promotive and inhibitory roles in cancer development and progression. SOCS2-AS1 promotes the progression of glioma by upregulating the expression of integrin subunit beta 1 (ITGB1), which plays an essential role in various cellular functions, including the regulation of cell proliferation, thereby influencing biological processes such as immune responses and carcinogenesis (41-43). It also plays a positive role in the development of castration-resistant prostate cancer by inhibiting apoptosis (44), Specifically, SOCS2-AS1 modulates the expression of genes associated with apoptosis, such as TNFSF10 (45). Additionally, the study revealed that silencing SOCS2-AS1 downregulates oncogenic genes, namely forkhead box protein M1 (FOXM1) and its downstream target, centromere protein F (CENPF). Co-expression of FOXM1 and CENPF has been linked to the activation of the PI3K and MAPK signaling pathways, thus playing a role in cell growth and migration processes (46,47). These findings lay the groundwork for further research in this area. In addition to its promoting role in cancer development mentioned above, SOCS2-AS1 exhibits inhibitory effects on several types of cancers, SOCS2-AS1 exerts its inhibitory effects on colorectal cancer progression and metastasis by stabilizing SOCS2 and acting as a sponge for miR-1264 (48), facilitating the expression of SOCS2, which is a tumor suppressor to inhibit proliferation and invasion of human cancer (49,50). In hepatocellular carcinoma (HCC), SOCS2-AS1 regulates miR-454-3p, which in turn affects the expression of CPEB1, which inhibits the proliferation of glioblastoma cells and regulates the differentiation of glioma stem cells (51,52), ultimately leading to the suppression of cancer stem cell properties and progression in liver cancer cells (53). LncRNA SOCS2-AS1 also promotes the degradation of AURKA by enhancing the interaction between FBXW7 and AURKA, which is frequently amplified in various tumors (54-56), associated with a poor prognosis, thereby inhibiting the growth and metastasis of endometrial cancer (57). In Glioma, the expression of SOCS2-AS1 is positively correlated with the expression levels of the core factor ITGB1 in the extracellular matrix (ECM)-receptor interaction signaling pathway, which plays an essential role in numerous cellular functions, including the control of cell proliferation (58). Regarding the role of this lncRNA in PTC, a study conducted by Zhang et al. proposed that the high expression of SOCS2-AS1 in PTC enhances the degradation of p53, and promotes PTC cell proliferation and the fatty acid oxidation rate (59).
In summary, lncRNA SOCS2-AS1 exhibits a promotive role in cancer development in glioma and prostate cancer, while it exerts inhibitory effects in HCC, endometrial cancer, and colorectal cancer. In HCC and colorectal cancer, its impact on cancer is mediated through post-regulation of downstream protein expression by interacting with miR-454-3p and miR-1264, respectively. Although other studies have suggested the regulation of specific proteins by lncRNA SOCS2-AS1, but whether a miRNA is involved in this process and which miRNA it is remains unclear. Therefore, this represents a subject worthy of further investigation in the future.
lncRNA gamma-glutamyltransferase containing (GLTC)
Previously, the role of the lncRNA GLTC in the development of cancer had not been established. However, a recent study has proposed that GLTC is an oncogenic lncRNA in PTC. This finding has been rigorously validated through experimental investigations. The study revealed that GLTC promotes cell growth and glycolysis in cultured PTC cells and in a mouse xenograft tumor model (60). LDHA is an oncogenic metabolic enzyme that initiates a phenotypic shift from mitochondrial respiration to glycolysis (61,62). Elevated levels of LDHA messenger RNA (mRNA) and protein significantly impact the progression of PTC (63). Data from the TCGA database confirm LDHA as a potential diagnostic and therapeutic target in PTC (64). The study by Shi et al. further demonstrated that GLTC increases the succinylation of LDHA at the K155 site, enhancing LDHA activity, thus promoting the progression of PTC (60,65). Furthermore, the enhanced LDHA activity mediated by GLTC contributes to the development of resistance to radioiodine therapy in PTC, a phenomenon observed both in vitro and in vivo (60). The role of LncRNA GLTC in PTC or cancer is still a relatively new field of study, and the research conducted by Shi and colleagues holds significant value. It can serve as a valuable reference for future studies in this area.
lncRNA X inactivate-specific transcript (XIST)
XIST is an identified lncRNA which has been found to be highly expressed in various types of cancers, including digestive system cancers; respiratory system cancers; nervous system tumors such as glioma and neuroblastoma; other system tumors such as sarcomas, bladder cancer, cervical cancer and melanoma. In these contexts, XIST exerts its influence by modulating specific miRNAs, which, in turn, regulate the expression of cancer-related target genes, ultimately contributing to tumor progression (66). In the regulatory networks, we have observed the recurrence of certain key genes. For instance, in esophageal cancer, gastric cancer, colorectal cancer, and laryngeal cancer, XIST consistently emerges as a pivotal factor influencing the expression of the EZH2 gene (67-70), which plays a critical role in the regulation of cell proliferation, migration, invasion, tumorigenesis and metastasis, ultimately driving cancer progression (71,72). In both colorectal cancer and pancreatic cancer, XIST ultimately promotes tumor progression by regulating the expression of the ZEB1 gene (73,74), which is an important regulator of epithelial-mesenchymal transition (EMT) and cell invasion in different tumor types (75,76), and in lung cancer, pancreatic cancer and osteosarcoma, XIST ultimately promotes tumor progression by regulating the expression of the iASPP gene (77-79), which has been discovered to function as an oncogene (80,81). The recurrent presence of these genes in the regulatory network of XIST underscores their significance. Furthermore, several key miRNAs have also been found to recur within the regulatory network governed by XIST. In colorectal cancer (69,82), pancreatic cancer (83), lung cancer (84), glioma (85,86), and osteosarcoma (87), XIST exerts its influence by targeting miR-137. In esophageal cancer, gastric cancer, retinoblastoma, XIST exerts its influence by targeting miR-101 (68,88,89). This also underscores the crucial role of these miRNAs in XIST-mediated regulation of malignant tumors. In addition to its promoting role, studies have also reported that XIST exhibits anti-tumorigenic properties in lymphoma (90) and contradictory effects within the same type of cancer (91,92). These studies indicate that lncRNA XIST has complex network mechanisms in the regulation of cancer, and the majority of research suggests its role in promoting cancer progression, while its role in PTC is less studied. Two studies currently demonstrate the involvement of lncRNA XIST in the occurrence and progression of PTC. Du et al. proposed in their study that XIST can upregulate CLDN1 by sequestering miR-101-3p, thereby promoting the migration and invasion of PTC cells (93). MiR-101-3p, known as a tumor-suppressive miRNA (94-96), and CLDN1, a tight junction protein associated with cancer proliferation, migration, and invasion, play key roles in this regulatory mechanism (97). In addition to the aforementioned studies, Cai et al. proposed that XIST enhances the malignant behavior of PTC by inhibiting miR-330-3p, thereby increasing the expression of PDE5A (98), which was recently demonstrated as a downstream target of BRAF (99). Activated BRAF downregulates the expression of PDE5A, resulting in reduced degradation of cGMP, leading to an increase in intracellular calcium ion concentration, triggering the invasion and metastasis of tumor cells (100). Xu et al. suggested that XIST functions as an oncogene in PTC progression by regulating miR-141. However, they did not provide information about the downstream genes affected or whether specific genes need to be targeted, which is a gap that needs to be addressed (101). The specific regulatory network can be seen in Figure 3.
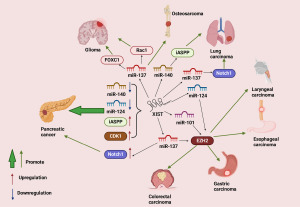
lncRNA MFSD4A antisense RNA 1 (MFSD4A-AS1)
MFSD4A-AS1 is a relatively novel lncRNA, and its precise functional mechanisms are yet to be fully elucidated. There is limited literature regarding its association with malignant tumors, with one study specifically highlighting its involvement in PTC. Liu et al.’s study proposed that MFSD4A-AS1 acts as a ceRNA by sequestering miR-30c-2-3p, miR-145-3p, and miR-139-5p (102). On one hand, it disrupts the miRNA-mediated inhibition of VEGFA and VEGFC, which are identified as the predominant tumor metastasis-driving factors in various human cancers types (103). On the other hand, MFSD4A-AS1 upregulates TGFBR2 and USP15 by sponging miR-30c-2-3p and activates the TGF-β signaling pathway. It has been demonstrated that the activation of the TGF-β signaling pathway promotes tumor lymphatic metastasis through various mechanisms (104-106). The synergistic effect of these two processes promotes lymphangiogenesis and lymphatic metastasis in PTC (102). However, the results of this study require further comprehensive clinical and experimental validation.
In conclusion, lncRNA MFSD4A-AS1, as an emerging lncRNA, presents significant potential for exploration in its association with malignancies, particularly in the context of PTC. Existing research has indicated its relevance to lymphatic metastasis in PTC. However, its expression levels show no significant differences between normal thyroid tissues and PTC tissues without lymph node metastasis, while it exhibits a notable increase in PTC tissues with lymph node metastasis. This raises the question of whether similar expression patterns exist in other tumor types, where elevated expression may also be specific to tumors with lymph node metastasis. This intriguing possibility opens up new avenues for future research on this gene.
lncRNA suppressing tumorigenicity 7 antisense RNA 1 (ST7-AS1)
ST7-AS1 is a newly discovered lncRNA that has been associated with immune infiltrates in breast cancer (107), the EMT process, anti-apoptotic mechanisms in gastric cancer (108,109), and the promotion of laryngeal squamous cell carcinoma by stabilizing CARM1 (110) which were negatively correlated with gene expression signatures of key immune pathways, including the MHC class I antigen presentation, type 1 interferon and IFNγ pathways (111), indicating its immunosuppressive effects and favorable contribution to tumor development. Additionally, it has been shown to facilitate the malignant transformation of lung adenocarcinoma by regulating the miR-181b-5p/KPNA4 axis (112). Moreover, studies have indicated that miR-3619-5p inhibits cell proliferation in cutaneous squamous cell carcinoma by targeting KPNA4 (113), and increased miR-181 reverses EMT in glioblastoma by reducing KPNA4 (114). This indirectly underscores the potential positive role of KPNA4 in cancer progression. Regarding the relationship between ST7-AS1 and PTC, there is a singular study positing that increased levels of ST7-AS1 may enhance the expression of TRIM3 by harboring miR-181b-5p, thereby manifesting a tumor-suppressive function (115). TRIM3 also functions as a tumor suppressor gene across multiple cancer types (116-118).
Based on the above discussion, it becomes evident that the impact of lncRNA ST7-AS1 varies across different types of tumors. It exhibits a positive effect on gastric cancer and laryngeal squamous cell carcinoma, while its effect is negative in the case of PTC. This highlights the intricate regulatory network of ST7-AS1 in the development of tumors. Given its status as a novel lncRNA, there remains significant room for further exploration into its relationship with cancer.
lncRNA forkhead box P4 antisense RNA 1 (FOXP4-AS1)
The lncRNA FOXP4-AS1 has recently been identified as an emerging cancer-related biomarker, exhibiting variable roles across different cancer types. The following is an introduction to specific regulatory mechanisms for each type of malignant tumor. In HCC, FOXP4-AS1 has been implicated in promoting tumor progression (119,120) and the mechanism involves FOXP4-AS1 inhibiting the expression of ZC3H12D by mediating the deposition of H3K27me3 through the recruitment of EZH2 (121). In esophageal squamous cell carcinoma (ESCC), FOXP4-AS1 also exerts a promotive role, as its mechanism involves upregulating the expression of FOXP4, thereby promoting the transcription of β-catenin, which is a crucial factor in the WNT signaling pathway and regulates tumorigenesis development (122), and ultimately driving the malignant progression of ESCC (123,124). In prostate cancer, FOXP4-AS1 functions by sequestering miR-3184-5p to upregulate FOXP4, which is a member of the P subfamily of the forkhead box (FOX) family, a novel transcription factor (125) and can regulate tumor growth, progression and metastasis (126), thereby promoting the growth of prostate cancer cells (127), In non-small cell lung cancer (NSCLC), FOXP4-AS1 similarly promotes tumor cell growth by interacting with miR-3184-5p. However, in this context, miR-3184-5p ultimately targets the protein EIF5A (128), which exists complex relationships between tumor growth and cell signal transduction (129). In nasopharyngeal carcinoma, the knockdown of FOXP4-AS1 resulted in a reduction of metastasis and the inhibition of EMT through the miR-136-5p/MAPK1 pathway (130-132). In cervical cancer, FOXP4-AS1 also acts as competing endogenous RNA for miR-136-5p but ultimately regulating chromobox 4 (CBX4) expression (133). In Ewing sarcoma (ES), Xiong et al. proposed that FOXP4-AS1 may potentially modulate the expression of TMPO by sequestering miR-298, thereby regulating the malignant phenotype of ES. However, further validation is required to substantiate this hypothesis. They also uncovered that FOXP4-AS1 might modulate the tumor immune microenvironment through extracellular vesicle-mediated mechanisms. Notably, three immune cell types, namely Tregs, activated NK cells, and M1 macrophages, exhibited significant associations with FOXP4-AS1 expression (134). These findings could potentially provide valuable insights for future immunotherapeutic strategies. In both colorectal cancer and Mantle cell lymphoma (MCL), FOXP4-AS1 operates as a competitive endogenous RNA by sequestering miR-423-5p, ultimately leading to an upregulation of nucleus accumbens-associated 1 (NACC1) expression, which has been reported to promote the progression of various cancers and studies have indicated its ability to transcriptionally activate HOXA9, thereby regulating apoptosis in colorectal cancer cells (135), then promoting tumor progression (136,137). This highlights the significance of this axis in oncogenesis. Furthermore, in osteosarcoma, upregulated FOXP4-AS1 promotes proliferation, migration, and cell cycle progression by downregulating LATS1, which has also been reported to be a tumor suppressor (138), through its interaction with LSD1 and EZH2 (139). Regarding its relationship with PTC, it exhibits a unique characteristic, as it exerts inhibitory effects on PTC through the modulation of the AKT signaling pathway. In contrast to previous findings, Luo et al. have suggested that FOXP4-AS1 also negatively regulates the expression of its host gene, FOXP4 (140).
In summary, FOXP4-AS1 appears to promote tumor progression in the majority of cancers, except for PTC, where it plays a suppressive role. The underlying reasons for its inhibitory role in PTC warrant further investigation. Additionally, the involvement of the FOXP4-AS1/miR-423-5p/NACC1 axis in two distinct classes of tumors underscores the significance of this regulatory pathway.
lncRNA solute carrier family 8 member A1 antisense RNA 1 (SLC8A1-AS1)
The lncRNA SLC8A1-AS1 is a recently discovered lncRNA in the past few years. Prior studies have suggested that lncRNA SLC8A1-AS1 may serve as an early diagnostic marker for oral squamous cell carcinoma (141). Study has also reported that the knockout of SLC8A1-AS1 inhibits the growth and migration of glioma cancer cells by suppressing the Wnt/β-catenin signaling pathway (142). Regarding its relationship with PTC, recent research has proposed a mechanism wherein SLC8A1-AS1 interacts with FUS within PTC cells, thereby preserving the stability of Numbl mRNA and subsequently modulating the notch signaling pathway. In a word, SLC8A1-AS1 suppresses PTC progression via the FUS/Numbl axis (143).
From the above content, it can be observed that there is currently a lack of comprehensive research on the relationship between SLC8A1-AS1 and cancer. Existing reports suggest that it can either promote the progression of certain tumors or inhibit the progression of others, indicating the existence of a complex regulatory network.
lncRNA long non-coding RNA-MPEG1-1 (lnc-MPEG1-1)
Research on the relationship between lnc-MPEG1-1 and cancer is very limited, with only one study suggesting its regulatory role in the progression of PTC. Huang et al. proposed that lnc-MPEG1-1 is overexpressed in the cytoplasm of PTC cells and functionally promotes cellular proliferation and migration in PTC cells by competitively occupying the shared binding sites of miR-766-5p (144), which is considered a tumor suppressor gene in many cancers (145,146). lnc-MPEG1-1 knockdown suppresses EMT by miR-766-5p in PTC cells. Additionally, it can serve as a predictor of lymph node metastasis in PTC (144).
lncRNA ring finger protein 185 antisense RNA 1 (RNF185-AS1)
LncRNA RNF185-AS1, situated on chromosome 22, represents a recently discovered lncRNA. Huang and colleagues have proposed that the lncRNA RNF185-AS1 can act as a “sponge” for miR-221-5p, binding to miR-221-5p and influencing its function. This interaction subsequently leads to the regulation of integrin β5, a substance known to promote tumorigenesis (147,148). Ultimately, these molecular mechanisms promotes the growth and metastasis of HCC (149). Regarding its relationship with PTC, Liu et al. suggested that the downregulation of RNF185-AS1 may potentially act as a “sponge” for miR-429, hindering the expression of LRP4 (150), a protein known to promote the development of various cancers (151,152). This inhibition of LRP4 expression could ultimately suppress the progression of PTC (150).
In summary, RNF185-AS1 has been found to target miRNAs, specifically miR-221-5p and miR-429, affecting HCC and PTC. Its mechanism of action involves competitive binding with these target miRNAs, ultimately regulating the expression of downstream cancer-related proteins, such as integrin β5 and LRP4, and thereby promoting cancer progression. Further research is needed to explore its associations with other types of cancer.
lncRNA regulator of reprogramming (ROR)
LncRNA ROR, known as a mediator of reprogramming, is recognized as an oncogene involved in cancer development (153). There is extensive research on the relationship between lncRNA ROR and cancers, with the majority suggesting its promotive role in cancer progression. we have found a close relationship between ROR and miRNA-145 in various cancer types. Specifically, in liver cancer, ROR acts as a competing endogenous RNA for miR-145, thereby regulating RAD18 expression and ultimately promoting DNA repair (154). In ovarian cancer, ROR promotes the EMT process through the miR-145/FLNB regulatory axis (155). In esophageal cancer, ROR regulates the miR-145/LMNB2 axis, thereby promoting the proliferation and migration of esophageal cancer cells (156). Lastly, in prostate cancer, inhibition of ROR increases the available concentration of miR-145, which in turn prevents cell proliferation by decreasing oct4 expression (157). Additionally, by reviewing a substantial body of literature, we have found that ROR promotes the progression of various tumors by facilitating the EMT process. Here are some specific examples: In nasopharyngeal carcinoma, breast cancer, and bladder cancer, high expression of ROR is associated with enhanced EMT process (156,158-160). In ovarian cancer, ROR promotes EMT through the miR-145/FLNB regulatory axis (155). Furthermore, in gallbladder cancer, ROR promotes EMT mediated by TGF-β1 (161). We have also discovered a strong association between ROR and p53: ROR is capable of inhibiting the p53 signaling pathway, leading to the development of chemotherapy resistance in nasopharyngeal carcinoma cells (162). Through its regulation of p53, ROR promotes the proliferation and viability of colorectal cancer cells (163). Depletion of ROR, on the other hand, enhances p53 expression, thereby facilitating apoptosis in renal cancer cells (164). Key studies have indicated that lncRNA ROR enhances breast cancer development by recruiting histone methyltransferase MLL1 to up-regulate TIMP3 expression (165), facilitates endometrial cancer cell proliferation by modulating the Notch1 signaling pathway (166), contributes to human colorectal cancer tumorigenesis by targeting miR-6833-3p through SMC4 (167), inhibits the tumor suppressor gene NF2 through interaction with miR-223-3p, affecting colon cancer (168). Additionally, lncRNA ROR has been found to promote breast cancer by regulating the TGF-β pathway (169). Notably, one significant study suggests that lncRNA ROR acts as a decoy for gene-specific histone methylation, thereby promoting tumorigenesis (170). In gastric cancer, ROR knockdown downregulated HMGA2 to restrain cell proliferation, migration, invasion by targeting miR-519d-3p (171). ROR expression promotes MRP1 expression and multi-drug resistant of gastric cancer (172) and led to upregulation of several key stemness transcriptional factors, such as OCT4, SOX2, and NANOG, increasing the proliferation and invasion of gastric cancer stem cells (173). ROR contributed to cisplatin resistance in osteosarcoma via miR-153-3p/ABCB1 axis (174). These findings collectively underscore the diverse roles of lncRNA ROR in cancer biology. For the specific detailed regulatory pathways of ROR in various types of tumors, please refer to Table 2. In relation to its association with PTC, Fan et al. revealed that lncRNA ROR inhibits the recruitment of G9a to the TESC promoter, thereby upregulating TESC expression. TESC is likely to induce the upregulation of ALDH1, which in turn increases the expression of TUBB3, leading to reduced PTEN expression. Consequently, this cascade of events promotes the proliferation, migration, and invasion of PTC cells while suppressing apoptosis (175). The specific regulatory network can be seen in Figure 4.
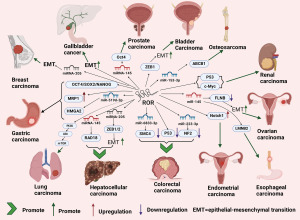
In summary, lncRNA ROR exhibits a complex regulatory network in the development and progression of various types of tumors. It promotes cell growth and invasion in different tumor types and contributes to resistance to chemotherapy and radiotherapy. The mechanisms by which ROR exerts its effects are commonly through interactions with miR-145, further regulating the expression of downstream target genes. It can also promote the EMT process in cancer cells. The aforementioned key mechanisms are worthy of further investigation.
lncRNA alpha/beta hydrolase domain containing 11 antisense RNA 1 (ABHD11-AS1)
There is a substantial amount of research regarding the functional role of lncRNA ABHD11-AS1, located on human chromosome 7 q11.23. LncRNA ABHD11-AS1 plays a regulatory role in various cancer types by modulating different molecular pathways. In cervical cancer, it prevents FUS-mediated degradation of ABHD11 mRNA, leading to the activation of the EGFR signaling pathway (176). It also competitively binds to miR-330-5p and upregulates MARK2 expression, thereby promoting cervical cancer development (177). In addition, it accelerates the proliferation, invasion, and migration of cervical carcinoma cells by regulating miR-1254 (178). In epithelial ovarian cancer, three studies have demonstrated that ABHD11-AS1 promotes the progression of ovarian cancer through different mechanisms, namely, the EZH2/ABHD11-AS1/miR-133a-3p axis (179), the ABHD11-AS1/EZH2/TIMP2 axis (180), and by increasing the expression of RhoC (181). ABHD11-AS1 functions as an oncogene to promote cell proliferation and invasion in endometrial carcinoma by positively targeting cyclin D1 (182). Knockdown of ABHD11-AS1 suppressed the progression of triple-negative breast cancer (TNBC) via upregulation of miR-199a-5p (183). In gastric cancer, boosts gastric cancer development by regulating miR-361-3p/PDPK1 signalling pathway (184). Furthermore, a study has suggested that the level of ABHD11-AS1 in gastric fluid is associated with tumor size, tumor stage, Lauren classification, and carcinoembryonic antigen levels in gastric cancer patients and ABHD11-AS1 in gastric fluid may serve as a potential biomarker for early gastric cancer screening (185). In pancreatic cancer, ABHD11-AS1 sponges miR-1231 (186), or activating the PI3K-AKT pathway (187), fostering cancer growth, and promoting lymphangiogenesis. In colorectal cancer, it advances cancer and invasion via ITGA5/FAK/PI3K/Akt pathway (188), miR-133a/SOX4 axis (189) or modulating the miR-1254-WNT11 pathway (190). In endometrial carcinoma, ABHD11-AS1 promotes tumor growth by targeting cyclin D1 (182). In epithelial ovarian cancer, it contributes to tumorigenesis and progression by regulating RhoC (181). Regarding its relationship with PTC, Lu et al. posit that ABHD11-AS1 promotes the progression of PTC by modulating the EPS15L1/EGFR pathway, leading to the activation of EGFR, EPS15L1, STAT3, and p-STAT3 (191). Zhuang et al. proposed that ABHD11-AS1 enhances cell proliferation, migration, and invasion in vitro by sequestering miR-199a-5p, leading to the activation of SLC1A5 and suppression of apoptosis, ultimately promoting tumorigenesis in vivo (192). Wen et al. proposed that ABHD11-AS1 positively regulates the PI3K/AKT signaling pathway, acting as a competitive endogenous RNA (ceRNA) by sequestering miR-1301-3p, thereby upregulating STAT3 expression, ultimately promoting the progression of PTC (193). The specific regulatory network can be seen in Figure 5.
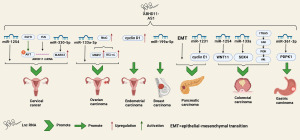
Based on the above information, it can be observed that lncRNA ABHD11-AS1 has been extensively studied in female reproductive system cancers such as cervical cancer and ovarian cancer, as well as digestive system cancers including pancreatic cancer, colorectal cancer, and gastric cancer. In all these cancers, ABHD11-AS1 has been found to play a role in promoting cancer progression. Additionally, in PTC, ABHD11-AS1 is also involved in tumor progression through different mechanisms. ABHD11-AS1 has the potential to serve as a novel target for cancer therapy. We have summarized the significant signaling pathways related to tumors other than PTC in Table 2. The regulation related to PTC has been compiled in Table 3.
Discussion
Cancer-related lncRNAs have gradually become a hot topic in the fields of RNA biology and oncology. In recent years, numerous studies have explored lncRNAs associated with PTC (194-196). Specifically, the biological and clinical relevance between lncRNAs and the occurrence and development of PTC is mainly manifested in the following aspects: (I) lncRNAs can competitively bind to targeted miRNAs that can mediate the degradation of mRNAs, thereby promoting the expression of specific PTC-related mRNAs and proteins. (II) Promote the modification of specific proteins and then affect their protein functions, such as enhancing the activity of metabolism-related enzymes. (III) Stimulate the degradation of tumor suppressor proteins such as P53. (IV) Activate specific oncogenic signaling pathways, such as the EPS15L1/EGFR signaling pathway, thereby promoting lymph node metastasis of PTC. All these pathways lead to the occurrence and development of PTC and poor clinical prognosis. However, current studies also show that a few lncRNAs can enhance the apoptosis of PTC cells and inhibit their proliferation (115,140,143). The mechanism is generally as follows: (I) competitively bind to targeted miRNAs to promote the expression of specific PTC inhibitory mRNAs and proteins. (II) Inhibit the progression of PTC by regulating tumor-related signaling pathways such as the AKT signaling pathway. Therefore, the role of lncRNAs in the occurrence and development of PTC is very complex and still has a very broad exploration prospect. It is worth noting that the most important mechanism by which lncRNAs affect PTC is through competitively binding to targeted miRNAs, thereby influencing the tumor-related mRNAs and proteins downstream of miRNAs and ultimately affecting the occurrence, development and prognosis of PTC.
According to the important role of lncRNAs in the pathogenesis of PTC as described above, we can propose corresponding possible treatment plans based on its carcinogenic pathogenesis: (I) since lncRNAs competitively bind to miRNAs and prevent miRNAs from degrading targeted mRNAs, we can consider developing molecules that mimic miRNA activity to compensate for the role of miRNAs and play an antagonistic role against the carcinogenesis of lncRNAs. Existing studies have shown that this method can be used for the treatment of diseases (197,198). (II) Since carcinogenic lncRNAs related to PTC are overexpressed in PTC, specific drugs for treating PTC can be developed based on the principle that antisense oligonucleotides (ASOs) and siRNAs specifically bind to the target lncRNAs and then mediate the degradation of the target lncRNAs (199-202).
Radiotherapy has currently become an important means of treating patients with PTC (203). However, if patients have radiotherapy resistance, the therapeutic effect will be greatly reduced. A study has pointed out that radioiodine resistance is an important cause of death during PTC treatment, and the 10-year survival rate of affected patients is as low as 10% (204). Therefore, studying the influence of lncRNA on the sensitivity or resistance of radiotherapy in PTC patients is a very valuable topic. Existing studies have shown that lncRNA has a complex influence on the radioresistance and radiosensitivity of PTC. Among them, the study by Shi et al. found that LncRNA GLTC can enhance radioiodine resistance in papillary thyroid cancer (60). However, studies by some scholars have found that LncRNA-SLC6A9-5:2 and lncRNA CASC2 can increase the sensitivity of drug-resistant thyroid cancer cells to iodine-131 treatment (205,206). This clearly indicates that at present, there remain gaps in this field, and there is still extensive research space that merits further exploration.
Screening lncRNAs related to prognosis is of great significance for our future translational applications in clinical practice. Currently, the commonly used lncRNAs related to the prognosis of PTC patients are determined through statistical analysis and screening through The Cancer Genome Atlas (TCGA) database or other public databases. However, the lncRNAs identified by the above methods have not been verified by in vivo and in vitro experiments, so they do not have high credibility. Therefore, by searching relevant articles, we first determine high-quality PTC-related lncRNAs that have been experimentally verified, and then screen out lncRNAs related to the prognosis of PTC from them, which can effectively ensure their reliability. After careful screening, we found that in PTC patients, lncRNA SOCS2-AS1 and ABHD11-AS1 are significantly associated with poor prognosis (59,193). High expression of RNF185-AS1 is associated with larger tumor size, lymph node metastasis, and more advanced tumor-node-metastasis stage (150). High expression of lncRNA lnc-MPEG1-1 and MFSD4A-AS1 is significantly associated with lymph node metastasis in PTC patients, also indicating their association with worse prognosis (102,144). However, lncRNA FOXP4-AS1 is associated with a better prognosis in PTC patients (140). Based on this, we can construct a multi-lncRNA signature (SOCS2-AS1, ABHD11-AS1, RNF185-AS1, lnc-MPEG1-1, MFSD4A-AS1, FOXP4-AS1) to effectively predict the prognosis of PTC patients.
Moreover, it has been discovered that these lncRNAs also play regulatory roles in other malignant tumors. This indicates that they have complex regulatory networks involved in the growth processes of malignant tumors. Common mechanisms include the regulation of protein expression related to apoptosis, autophagy, and the cell cycle, as well as involvement in the EMT process of malignant tumor cells and the regulation of specific signaling pathways. Among these, the most common mechanism is the competitive binding of lncRNAs with specific miRNAs, ultimately affecting the expression of downstream proteins. The specific mechanisms are illustrated in Figure 6. However, for some recently discovered PTC-related lncRNAs, there is currently a lack of research reports on their association with other malignant tumors. Therefore, further research in this area is warranted. This review provides a valuable reference for future research.
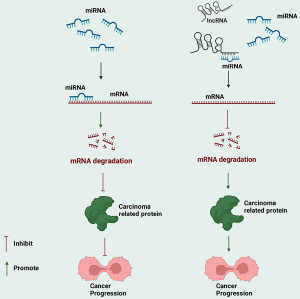
Finally, although developing treatment regimens for malignant tumors based on lncRNAs is highly promising, it cannot be ignored that there are indeed some issues and challenges in the clinical application of lncRNAs that need to be overcome. (I) Specificity issues: undesired on-target effects due to uptake in cells other than the target cells, or off-target effects caused by sequence similarities or overdosing to levels much higher than the expected endogenous level. (II) Delivery issues: the “naked”, unchemically modified RNA structure is unstable; there is inefficient intracellular delivery of RNA, and endosomal escape mechanisms need to be utilized for improvement; there is a lack of delivery vehicles suitable for targeting specific target organs and cell types. (III) Tolerability issues, such as the recognition of RNA structures by pathogen-associated molecular pattern receptors (such as Toll-like receptors), leading to adverse immune reactions (207,208). The above challenges also provide valuable directions for future research in this field.
Conclusions
The lncRNAs associated with PTC exert regulatory functions in other malignancies as well and possess similar regulatory mechanisms. The main mechanism is that PTC-related lncRNAs can competitively interact with miRNAs. This interaction affects miRNA-targeted mRNA and the expression of cancer-related proteins, ultimately promoting the progression of PTC and other malignant tumors. This provides a molecular basis for the future development of relevant targeted therapies.
Acknowledgments
None.
Footnote
Reporting Checklist: The authors have completed the Narrative Review reporting checklist. Available at https://tcr.amegroups.com/article/view/10.21037/tcr-24-1038/rc
Peer Review File: Available at https://tcr.amegroups.com/article/view/10.21037/tcr-24-1038/prf
Funding: None.
Conflicts of Interest: Both authors have completed the ICMJE uniform disclosure form (available at https://tcr.amegroups.com/article/view/10.21037/tcr-24-1038/coif). The authors have no conflicts of interest to declare.
Ethical Statement: The authors are accountable for all aspects of the work in ensuring that questions related to the accuracy or integrity of any part of the work are appropriately investigated and resolved.
Open Access Statement: This is an Open Access article distributed in accordance with the Creative Commons Attribution-NonCommercial-NoDerivs 4.0 International License (CC BY-NC-ND 4.0), which permits the non-commercial replication and distribution of the article with the strict proviso that no changes or edits are made and the original work is properly cited (including links to both the formal publication through the relevant DOI and the license). See: https://creativecommons.org/licenses/by-nc-nd/4.0/.
References
- Megwalu UC, Moon PK. Thyroid Cancer Incidence and Mortality Trends in the United States: 2000-2018. Thyroid 2022;32:560-70. [Crossref] [PubMed]
- Lim H, Devesa SS, Sosa JA, et al. Trends in Thyroid Cancer Incidence and Mortality in the United States, 1974-2013. JAMA 2017;317:1338-48. [Crossref] [PubMed]
- Luo H, Xia X, Kim GD, et al. Characterizing dedifferentiation of thyroid cancer by integrated analysis. Sci Adv 2021;7:eabf3657. [Crossref] [PubMed]
- Xing M, Haugen BR, Schlumberger M. Progress in molecular-based management of differentiated thyroid cancer. Lancet 2013;381:1058-69. [Crossref] [PubMed]
- Anastasiadou E, Jacob LS, Slack FJ. Non-coding RNA networks in cancer. Nat Rev Cancer 2018;18:5-18. [Crossref] [PubMed]
- Xu M, Chen X, Lin K, et al. lncRNA SNHG6 regulates EZH2 expression by sponging miR-26a/b and miR-214 in colorectal cancer. J Hematol Oncol 2019;12:3. [Crossref] [PubMed]
- Shi L, Yan H, An S, et al. SIRT5-mediated deacetylation of LDHB promotes autophagy and tumorigenesis in colorectal cancer. Mol Oncol 2019;13:358-75. [Crossref] [PubMed]
- Xu M, Chen X, Lin K, et al. The long noncoding RNA SNHG1 regulates colorectal cancer cell growth through interactions with EZH2 and miR-154-5p. Mol Cancer 2018;17:141. [Crossref] [PubMed]
- Xu M, Xu X, Pan B, et al. LncRNA SATB2-AS1 inhibits tumor metastasis and affects the tumor immune cell microenvironment in colorectal cancer by regulating SATB2. Mol Cancer 2019;18:135. [Crossref] [PubMed]
- Peng JY, Cai DK, Zeng RL, et al. Upregulation of Superenhancer-Driven LncRNA FASRL by USF1 Promotes De Novo Fatty Acid Biosynthesis to Exacerbate Hepatocellular Carcinoma. Adv Sci (Weinh) 2022; Epub ahead of print. [Crossref]
- Liu F, Tian T, Zhang Z, et al. Long non-coding RNA SNHG6 couples cholesterol sensing with mTORC1 activation in hepatocellular carcinoma. Nat Metab 2022;4:1022-40. [Crossref] [PubMed]
- Zhang B, Bao W, Zhang S, et al. LncRNA HEPFAL accelerates ferroptosis in hepatocellular carcinoma by regulating SLC7A11 ubiquitination. Cell Death Dis 2022;13:734. [Crossref] [PubMed]
- Zhang Q, Wei T, Yan L, et al. Hypoxia-Responsive lncRNA AC115619 Encodes a Micropeptide That Suppresses m6A Modifications and Hepatocellular Carcinoma Progression. Cancer Res 2023;83:2496-512. [Crossref] [PubMed]
- Wang H, Huo X, Yang XR, et al. STAT3-mediated upregulation of lncRNA HOXD-AS1 as a ceRNA facilitates liver cancer metastasis by regulating SOX4. Mol Cancer 2017;16:136. [Crossref] [PubMed]
- Hou YR, Diao LT, Hu YX, et al. The Conserved LncRNA DIO3OS Restricts Hepatocellular Carcinoma Stemness by Interfering with NONO-Mediated Nuclear Export of ZEB1 mRNA. Adv Sci (Weinh) 2023;10:e2301983. [Crossref] [PubMed]
- Su T, Zhang N, Wang T, et al. Super Enhancer-Regulated LncRNA LINC01089 Induces Alternative Splicing of DIAPH3 to Drive Hepatocellular Carcinoma Metastasis. Cancer Res 2023;83:4080-94. [Crossref] [PubMed]
- Yu X, Qian F, Zhang X, et al. Promotion effect of FOXCUT as a microRNA sponge for miR-24-3p on progression in triple-negative breast cancer through the p38 MAPK signaling pathway. Chin Med J (Engl) 2024;137:105-14. [Crossref] [PubMed]
- Collette J, Le Bourhis X, Adriaenssens E. Regulation of Human Breast Cancer by the Long Non-Coding RNA H19. Int J Mol Sci 2017;18:2319. [Crossref] [PubMed]
- Bin X, Hongjian Y, Xiping Z, et al. Research progresses in roles of LncRNA and its relationships with breast cancer. Cancer Cell Int 2018;18:179. [Crossref] [PubMed]
- Bian S, Wang Y, Zhou Y, et al. Integrative single-cell multiomics analyses dissect molecular signatures of intratumoral heterogeneities and differentiation states of human gastric cancer. Natl Sci Rev 2023;10:nwad094. [Crossref] [PubMed]
- Fu D, Huang Y, Wang S, et al. HAGLROS knockdown restrained cell proliferation, migration and invasion and facilitated apoptosis in laryngeal cancer via miR-138-5p/CLN5 axis. J Clin Lab Anal 2022;36:e24712. [Crossref] [PubMed]
- Xiao S, Zuo Y, Li Y, et al. Long Noncoding RNA HAGLROS Promotes the Malignant Progression of Bladder Cancer by Regulating the miR-330-5p/SPRR1B Axis. Front Oncol 2022;12:876090. [Crossref] [PubMed]
- Tang G, Zhao H, Xie Z, et al. Long non-coding RNA HAGLROS facilitates tumorigenesis and progression in hepatocellular carcinoma by sponging miR-26b-5p to up-regulate karyopherin α2 (KPNA2) and inactivate p53 signaling. Bioengineered 2022;13:7829-46. [Crossref] [PubMed]
- Zheng L, He J, Li M, et al. Clinical significance and mechanism of long noncoding RNA HAGLROS in triple negative breast cancer. Pathol Res Pract 2022;231:153810. [Crossref] [PubMed]
- Gai L, Huang Y, Zhao L, et al. Long non-coding RNA HAGLROS regulates the proliferation, migration, and apoptosis of esophageal cancer cells via the HAGLROS-miR-206-NOTCH3 axis. J Gastrointest Oncol 2021;12:2093-108. [Crossref] [PubMed]
- Zhu L, Mei M. Interference of long non-coding RNA HAGLROS inhibits the proliferation and promotes the apoptosis of ovarian cancer cells by targeting miR-26b-5p. Exp Ther Med 2021;22:879. [Crossref] [PubMed]
- Li L, Zhu H, Li X, et al. Long non-coding RNA HAGLROS facilitates the malignant phenotypes of NSCLC cells via repressing miR-100 and up-regulating SMARCA5. Biomed J 2021;44:S305-15. [Crossref] [PubMed]
- Wang WL, Yu DJ, Zhong M. LncRNA HAGLROS accelerates the progression of lung carcinoma via sponging microRNA-152. Eur Rev Med Pharmacol Sci 2019;23:6531-8. [Crossref] [PubMed]
- Wei H, Hu J, Pu J, et al. Long noncoding RNA HAGLROS promotes cell proliferation, inhibits apoptosis and enhances autophagy via regulating miR-5095/ATG12 axis in hepatocellular carcinoma cells. Int Immunopharmacol 2019;73:72-80. [Crossref] [PubMed]
- Chen JF, Wu P, Xia R, et al. STAT3-induced lncRNA HAGLROS overexpression contributes to the malignant progression of gastric cancer cells via mTOR signal-mediated inhibition of autophagy. Mol Cancer 2018;17:6. [Crossref] [PubMed]
- Shu L, Guo K, Lin ZH, et al. Long non-coding RNA HAGLROS promotes the development of diffuse large B-cell lymphoma via suppressing miR-100. J Clin Lab Anal 2022;36:e24168. [Crossref] [PubMed]
- Mutlu Icduygu F, Akgun E, Ozgoz A, et al. Upregulation and the clinical significance of KCNQ1OT1 and HAGLROS lncRNAs in papillary thyroid cancer: An observational study. Medicine (Baltimore) 2023;102:e34379. [Crossref] [PubMed]
- Zeng Z, Tang S, Chen L, et al. LncRNA HAGLROS contribute to papillary thyroid cancer progression by modulating miR-206/HMGA2 expression. Aging (Albany NY) 2023;15:14930-44. [Crossref] [PubMed]
- Zhang T, Zhang G, Yang W, et al. Lnc-PFAR facilitates autophagy and exacerbates pancreatic fibrosis by reducing pre-miR-141 maturation in chronic pancreatitis. Cell Death Dis 2021;12:996. [Crossref] [PubMed]
- Fang T, Yu K. LncRNA PFAR facilitates the proliferation and migration of papillary thyroid carcinoma by competitively binding to miR-15a. Naunyn Schmiedebergs Arch Pharmacol 2024;397:3037-48. [Crossref] [PubMed]
- Carr LL, Mankoff DA, Goulart BH, et al. Phase II study of daily sunitinib in FDG-PET-positive, iodine-refractory differentiated thyroid cancer and metastatic medullary carcinoma of the thyroid with functional imaging correlation. Clin Cancer Res 2010;16:5260-8. [Crossref] [PubMed]
- Jin J, Zhang J, Xue Y, et al. miRNA-15a regulates the proliferation and apoptosis of papillary thyroid carcinoma via regulating AKT pathway. Onco Targets Ther 2019;12:6217-26. [Crossref] [PubMed]
- Couto JP, Almeida A, Daly L, et al. AZD1480 blocks growth and tumorigenesis of RET- activated thyroid cancer cell lines. PLoS One 2012;7:e46869. [Crossref] [PubMed]
- Heras-Sandoval D, Pérez-Rojas JM, Hernández-Damián J, et al. The role of PI3K/AKT/mTOR pathway in the modulation of autophagy and the clearance of protein aggregates in neurodegeneration. Cell Signal 2014;26:2694-701. [Crossref] [PubMed]
- Bhardwaj JK, Paliwal A, Saraf P, et al. Role of autophagy in follicular development and maintenance of primordial follicular pool in the ovary. J Cell Physiol 2022;237:1157-70. [Crossref] [PubMed]
- Bianconi D, Unseld M, Prager GW. Integrins in the Spotlight of Cancer. Int J Mol Sci 2016;17:2037. [Crossref] [PubMed]
- Xie L, Li Z, Chen Z, et al. ITGB1 alleviates osteoarthritis by inhibiting cartilage inflammation and apoptosis via activating cAMP pathway. J Orthop Surg Res 2023;18:849. [Crossref] [PubMed]
- Liu D, Liu S, Fang Y, et al. Comprehensive Analysis of the Expression and Prognosis for ITGBs: Identification of ITGB5 as a Biomarker of Poor Prognosis and Correlated with Immune Infiltrates in Gastric Cancer. Front Cell Dev Biol 2021;9:816230. [Crossref] [PubMed]
- Misawa A, Takayama K, Urano T, et al. Androgen-induced Long Noncoding RNA (lncRNA) SOCS2-AS1 Promotes Cell Growth and Inhibits Apoptosis in Prostate Cancer Cells. J Biol Chem 2016;291:17861-80. [Crossref] [PubMed]
- Ratanabunyong S, Siriwaseree J, Wanaragthai P, et al. Exploring the apoptotic effects of sericin on HCT116 cells through comprehensive nanostring transcriptomics and proteomics analysis. Sci Rep 2024;14:2366. [Crossref] [PubMed]
- Lefebvre C, Rajbhandari P, Alvarez MJ, et al. A human B-cell interactome identifies MYB and FOXM1 as master regulators of proliferation in germinal centers. Mol Syst Biol 2010;6:377. [Crossref] [PubMed]
- Zhang N, Wei P, Gong A, et al. FoxM1 promotes β-catenin nuclear localization and controls Wnt target-gene expression and glioma tumorigenesis. Cancer Cell 2011;20:427-42. [Crossref] [PubMed]
- Zheng Z, Li X, You H, et al. LncRNA SOCS2-AS1 inhibits progression and metastasis of colorectal cancer through stabilizing SOCS2 and sponging miR-1264. Aging (Albany NY) 2020;12:10517-26. [Crossref] [PubMed]
- Zhao X, Zhang W, Ji W. miR-196b is a prognostic factor of human laryngeal squamous cell carcinoma and promotes tumor progression by targeting SOCS2. Biochem Biophys Res Commun 2018;501:584-92. [Crossref] [PubMed]
- Tong JL, Wang LL, Ling XF, et al. MiR-875 can regulate the proliferation and apoptosis of non-small cell lung cancer cells via targeting SOCS2. Eur Rev Med Pharmacol Sci 2019;23:5235-41. [Crossref] [PubMed]
- Yin J, Park G, Lee JE, et al. CPEB1 modulates differentiation of glioma stem cells via downregulation of HES1 and SIRT1 expression. Oncotarget 2014;5:6756-69. [Crossref] [PubMed]
- Galardi S, Petretich M, Pinna G, et al. CPEB1 restrains proliferation of Glioblastoma cells through the regulation of p27(Kip1) mRNA translation. Sci Rep 2016;6:25219. [Crossref] [PubMed]
- Zhong F, Wang Y. YY1-regulated lncRNA SOCS2-AS1 suppresses HCC cell stemness and progression via miR-454-3p/CPEB1. Biochem Biophys Res Commun 2023;679:98-109. [Crossref] [PubMed]
- Damodaran AP, Vaufrey L, Gavard O, et al. Aurora A Kinase Is a Priority Pharmaceutical Target for the Treatment of Cancers. Trends Pharmacol Sci 2017;38:687-700. [Crossref] [PubMed]
- Wu C, Lyu J, Yang EJ, et al. Targeting AURKA-CDC25C axis to induce synthetic lethality in ARID1A-deficient colorectal cancer cells. Nat Commun 2018;9:3212. [Crossref] [PubMed]
- Wang-Bishop L, Chen Z, Gomaa A, et al. Inhibition of AURKA Reduces Proliferation and Survival of Gastrointestinal Cancer Cells With Activated KRAS by Preventing Activation of RPS6KB1. Gastroenterology 2019;156:662-675.e7. [Crossref] [PubMed]
- Jian F, Che X, Zhang J, et al. The long-noncoding RNA SOCS2-AS1 suppresses endometrial cancer progression by regulating AURKA degradation. Cell Death Dis 2021;12:351. [Crossref] [PubMed]
- Wu D, Sun J, Wang H, et al. LncRNA SOCS2-AS1 promotes the progression of glioma via regulating ITGB1 expression. Neurosci Lett 2021;765:136248. [Crossref] [PubMed]
- Zhang X, Zhang X, Yang G, et al. LncRNA SOCS2-AS1 promotes the progression of papillary thyroid cancer by destabilizing p53 protein. Biochem Biophys Res Commun 2023;669:95-102. [Crossref] [PubMed]
- Shi L, Duan R, Sun Z, et al. LncRNA GLTC targets LDHA for succinylation and enzymatic activity to promote progression and radioiodine resistance in papillary thyroid cancer. Cell Death Differ 2023;30:1517-32. [Crossref] [PubMed]
- Icard P, Loi M, Wu Z, et al. Metabolic Strategies for Inhibiting Cancer Development. Adv Nutr 2021;12:1461-80. [Crossref] [PubMed]
- Ganapathy-Kanniappan S, Geschwind JF. Tumor glycolysis as a target for cancer therapy: progress and prospects. Mol Cancer 2013;12:152. [Crossref] [PubMed]
- Hou X, Shi X, Zhang W, et al. LDHA induces EMT gene transcription and regulates autophagy to promote the metastasis and tumorigenesis of papillary thyroid carcinoma. Cell Death Dis 2021;12:347. [Crossref] [PubMed]
- Ban EJ, Kim D, Kim JK, et al. Lactate Dehydrogenase A as a Potential New Biomarker for Thyroid Cancer. Endocrinol Metab (Seoul) 2021;36:96-105. [Crossref] [PubMed]
- Li X, Zhang C, Zhao T, et al. Lysine-222 succinylation reduces lysosomal degradation of lactate dehydrogenase a and is increased in gastric cancer. J Exp Clin Cancer Res 2020;39:172. [Crossref] [PubMed]
- Yang J, Qi M, Fei X, et al. Long non-coding RNA XIST: a novel oncogene in multiple cancers. Mol Med 2021;27:159. [Crossref] [PubMed]
- Wu D, Nie X, Ma C, et al. RSF1 functions as an oncogene in osteosarcoma and is regulated by XIST/miR-193a-3p axis. Biomed Pharmacother 2017;95:207-14. [Crossref] [PubMed]
- Chen DL, Ju HQ, Lu YX, et al. Long non-coding RNA XIST regulates gastric cancer progression by acting as a molecular sponge of miR-101 to modulate EZH2 expression. J Exp Clin Cancer Res 2016;35:142. [Crossref] [PubMed]
- Liu X, Cui L, Hua D. Long Noncoding RNA XIST Regulates miR-137-EZH2 Axis to Promote Tumor Metastasis in Colorectal Cancer. Oncol Res 2018;27:99-106. Retracted Publication. [Crossref] [PubMed]
- Xiao D, Cui X, Wang X. Long noncoding RNA XIST increases the aggressiveness of laryngeal squamous cell carcinoma by regulating miR-124-3p/EZH2. Exp Cell Res 2019;381:172-8. [Crossref] [PubMed]
- Zhang D, Qiu Y, Zhang W, et al. Homeobox B9 promotes the invasion and metastasis of hepatocellular carcinoma cells via the EZH2-MIR203A-SNAI2 axis. J Transl Med 2024;22:918. [Crossref] [PubMed]
- Huang G, Xu J, Li Y, et al. Corynoxine exerts the anti-tumor effect on esophageal squamous cell carcinoma principally via the EZH2-DUSP5-ERK1/2-mediated cell growth inhibition. Phytomedicine 2024;135:156103. [Crossref] [PubMed]
- Chen DL, Chen LZ, Lu YX, et al. Long noncoding RNA XIST expedites metastasis and modulates epithelial-mesenchymal transition in colorectal cancer. Cell Death Dis 2017;8:e3011. [Crossref] [PubMed]
- Shen J, Hong L, Yu D, et al. LncRNA XIST promotes pancreatic cancer migration, invasion and EMT by sponging miR-429 to modulate ZEB1 expression. Int J Biochem Cell Biol 2019;113:17-26. [Crossref] [PubMed]
- Krebs AM, Mitschke J, Lasierra Losada M, et al. The EMT-activator Zeb1 is a key factor for cell plasticity and promotes metastasis in pancreatic cancer. Nat Cell Biol 2017;19:518-29. [Crossref] [PubMed]
- Ma J, Zhan Y, Xu Z, et al. ZEB1 induced miR-99b/let-7e/miR-125a cluster promotes invasion and metastasis in esophageal squamous cell carcinoma. Cancer Lett 2017;398:37-45. [Crossref] [PubMed]
- Tang Y, He R, An J, et al. lncRNA XIST interacts with miR-140 to modulate lung cancer growth by targeting iASPP. Oncol Rep 2017;38:941-8. [Crossref] [PubMed]
- Hai B, Pan X, Du C, et al. LncRNA XIST Promotes Growth of Human Chordoma Cells by Regulating miR-124-3p/iASPP Pathway. Onco Targets Ther 2020;13:4755-65. [Crossref] [PubMed]
- Liang S, Gong X, Zhang G, et al. The lncRNA XIST interacts with miR-140/miR-124/iASPP axis to promote pancreatic carcinoma growth. Oncotarget 2017;8:113701-18. [Crossref] [PubMed]
- Cao L, Huang Q, He J, et al. Elevated expression of iASPP correlates with poor prognosis and chemoresistance/radioresistance in FIGO Ib1-IIa squamous cell cervical cancer. Cell Tissue Res 2013;352:361-9. [Crossref] [PubMed]
- Lu B, Guo H, Zhao J, et al. Increased expression of iASPP, regulated by hepatitis B virus X protein-mediated NF-κB activation, in hepatocellular carcinoma. Gastroenterology 2010;139:2183-2194.e5. [Crossref] [PubMed]
- Zheng H, Zhang M, Ke X, et al. LncRNA XIST/miR-137 axis strengthens chemo-resistance and glycolysis of colorectal cancer cells by hindering transformation from PKM2 to PKM1. Cancer Biomark 2021;30:395-406. [Crossref] [PubMed]
- Liu PJ, Pan YH, Wang DW, et al. Long non coding RNA XIST promotes cell proliferation of pancreatic cancer through miR 137 and Notch1 pathway. Eur Rev Med Pharmacol Sci 2020;24:12161-70. [Crossref] [PubMed]
- Wang X, Zhang G, Cheng Z, et al. Knockdown of LncRNA-XIST Suppresses Proliferation and TGF-β1-Induced EMT in NSCLC Through the Notch-1 Pathway by Regulation of miR-137. Genet Test Mol Biomarkers 2018;22:333-42. [Crossref] [PubMed]
- Yu H, Xue Y, Wang P, et al. Knockdown of long non-coding RNA XIST increases blood-tumor barrier permeability and inhibits glioma angiogenesis by targeting miR-137. Oncogenesis 2017;6:e303. [Crossref] [PubMed]
- Wang Z, Yuan J, Li L, et al. Long non-coding RNA XIST exerts oncogenic functions in human glioma by targeting miR-137. Am J Transl Res 2017;9:1845-55.
- Li H, Cui J, Xu B, et al. Long non-coding RNA XIST serves an oncogenic role in osteosarcoma by sponging miR-137. Exp Ther Med 2019;17:730-8. [Crossref] [PubMed]
- Cheng Y, Chang Q, Zheng B, et al. LncRNA XIST promotes the epithelial to mesenchymal transition of retinoblastoma via sponging miR-101. Eur J Pharmacol 2019;843:210-6. [Crossref] [PubMed]
- Wu X, Dinglin X, Wang X, et al. Long noncoding RNA XIST promotes malignancies of esophageal squamous cell carcinoma via regulation of miR-101/EZH2. Oncotarget 2017;8:76015-28. [Crossref] [PubMed]
- Parodi S. Xist noncoding RNA could act as a tumor suppressor gene in patients with classical Hodgkin's disease. J Cancer Res Ther 2020;16:7-12. [Crossref] [PubMed]
- Ma SQ, Wang YC, Li Y, et al. LncRNA XIST promotes proliferation and cisplatin resistance of oral squamous cell carcinoma by downregulating miR-27b-3p. J Biol Regul Homeost Agents 2020;34:1993-2001. [Crossref] [PubMed]
- Li Q, Sun Q, Zhu B. LncRNA XIST Inhibits the Progression of Oral Squamous Cell Carcinoma via Sponging miR-455-3p/BTG2 Axis. Onco Targets Ther 2020;13:11211-20. [Crossref] [PubMed]
- Du YL, Liang Y, Cao Y, et al. LncRNA XIST Promotes Migration and Invasion of Papillary Thyroid Cancer Cell by Modulating MiR-101-3p/CLDN1 Axis. Biochem Genet 2021;59:437-52. [Crossref] [PubMed]
- Qiu J, Zhang W, Zang C, et al. Identification of key genes and miRNAs markers of papillary thyroid cancer. Biol Res 2018;51:45. [Crossref] [PubMed]
- Wang C, Lu S, Jiang J, et al. Hsa-microRNA-101 suppresses migration and invasion by targeting Rac1 in thyroid cancer cells. Oncol Lett 2014;8:1815-21. [Crossref] [PubMed]
- Zhao H, Tang H, Huang Q, et al. MiR-101 targets USP22 to inhibit the tumorigenesis of papillary thyroid carcinoma. Am J Cancer Res 2016;6:2575-86.
- Kwon MJ. Emerging roles of claudins in human cancer. Int J Mol Sci 2013;14:18148-80. [Crossref] [PubMed]
- Cai T, He Y, Peng B. IncRNA XIST Stimulates Papillary Thyroid Cancer Development through the miR-330-3p/PDE5A Axis. Crit Rev Eukaryot Gene Expr 2023;33:13-26. [Crossref] [PubMed]
- Arozarena I, Sanchez-Laorden B, Packer L, et al. Oncogenic BRAF induces melanoma cell invasion by downregulating the cGMP-specific phosphodiesterase PDE5A. Cancer Cell 2011;19:45-57. [Crossref] [PubMed]
- Houslay MD. Hard times for oncogenic BRAF-expressing melanoma cells. Cancer Cell 2011;19:3-4. [Crossref] [PubMed]
- Xu Y, Wang J, Wang J. Long noncoding RNA XIST promotes proliferation and invasion by targeting miR-141 in papillary thyroid carcinoma. Onco Targets Ther 2018;11:5035-43. [Crossref] [PubMed]
- Liu X, Zhang C, Wang X, et al. Long non-coding RNA MFSD4A-AS1 promotes lymphangiogenesis and lymphatic metastasis of papillary thyroid cancer. Endocr Relat Cancer 2023;30:e220221. [Crossref] [PubMed]
- Liu Z, Wang J, Mao Y, et al. MicroRNA-101 suppresses migration and invasion via targeting vascular endothelial growth factor-C in hepatocellular carcinoma cells. Oncol Lett 2016;11:433-8. [Crossref] [PubMed]
- Liu D, Li L, Zhang XX, et al. SIX1 promotes tumor lymphangiogenesis by coordinating TGFβ signals that increase expression of VEGF-C. Cancer Res 2014;74:5597-607. [Crossref] [PubMed]
- Huang SC, Wei PC, Hwang-Verslues WW, et al. TGF-β1 secreted by Tregs in lymph nodes promotes breast cancer malignancy via up-regulation of IL-17RB. EMBO Mol Med 2017;9:1660-80. [Crossref] [PubMed]
- Shu YJ, Bao RF, Jiang L, et al. MicroRNA-29c-5p suppresses gallbladder carcinoma progression by directly targeting CPEB4 and inhibiting the MAPK pathway. Cell Death Differ 2017;24:445-57. [Crossref] [PubMed]
- Zhang Z, Zhang H, Li D, et al. LncRNA ST7-AS1 is a Potential Novel Biomarker and Correlated With Immune Infiltrates for Breast Cancer. Front Mol Biosci 2021;8:604261. [Crossref] [PubMed]
- Cai S, Weng Y, Liu P, et al. Knockdown of ST7-AS1 inhibits migration, invasion, cell cycle progression and induces apoptosis of gastric cancer. Oncol Lett 2020;19:777-82. [Crossref] [PubMed]
- Yang KD, Wang Y, Zhang F, et al. CAF-derived midkine promotes EMT and cisplatin resistance by upregulating lncRNA ST7-AS1 in gastric cancer. Mol Cell Biochem 2022;477:2493-505. [Crossref] [PubMed]
- Qin H, Xu J, Gong L, et al. The long noncoding RNA ST7-AS1 promotes laryngeal squamous cell carcinoma by stabilizing CARM1. Biochem Biophys Res Commun 2019;512:34-40. [Crossref] [PubMed]
- Kumar S, Zeng Z, Bagati A, et al. CARM1 Inhibition Enables Immunotherapy of Resistant Tumors by Dual Action on Tumor Cells and T Cells. Cancer Discov 2021;11:2050-71. [Crossref] [PubMed]
- Hu RH, Zhang ZT, Wei HX, et al. LncRNA ST7-AS1, by regulating miR-181b-5p/KPNA4 axis, promotes the malignancy of lung adenocarcinoma. Cancer Cell Int 2020;20:568. [Crossref] [PubMed]
- Zhang M, Luo H, Hui L. MiR-3619-5p hampers proliferation and cisplatin resistance in cutaneous squamous-cell carcinoma via KPNA4. Biochem Biophys Res Commun 2019;513:419-25. [Crossref] [PubMed]
- Yang J, Lu C, Wei J, et al. Inhibition of KPNA4 attenuates prostate cancer metastasis. Oncogene 2017;36:2868-78. [Crossref] [PubMed]
- Zhao Y, Feng X, Zhao Y, et al. Overexpression of ST7-AS1 Enhances Apoptosis and Inhibits Proliferation of Papillary Thyroid Carcinoma Cells Via microRNA-181b-5p-Dependent Inhibition Tripartite Motif Containing 3. Mol Biotechnol 2023;65:477-90. [Crossref] [PubMed]
- Huang XQ, Zhang XF, Xia JH, et al. Tripartite motif-containing 3 (TRIM3) inhibits tumor growth and metastasis of liver cancer. Chin J Cancer 2017;36:77. [Crossref] [PubMed]
- Piao MY, Cao HL, He NN, et al. Potential role of TRIM3 as a novel tumour suppressor in colorectal cancer (CRC) development. Scand J Gastroenterol 2016;51:572-82. [Crossref] [PubMed]
- Chen G, Kong J, Tucker-Burden C, et al. Human Brat ortholog TRIM3 is a tumor suppressor that regulates asymmetric cell division in glioblastoma. Cancer Res 2014;74:4536-48. [Crossref] [PubMed]
- Liang J, Wang D, Qiu G, et al. Long Noncoding RNA FOXP4-AS1 Predicts Unfavourable Prognosis and Regulates Proliferation and Invasion in Hepatocellular Carcinoma. Biomed Res Int 2021;2021:8850656. [Crossref] [PubMed]
- Ye J, Fu Y, Wang Z, et al. Long non-coding RNA FOXP4-AS1 facilitates the biological functions of hepatocellular carcinoma cells via downregulating ZC3H12D by mediating H3K27me3 through recruitment of EZH2. Cell Biol Toxicol 2022;38:1047-62. [Crossref] [PubMed]
- Gnani D, Romito I, Artuso S, et al. Focal adhesion kinase depletion reduces human hepatocellular carcinoma growth by repressing enhancer of zeste homolog 2. Cell Death Differ 2017;24:889-902. [Crossref] [PubMed]
- Tang Y, Yang P, Zhu Y, et al. LncRNA TUG1 contributes to ESCC progression via regulating miR-148a-3p/MCL-1/Wnt/β-catenin axis in vitro. Thorac Cancer 2020;11:82-94. [Crossref] [PubMed]
- Niu Y, Wang G, Li Y, et al. LncRNA FOXP4-AS1 Promotes the Progression of Esophageal Squamous Cell Carcinoma by Interacting With MLL2/H3K4me3 to Upregulate FOXP4. Front Oncol 2021;11:773864. [Crossref] [PubMed]
- Li Y, Li T, Yang Y, et al. YY1-induced upregulation of FOXP4-AS1 and FOXP4 promote the proliferation of esophageal squamous cell carcinoma cells. Cell Biol Int 2020;44:1447-57. [Crossref] [PubMed]
- Chen T, Liu Y, Chen J, et al. Exosomal miR-3180-3p inhibits proliferation and metastasis of non-small cell lung cancer by downregulating FOXP4. Thorac Cancer 2021;12:372-81. [Crossref] [PubMed]
- Ma HF, He WW, Wang JJ. Long noncoding RNA LINC00858 promotes the proliferation, migration and invasion of gastric cancer cells via the miR-363-3p/FOXP4 axis. Eur Rev Med Pharmacol Sci 2020;24:9391-9. [Crossref] [PubMed]
- Wu X, Xiao Y, Zhou Y, et al. LncRNA FOXP4-AS1 is activated by PAX5 and promotes the growth of prostate cancer by sequestering miR-3184-5p to upregulate FOXP4. Cell Death Dis 2019;10:472. [Crossref] [PubMed]
- Li D, Li Z. LncRNA FOXP4-AS promotes the progression of non-small cell lung cancer by regulating the miR-3184-5p/EIF5A axis. J Tissue Eng Regen Med 2022;16:335-45. [Crossref] [PubMed]
- Sfakianos AP, Raven RM, Willis AE. The pleiotropic roles of eIF5A in cellular life and its therapeutic potential in cancer. Biochem Soc Trans 2022;50:1885-95. [Crossref] [PubMed]
- Yao L, Wang T, Wang X. LncRNA FOXP4-AS1 serves as a biomarker for nasopharyngeal carcinoma diagnosis and prognosis. 3 Biotech 2021;11:25.
- Zhong LK, Zhou J, He X, et al. Long non-coding RNA FOXP4-AS1 acts as an adverse prognostic factor and regulates proliferation and apoptosis in nasopharyngeal carcinoma. Eur Rev Med Pharmacol Sci 2020;24:8008-16. [Crossref] [PubMed]
- Yan J, Zhou Q. LncRNA FOXP4-AS1 silencing inhibits metastasis and epithelial-mesenchymal transition in nasopharyngeal carcinoma via miR-136-5p/MAPK1. Anticancer Drugs 2023;34:1104-11. [Crossref] [PubMed]
- Zhao J, Yang T, Li L. LncRNA FOXP4-AS1 Is Involved in Cervical Cancer Progression via Regulating miR-136-5p/CBX4 Axis. Onco Targets Ther 2020;13:2347-55. [Crossref] [PubMed]
- Xiong J, Wu L, Huang L, et al. LncRNA FOXP4-AS1 Promotes Progression of Ewing Sarcoma and Is Associated With Immune Infiltrates. Front Oncol 2021;11:718876. [Crossref] [PubMed]
- Ju T, Jin H, Ying R, et al. Overexpression of NAC1 confers drug resistance via HOXA9 in colorectal carcinoma cells. Mol Med Rep 2017;16:3194-200. [Crossref] [PubMed]
- Tao HF, Shen JX, Hou ZW, et al. lncRNA FOXP4 AS1 predicts poor prognosis and accelerates the progression of mantle cell lymphoma through the miR 423 5p/NACC1 pathway. Oncol Rep 2021;45:469-80. [Crossref] [PubMed]
- Cheng Z, Jiang S, Tao R, et al. Activating transcription factor 3-activated long noncoding RNA forkhead box P4-antisense RNA 1 aggravates colorectal cancer progression by regulating microRNA-423-5p/nucleus accumbens associated 1 axis. Bioengineered 2022;13:2114-29. [Crossref] [PubMed]
- Yu T, Bachman J, Lai ZC. Mutation analysis of large tumor suppressor genes LATS1 and LATS2 supports a tumor suppressor role in human cancer. Protein Cell 2015;6:6-11. [Crossref] [PubMed]
- Yang L, Ge D, Chen X, et al. FOXP4-AS1 participates in the development and progression of osteosarcoma by downregulating LATS1 via binding to LSD1 and EZH2. Biochem Biophys Res Commun 2018;502:493-500. [Crossref] [PubMed]
- Luo X, Gao Q, Zhou T, et al. FOXP4-AS1 Inhibits Papillary Thyroid Carcinoma Proliferation and Migration Through the AKT Signaling Pathway. Front Oncol 2022;12:900836. [Crossref] [PubMed]
- Li Y, Cao X, Li H. Identification and Validation of Novel Long Non-coding RNA Biomarkers for Early Diagnosis of Oral Squamous Cell Carcinoma. Front Bioeng Biotechnol 2020;8:256. [Crossref] [PubMed]
- He L, Yang H, Zhu XL, et al. Knockdown of long non-coding RNA SLC8A1-AS1 attenuates cell invasion and migration in glioma via suppression of Wnt/β-catenin signaling pathways. Brain Res Bull 2021;176:112-20. [Crossref] [PubMed]
- Xin Y, Shang X, Sun X, et al. SLC8A1 antisense RNA 1 suppresses papillary thyroid cancer malignant progression via the FUS RNA binding protein (FUS)/NUMB like endocytic adaptor protein (Numbl) axis. Bioengineered 2022;13:12572-82. [Crossref] [PubMed]
- Huang C, Su X, Zhou DL, et al. A diagnostic and predictive lncRNA lnc-MPEG1-1 promotes the proliferation and metastasis of papillary thyroid cancer cells by occupying miR-766-5p. Mol Ther Nucleic Acids 2022;28:408-22. [Crossref] [PubMed]
- Bai Y, Zhang G, Cheng R, et al. CASC15 contributes to proliferation and invasion through regulating miR-766-5p/ KLK12 axis in lung cancer. Cell Cycle 2019;18:2323-31. [Crossref] [PubMed]
- Cai Y, Zhang K, Cao L, et al. Inhibition of Microrna-766-5p Attenuates the Development of Cervical Cancer Through Regulating SCAI. Technol Cancer Res Treat 2020;19:1533033820980081. [Crossref] [PubMed]
- Wang S, Xie J, Li J, et al. Cisplatin suppresses the growth and proliferation of breast and cervical cancer cell lines by inhibiting integrin β5-mediated glycolysis. Am J Cancer Res 2016;6:1108-17.
- Shi W, He J, Huang Y, et al. Integrin β5 enhances the malignancy of human colorectal cancer by increasing the TGF-β signaling. Anticancer Drugs 2021;32:717-26. [Crossref] [PubMed]
- Huang C, Li K, Huang R, et al. RNF185-AS1 promotes hepatocellular carcinoma progression through targeting miR-221-5p/integrin β5 axis. Life Sci 2021;267:118928. [Crossref] [PubMed]
- Liu D, Zhang M, Song Y, et al. RNF185 antisense RNA 1 (RNF185-AS1) promotes proliferation, migration, and invasion in papillary thyroid carcinoma. Anticancer Drugs 2022;33:595-606. [Crossref] [PubMed]
- Zou X, Sun P, Xie H, et al. Knockdown of long noncoding RNA HUMT inhibits the proliferation and metastasis by regulating miR-455-5p/LRP4 axis in hepatocellular carcinoma. Bioengineered 2022;13:8051-63. [Crossref] [PubMed]
- Mao Z, Wang Z, Zhang S, et al. LRP4 promotes migration and invasion of gastric cancer under the regulation of microRNA-140-5p. Cancer Biomark 2020;29:245-53. [Crossref] [PubMed]
- Fu Z, Li G, Li Z, et al. Endogenous miRNA Sponge LincRNA-ROR promotes proliferation, invasion and stem cell-like phenotype of pancreatic cancer cells. Cell Death Discov 2017;3:17004. [Crossref] [PubMed]
- Chen Y, Shen Z, Zhi Y, et al. Long non-coding RNA ROR promotes radioresistance in hepatocelluar carcinoma cells by acting as a ceRNA for microRNA-145 to regulate RAD18 expression. Arch Biochem Biophys 2018;645:117-25. [Crossref] [PubMed]
- Li J, Zhang S, Wu L, et al. Interaction between LncRNA-ROR and miR-145 contributes to epithelial-mesenchymal transition of ovarian cancer cells. Gen Physiol Biophys 2019;38:461-71. [Crossref] [PubMed]
- Su X, Feng X, Gao C, et al. ROR promotes the proliferation and migration of esophageal cancer through regulating miR-145/LMNB2 signal axis. Am J Transl Res 2020;12:7223-35.
- Liu T, Chi H, Chen J, et al. Curcumin suppresses proliferation and in vitro invasion of human prostate cancer stem cells by ceRNA effect of miR-145 and lncRNA-ROR. Gene 2017;631:29-38. [Crossref] [PubMed]
- Jiang B, Zhu H, Tang L, et al. Apatinib Inhibits Stem Properties and Malignant Biological Behaviors of Breast Cancer Stem Cells by Blocking Wnt/β-catenin Signal Pathway through Downregulating LncRNA ROR. Anticancer Agents Med Chem 2022;22:1723-34. [Crossref] [PubMed]
- Chen Y, Peng Y, Xu Z, et al. LncROR Promotes Bladder Cancer Cell Proliferation, Migration, and Epithelial-Mesenchymal Transition. Cell Physiol Biochem 2017;41:2399-410. [Crossref] [PubMed]
- Zhang HY, Liang F, Zhang JW, et al. Effects of long noncoding RNA-ROR on tamoxifen resistance of breast cancer cells by regulating microRNA-205. Cancer Chemother Pharmacol 2017;79:327-37. [Crossref] [PubMed]
- Wang SH, Zhang MD, Wu XC, et al. Overexpression of LncRNA-ROR predicts a poor outcome in gallbladder cancer patients and promotes the tumor cells proliferation, migration, and invasion. Tumour Biol 2016;37:12867-75. [Crossref] [PubMed]
- Li L, Gu M, You B, et al. Long non-coding RNA ROR promotes proliferation, migration and chemoresistance of nasopharyngeal carcinoma. Cancer Sci 2016;107:1215-22. [Crossref] [PubMed]
- Li H, Jiang X, Niu X. Long Non-Coding RNA Reprogramming (ROR) Promotes Cell Proliferation in Colorectal Cancer via Affecting P53. Med Sci Monit 2017;23:919-28. [Crossref] [PubMed]
- Shi J, Zhang W, Tian H, et al. lncRNA ROR promotes the proliferation of renal cancer and is negatively associated with favorable prognosis. Mol Med Rep 2017;16:9561-6. [Crossref] [PubMed]
- Hu A, Hong F, Li D, et al. Long non-coding RNA ROR recruits histone transmethylase MLL1 to up-regulate TIMP3 expression and promote breast cancer progression. J Transl Med 2021;19:95. [Crossref] [PubMed]
- Zeng SY, Liu CQ, Zhuang Y, et al. LncRNA ROR promotes proliferation of endometrial cancer cells via regulating Notch1 pathway. Eur Rev Med Pharmacol Sci 2020;24:5970-8. [Crossref] [PubMed]
- Li X, Chen W, Jia J, et al. The Long Non-Coding RNA-RoR Promotes the Tumorigenesis of Human Colorectal Cancer by Targeting miR-6833-3p Through SMC4. Onco Targets Ther 2020;13:2573-81. [Crossref] [PubMed]
- Ma YL, Wang CY, Guan YJ, et al. Long noncoding RNA ROR promotes proliferation and invasion of colorectal cancer by inhibiting tumor suppressor gene NF2 through interacting with miR-223-3p. Eur Rev Med Pharmacol Sci 2020;24:2401-11. [Crossref] [PubMed]
- Hou L, Tu J, Cheng F, et al. Long noncoding RNA ROR promotes breast cancer by regulating the TGF-β pathway. Cancer Cell Int 2018;18:142. [Crossref] [PubMed]
- Fan J, Xing Y, Wen X, et al. Long non-coding RNA ROR decoys gene-specific histone methylation to promote tumorigenesis. Genome Biol 2015;16:139. [Crossref] [PubMed]
- Jin W, Zhang H, Li M, et al. Long Noncoding RNA Regulator of Reprogramming Regulates Cell Growth, Metastasis, and Cisplatin Resistance in Gastric Cancer via miR-519d-3p/HMGA2 Axis. Cancer Biother Radiopharm 2023;38:122-31. [Crossref] [PubMed]
- Wang S, Chen W, Yu H, et al. lncRNA ROR Promotes Gastric Cancer Drug Resistance. Cancer Control 2020;27:1073274820904694. [Crossref] [PubMed]
- Wang S, Liu F, Deng J, et al. Long Noncoding RNA ROR Regulates Proliferation, Invasion, and Stemness of Gastric Cancer Stem Cell. Cell Reprogram 2016;18:319-26. [Crossref] [PubMed]
- Cheng FH, Zhao ZS, Liu WD. Long non-coding RNA ROR regulated ABCB1 to induce cisplatin resistance in osteosarcoma by sponging miR-153-3p. Eur Rev Med Pharmacol Sci 2019;23:7256-65. [Crossref] [PubMed]
- Fan Y, Fan X, Yan H, et al. Long non-coding ROR promotes the progression of papillary thyroid carcinoma through regulation of the TESC/ALDH1A1/TUBB3/PTEN axis. Cell Death Dis 2022;13:157. [Crossref] [PubMed]
- Yang T, Tian S, Zhao J, et al. LncRNA ABHD11-AS1 activates EGFR signaling to promote cervical cancer progression by preventing FUS-mediated degradation of ABHD11 mRNA. Cell Cycle 2023;22:2538-51. [Crossref] [PubMed]
- Hou S, Zhang X, Yang J. Long non-coding RNA ABHD11-AS1 facilitates the progression of cervical cancer by competitively binding to miR-330-5p and upregulating MARK2. Exp Cell Res 2022;410:112929. [Crossref] [PubMed]
- Zhu D, Hao Q, Qian M, et al. LncRNA ABHD11-AS1 Participates in the Progression of Cervical Carcinoma by Targeting miR-1254 and Is the Key to the Diagnosis and Treatment of Cervical Carcinoma in the Future. J Healthc Eng 2022;2022:8387458. [Crossref] [PubMed]
- Zhang W, Huang X, Shi J. EZH2-mediated lncRNA ABHD11-AS1 promoter regulates the progression of ovarian cancer by targeting miR-133a-3p. Anticancer Drugs 2021;32:269-77. [Crossref] [PubMed]
- Zeng XY, Jiang XY, Yong JH, et al. lncRNA ABHD11-AS1, regulated by the EGFR pathway, contributes to the ovarian cancer tumorigenesis by epigenetically suppressing TIMP2. Cancer Med 2019;8:7074-85. Retracted Publication. [Crossref] [PubMed]
- Wu DD, Chen X, Sun KX, et al. Role of the lncRNA ABHD11-AS(1) in the tumorigenesis and progression of epithelial ovarian cancer through targeted regulation of RhoC. Mol Cancer 2017;16:138. [Crossref] [PubMed]
- Liu Y, Wang LL, Chen S, et al. LncRNA ABHD11-AS1 promotes the development of endometrial carcinoma by targeting cyclin D1. J Cell Mol Med 2018;22:3955-64. [Crossref] [PubMed]
- Dong Y, Zhang T, Shao S, et al. Knockdown of ABHD11 AS1 prevents the procession of TNBC by upregulating miR 199a 5p. Biomed Rep 2023;19:69. [Crossref] [PubMed]
- Xin H, Yan Z, Cao J. Long non-coding RNA ABHD11-AS1 boosts gastric cancer development by regulating miR-361-3p/PDPK1 signalling. J Biochem 2020;168:465-76. [Crossref] [PubMed]
- Yang Y, Shao Y, Zhu M, et al. Using gastric juice lncRNA-ABHD11-AS1 as a novel type of biomarker in the screening of gastric cancer. Tumour Biol 2016;37:1183-8. [Crossref] [PubMed]
- Liu B, Wang W, Sun S, et al. Knockdown of lncRNA ABHD11-AS1 Suppresses the Tumorigenesis of Pancreatic Cancer via Sponging miR-1231. Onco Targets Ther 2020;13:11347-58. [Crossref] [PubMed]
- Qiao X, Lv SX, Qiao Y, et al. Long noncoding RNA ABHD11-AS1 predicts the prognosis of pancreatic cancer patients and serves as a promoter by activating the PI3K-AKT pathway. Eur Rev Med Pharmacol Sci 2018;22:8630-9. [Crossref] [PubMed]
- Luo J, Jiang Y, Wu L, et al. Long non-coding RNA ABHD11-AS1 promotes colorectal cancer progression and invasion through targeting the integrin subunit alpha 5/focal adhesion kinase/phosphoinositide 3 kinase/Akt signaling pathway. Aging (Albany NY) 2021;13:20179-91. [Crossref] [PubMed]
- Lei X, Li L, Duan X. Long non-coding RNA ABHD11-AS1 promotes colorectal cancer development through regulation of miR-133a/SOX4 axis. Biosci Rep 2018;38:BSR20181386. [Crossref] [PubMed]
- He D, Yue Z, Liu L, et al. Long noncoding RNA ABHD11-AS1 promote cells proliferation and invasion of colorectal cancer via regulating the miR-1254-WNT11 pathway. J Cell Physiol 2019;234:12070-9. [Crossref] [PubMed]
- Lu H, Zhu C, Chen Y, et al. LncRNA ABHD11-AS1 promotes tumor progression in papillary thyroid carcinoma by regulating EPS15L1/EGFR signaling pathway. Clin Transl Oncol 2022;24:1124-33. [Crossref] [PubMed]
- Zhuang X, Tong H, Ding Y, et al. Long noncoding RNA ABHD11-AS1 functions as a competing endogenous RNA to regulate papillary thyroid cancer progression by miR-199a-5p/SLC1A5 axis. Cell Death Dis 2019;10:620. [Crossref] [PubMed]
- Wen J, Wang H, Dong T, et al. STAT3-induced upregulation of lncRNA ABHD11-AS1 promotes tumour progression in papillary thyroid carcinoma by regulating miR-1301-3p/STAT3 axis and PI3K/AKT signalling pathway. Cell Prolif 2019;52:e12569. [Crossref] [PubMed]
- Guo K, Qian K, Shi Y, et al. LncRNA-MIAT promotes thyroid cancer progression and function as ceRNA to target EZH2 by sponging miR-150-5p. Cell Death Dis 2021;12:1097. [Crossref] [PubMed]
- Su Y, Mei L, Jiang T, et al. Novel role of lncRNAs regulatory network in papillary thyroid cancer. Biochem Biophys Rep 2024;38:101674. [Crossref] [PubMed]
- Mahmoudian-Sani MR, Jalali A, Jamshidi M, et al. Long Non-Coding RNAs in Thyroid Cancer: Implications for Pathogenesis, Diagnosis, and Therapy. Oncol Res Treat 2019;42:136-42. [Crossref] [PubMed]
- Gallant-Behm CL, Piper J, Lynch JM, et al. A MicroRNA-29 Mimic (Remlarsen) Represses Extracellular Matrix Expression and Fibroplasia in the Skin. J Invest Dermatol 2019;139:1073-81. [Crossref] [PubMed]
- Hassan M, Elzallat M, Aboushousha T, et al. MicroRNA-122 mimic/microRNA-221 inhibitor combination as a novel therapeutic tool against hepatocellular carcinoma. Noncoding RNA Res 2023;8:126-34. [Crossref] [PubMed]
- Bennett CF. Therapeutic Antisense Oligonucleotides Are Coming of Age. Annu Rev Med 2019;70:307-21. [Crossref] [PubMed]
- Bennett CF, Swayze EE. RNA targeting therapeutics: molecular mechanisms of antisense oligonucleotides as a therapeutic platform. Annu Rev Pharmacol Toxicol 2010;50:259-93. [Crossref] [PubMed]
- Friedrich M, Aigner A. Therapeutic siRNA: State-of-the-Art and Future Perspectives. BioDrugs 2022;36:549-71. [Crossref] [PubMed]
- Zhang MM, Bahal R, Rasmussen TP, et al. The growth of siRNA-based therapeutics: Updated clinical studies. Biochem Pharmacol 2021;189:114432. [Crossref] [PubMed]
- Haugen BR, Alexander EK, Bible KC, et al. 2015 American Thyroid Association Management Guidelines for Adult Patients with Thyroid Nodules and Differentiated Thyroid Cancer: The American Thyroid Association Guidelines Task Force on Thyroid Nodules and Differentiated Thyroid Cancer. Thyroid 2016;26:1-133. [Crossref] [PubMed]
- Liu Y, Wang J, Hu X, et al. Radioiodine therapy in advanced differentiated thyroid cancer: Resistance and overcoming strategy. Drug Resist Updat 2023;68:100939. [Crossref] [PubMed]
- Xiang C, Zhang ML, Zhao QZ, et al. LncRNA-SLC6A9-5:2: A potent sensitizer in 131I-resistant papillary thyroid carcinoma with PARP-1 induction. Oncotarget 2017;8:22954-67. [Crossref] [PubMed]
- Tao L, Tian P, Yang L, et al. lncRNA CASC2 Enhances (131)I Sensitivity in Papillary Thyroid Cancer by Sponging miR-155. Biomed Res Int 2020;2020:7183629. [Crossref] [PubMed]
- Winkle M, El-Daly SM, Fabbri M, et al. Noncoding RNA therapeutics - challenges and potential solutions. Nat Rev Drug Discov 2021;20:629-51. [Crossref] [PubMed]
- Nemeth K, Bayraktar R, Ferracin M, et al. Non-coding RNAs in disease: from mechanisms to therapeutics. Nat Rev Genet 2024;25:211-32. [Crossref] [PubMed]